The term polysomnography (PSG) describes the overnight monitoring during sleep of multiple biologic variables and the evaluation of their relationships within a specific state of alertness. It uses the 10–20 international electrode placement system for electroencephalography (EEG) in addition to monitoring respiratory parameters (airflow, snoring, effort, oxygen saturation, with or without carbon dioxide [CO 2 ] monitoring), cardiac monitoring, electromyographic recording, and video recording. Clinical PSG allows a broad investigation of biologic functions during different states of alertness. PSG can facilitate the investigation of the impact of sleep stages on the control of vital organs and on the autonomic nervous system. It can illustrate the impact of circadian rhythms on diverse biologic functions. Additionally, PSG can help evaluate the efficacy of treatment for various sleep disorders.
History of polysomnography
Hans Berger recorded the first continuous overnight EEG tracing in 1929 and demonstrated patterns that correlated with sleep and wakefulness. Loomis and colleagues devised a classification system for EEG during sleep in the late 1930s. In their classic paper in 1953, Aserinsky and Kleitman reported the cyclic occurrence during sleep of rapid eye movements associated with a specific EEG pattern of low-voltage, fast EEG activity. Dement and Kleitman in 1957 reported on the cyclic nature of two different states of alertness, using the terms rapid eye movement (REM) and non–rapid eye movement (non-REM). The term REM sleep still has a number of synonymous names. Among the most common are desynchronized sleep; D (for desynchronized and dreaming) states, commonly used in animal research; active sleep, used mainly during the neonatal period; and paradoxical sleep.
Non-REM sleep was defined better following the initial efforts of Loomis and his team. An international conference of sleep researchers in 1968 led to the publication of the Rechtschaffen and Kales manual, which, until recently, was the most commonly utilized standardized scoring of PSG. This manual gives specific instructions on how to score sleep states and stages, and defines four sleep stages of non-REM sleep (also called synchronized sleep; slow-wave sleep; and, in infants, quiet sleep). These criteria utilize scoring by epochs (i.e., 30-second recording) and the “majority of epoch” rule. This rule applies when more than one stage occurs in an epoch, so that the stage that constitutes greater than 50 percent of the epoch is the stage with which the epoch is scored. These definitions are valid for children over 2 years of age and for young or middle-aged adults, but are not as accurate for the elderly.
A second consensus conference produced a second manual for scoring sleep in premature and newborn infants that generally was used until the 50th to 52nd postgestational week of age (i.e., until an infant born at term is 10 to 12 weeks old). This atlas, edited by Anders and colleagues, recognizes the postnatal maturation processes that occur in the brain and the difficulties of subdividing quiet (non-REM) sleep into stages. Only four states are defined: wakefulness and quiet, active, and indeterminate sleep. The last of these indicates that, even in full-term newborns, EEG and other criteria may be insufficient to separate quiet from active sleep.
In 2007, the American Academy of Sleep Medicine (AASM) introduced the AASM Manual for the Scoring of Sleep and Associated Events, which has replaced the Rechtschaffen and Kales scoring system. The major changes set forth by AASM included primarily changes in EEG derivations, reduction in number of sleep stages (Rechtschaffen and Kales: wake, stages 1,2,3,4 non-REM sleep and stage REM sleep) by merging stages 3 and 4 and the elimination of movement time (AASM: N1, N2, N3, and stage R), the simplification of many context rules, and recommendations on sampling rates and filter settings for polysomnographic reporting and user interfaces for computer-assisted analysis.
Sleep overview
Human existence represents a continuum of three states of being: (1) wakefulness; (2) non-REM sleep; and (3) REM sleep. Wakefulness is associated with an alert state; eye movements with eyes open; erect, sitting, or recumbent posture; normal responsiveness to stimuli; and normal mobility. Sleep, by contrast, is associated with reversible unconsciousness, a closed-eye position with or without eye movements, recumbent posture, mild to moderate reduction in responsiveness to stimuli, and reduced or absent mobility.
Sleep Onset and Behavioral Correlates
Sleep onset is associated with perceptual disengagement from visual and auditory stimuli, discriminant response to meaningful versus nonmeaningful stimuli, impairment of memory, occurrence of hypnic myoclonia, and “automatic behavior.” Guilleminault and colleagues presented light flashes and instructed EEG-monitored subjects to press a microswitch taped to their hands. When the EEG patterns were consistent with stage 1 or stage 2 sleep, responses were absent 85 percent of the time, and subjects later reported that they did not see the light flash. Others reported that subjects exhibited longer reaction times in response to auditory tones presented close to the onset of stage 1 sleep, and responses were absent during unequivocal sleep. Soft calling of one’s name during light sleep may evoke an arousal, whereas a nonmeaningful stimulus may not. It has been hypothesized that sleep may close the gate between short-term memory and long-term memory stores. An experiment in which word pairs were presented at 1-minute intervals to volunteers demonstrated that when subjects were awakened 30 seconds after sleep onset, they could recall words presented 4 to 10 minutes (defined as long-term memory) and 0 to 3 minutes (defined as short-term memory) before EEG-defined sleep onset. By contrast, if they were awakened 10 minutes after sleep onset, they could recall words presented at 4 to 10 minutes but not words presented at 0 to 3 minutes before sleep onset. This experiment suggested that sleep inactivates the transfer of storage from short-term to long-term memory or that the encoding of the material presented before sleep onset was not of sufficient strength to allow recall. General or local muscle contraction (hypnic myoclonus), often associated with visual imagery, may be noted at sleep onset. “Automatic behavior” (e.g., subjects continuing to tap a switch for a few seconds after sleep onset) has also been reported.
EEG at sleep onset demonstrates a change from rhythmic alpha (8- to 13-Hz) activity to a relatively low-voltage mixed-frequency pattern. This change usually occurs seconds to minutes after the electro-oculogram (EOG) shows slow, often asynchronous, eye movements. The electromyogram (EMG) demonstrates gradual diminution in level.
Temporal Organization of Normal Sleep
Nocturnal sleep is associated with a regular pattern. A normal adult first enters non-REM sleep and then goes into REM sleep after about 80 or 90 minutes. Thereafter, non-REM and REM sleep alternate throughout the night with a periodicity of about 100 minutes. The combination of one non-REM sleep segment and one REM sleep segment is a sleep cycle.
Sleep Stages
Under the criteria of Rechtschaffen and Kales, non-REM sleep consists of four stages (1 to 4). Stage 1 sleep is associated with slow eye movements; EMG levels below those of relaxed wakefulness; and low-voltage, mixed-frequency EEG in the 2- to 7-Hz frequencies ( Fig. 33-1 ). During the latter part of stage 1, vertex sharp waves may appear associated with high-amplitude 2- to 7-Hz activity. Scoring of stage 1 sleep requires an absolute absence of clearly defined K-complexes and sleep spindles. Stage 2 sleep is defined by the presence of K-complexes or sleep spindles and by the presence of sleep-delta activity for less than 20 percent of the epoch ( Fig. 33-2 ). K-complexes are seen maximally over the vertex regions and represent EEG waveforms consisting of a well-defined negative sharp wave immediately followed by a positive component, with the entire complex exceeding 0.5 seconds in duration. Waves of 12- to 14-Hz activity may or may not be part of the complex. Stages 3 and 4 comprise slow-wave sleep. Stage 3 is scored when at least 20 percent but not more than 50 percent of the epoch consists of slow delta waves (i.e., 2 Hz or less, with amplitude greater than 75 μV measured from peak to peak) ( Fig. 33-3 ). Stage 4 is scored when at least 50 percent of the epoch consists of such delta waves. Sleep spindles may or may not be present in stage 3 or 4.



The 2007 AASM scoring manual designates three stages of non-REM sleep (N1, N2, N3) and one stage of REM sleep (R). N1 is equivalent to stage 1 non-REM sleep, N2 to stage 2 non-REM sleep, N3 to stages 3 and 4 non-REM sleep, and R to stage REM sleep.
EEG during REM sleep demonstrates the concomitant appearance of low-voltage, mixed-frequency EEG activity and episodic rapid eye movements. During REM sleep, “sawtooth” (i.e., 2- to 3-Hz, sharply contoured, triangular) waves can be noted in the vertex and frontal regions in conjunction with bursts of REM ( Fig. 33-4 ). Alpha activity can also be seen, but its frequency is 1 to 2 Hz slower than during wakefulness. There is an absolute absence of sleep spindles and K-complexes during REM sleep. REM sleep can be divided into tonic REM sleep and phasic REM sleep. During tonic REM sleep, the EEG is desynchronized; there is hypotonia or atonia of major muscle groups and depression of monosynaptic and polysynaptic reflexes. Tonic EMG activity is low in amplitude. Phasic REM sleep is associated with rapid eye movements; irregularities in blood pressure, heart rate, and respiration; spontaneous muscle activity; and tongue movements.

First Sleep Cycle
In a normal young adult, the first sleep cycle begins with stage 1 sleep, which generally lasts for only a few (1 to 7) minutes. Stage 1 non-REM sleep is interrupted easily, and oscillations from wakefulness to stage 1 are often observed during the sleep-onset period. Stage 2 non-REM sleep follows this short stage 1 transition from wakefulness and continues for 10 to 25 minutes. Progressive, gradual appearance of high-voltage slow-wave activity signals the evolution toward stage 3 non-REM sleep. This stage usually lasts only a few minutes and represents a transition to stage 4 non-REM sleep as more and more high-voltage activity is seen. Stage 4 non-REM sleep lasts for 20 to 40 minutes during the first cycle. If body movements occur, there is a transient switch to lighter sleep (i.e., stage 1 or stage 2).
Often, a brief switch from stage 4 to stage 2 non-REM sleep occurs several minutes before the subject goes into REM sleep. The passage from non-REM to REM sleep is not abrupt; the EMG may decline long before the switch to REM sleep occurs. Although the EEG pattern characteristic of REM sleep will become more and more prominent, REM sleep cannot be declared before identification of the first rapid eye movement. One must go back from this first eye movement to pinpoint the onset of the first REM period using EEG and EMG criteria. This first REM sleep period is short, lasting between 2 and 6 minutes. It may be suppressed easily if any discomfort occurs (which may be related to the recording apparatus). REM sleep often ends with a short body movement, and a new cycle begins.
Later Sleep Cycles
Non-REM and REM sleep continue to alternate throughout the night in a cyclic fashion. As the night progresses, each sleep cycle differs slightly from the previous one. The average length of the second and later sleep cycles is about 100 to 120 minutes. The last sleep cycle is usually the longest.
Distribution of Sleep Stages Throughout the Night
The distribution of sleep stages varies slightly during the night from sleep cycle to sleep cycle, with stage 3 and stage 4 non-REM sleep occurring mainly during the first two sleep cycles and the REM sleep period growing progressively longer in the last two sleep cycles. Stage 3 and stage 4 non-REM sleep, also known as slow-wave sleep or N3 sleep, is most prominent during the first third of the night. The preferential distribution of slow-wave sleep toward the beginning of nocturnal sleep is related to the length of prior wakefulness, the time at which sleep onset occurs, and the time course of sleep per se. Factor “S” (for slow-wave activity) may be manipulated by conditions such as sleep deprivation, sleep satiety, and time of sleep; it is the object of theoretical investigations and mathematic modeling. The preferential distribution of REM sleep toward the latter portion of the night in young adults is hypothesized to be linked to a circadian oscillator, which can be investigated by monitoring the 24-hour core body temperature. In an adult, non-REM sleep takes up approximately 80 percent of the total sleep time, with stage 1 occupying 5 percent; stage 2, 50 to 55 percent; stages 3 and 4, 20 percent; and REM sleep, 20 to 22 percent.
Effect of Age
Sleep patterns vary with age ( Fig. 33-5 ). In the neonate, active (REM) sleep usually consumes 50 percent of the total sleep time. Before puberty, this percentage rapidly declines and then stabilizes at around 20 percent until old age. Stages 3 and 4 of non-REM sleep are usually of maximal duration in childhood and decline progressively in adulthood, with fairly low levels attained around 50 to 55 years of age. Although several studies of sleep changes during aging have been performed, normative data are still relatively few, and a number of areas must still be explored.

Development of Sleep in Infancy
It has been established clearly that the basic rest–activity cycle originates during fetal life. REM sleep has been recognized in infants near 28 weeks gestational age (GA), but non-REM sleep is very difficult to identify before 34 to 35 weeks GA. It is only near the 36th week GA that observable variables such as body movement, eye movements, and changes in heart rate and respiratory patterns allow the scoring of both sleep states without much difficulty. Between the 40th week GA and the 3rd month of postnatal life in a full-term infant, a tighter association between physiologic and behavioral variables allows even easier definition of sleep and its states. During that period, the polyphasic sleeping–waking pattern, which seems to be associated with the 4-hour feeding cycle, changes to a diurnal pattern. Thus, a full-term infant has, at birth, a rhythm that is generally longer than 24 hours. A full-term newborn spends 70 to 80 percent of life asleep.
At birth, the lack of concordance between EEG, eye movements, and chin EMG results in the classifications of quiet, active, and indeterminate sleep. During active (REM) sleep, fast eye movements can be seen, often behind closed eyelids, but at times are associated with brief eye openings. Smiles, facial grimaces, frowns, blinking, and bursts of sucking are observed. Gross body writhing admixed with limb twitching is noted. Vocalizations consisting of brief grunts, whimpers, or cries are heard. The heart rate and respiratory rate are characterized by abrupt, short-lived irregularities. One breath may be missing, prolonged, or shortened. The EMG is variable but often low. The EEG during active sleep demonstrates any of the following patterns: a low-voltage (14- to 35-μV) irregular pattern admixed with slower activity (1 to 5 Hz), a mixed pattern with high- and low-voltage polyrhythmic waves intermingled without periodicity, or a high-voltage slow (HVS) pattern with continuous rhythmic medium to high voltage (50- to 150- μV) slow (0.5- to 4-Hz) waves.
During quiet sleep, the newborn has eyes closed and no fast eye movements are noted. There are no body movements except for occasional startles and mouth movements. Respiration is quiet and very regular, as is the heart rate. EMG activity is variable, but usually tonus is maintained. The EEG demonstrates one of the following patterns: tracé alternant pattern of bursts of high-voltage slow waves (0.5 to 3 Hz) with superimposed rapid low-voltage waves and with sharp waves of 2 to 4 Hz interspersed between the slow waves; a low-voltage irregular pattern; or a mixed pattern.
It is usual to require that, during a given “epoch” (usually 30 to 60 seconds of a recording), three variables (i.e., EEG, EMG, and EOG) be concordant for the state in order for it to be scored. If concordance is lacking, indeterminate sleep is the recommended label. Identification of various non-REM and REM features of sleep becomes easier with increasing age of the subject. By 3 months of age, non-REM and REM sleep can be recognized. At this age, non-REM sleep can be subdivided into two stages: 1 and 2; and 3 and 4 (or delta) sleep. Theta activity (4- to 7-Hz) is prominent during stage 1 or 2, but sleep spindles can be recognized by their synchronous or asynchronous occurrence over both hemispheres. When 20 percent or more of an epoch contains delta waves, stage 3 or stage 4 (delta) sleep is scored. By 3 months of age, stage 3 or stage 4 sleep has become prominent at the beginning of the night; the prominence of REM sleep during the second half of the night is noted clearly after 6 months of age. The classic stages 1 to 4 of non-REM sleep can be dissociated completely from one another by 6 months of age.
Sleep states can be recognized more easily with increasing age. Total sleep time takes up about 70 percent of the 24-hour period at birth. Between the ages of 6 and 10 weeks, a circadian rhythm emerges, the timing of which suggests interaction between environmental and maturational influences. At about 3 months of age, a rhythm has been established, with sleep occurring predominantly in the nocturnal period. Once this longest sleep period has been established, the longest period of wakefulness is established in the late afternoon or early evening, just before the nocturnal period. With maturation, wakefulness progressively increases, and by 6 months of age the infant remains awake for about 50 percent of the 24-hour period. The increase in wakefulness at this age occurs mainly through a decrease in the proportion of REM sleep from one-third to one-quarter of the sleep time. As children age, the proportion of time spent in REM sleep declines. By the age of 5 years, REM sleep is reduced further to between 20 and 22 percent of the total sleep time, whereas the time spent in stage 4 non-REM sleep increases from 14 to 18 percent between the ages of 2 and 5 years. So begins the pattern of sleep and wakefulness that will continue more or less throughout life. The distribution of sleep and wakefulness in the morning hours and early afternoon is still variable. At this age, the maturational processes have been such that sleep scoring can be based on classic EEG, EMG, and EOG criteria.
Recognition of these stages in infants is important. A lack of concordance for scoring states or a disturbance in maturation that impairs the scoring of stages at the appropriate time indicates that a pathologic process has interacted or is interacting with development of the sleep–wake cycle. During the first year of life, the nocturnal organization of sleep that will continue throughout life is established.
In summary, the basic sleep–wake organization of adults is present in infants by 3 months of age. Some changes do occur thereafter, but they are much less profound than those occurring during the first 3 months. All of the sleep stages observed in the adult can be identified and scored by 6 months of age, and it appears that the initial organization of wakefulness follows the organization of sleep.
The AASM pediatric taskforce indicated that Anders infant sleep criteria remain suitable for scoring sleep–wake states in infants of 46 to 48 weeks conceptional age, until sleep spindles are observed. With the Anders criteria, the newborn infant has four states: quiet sleep, active sleep, indeterminate sleep, and wakefulness. The 2007 AASM scoring rules for wakefulness and sleep are applicable to children older than 2 months post-term. Arousals are also defined. Caution is advised in adopting the new scoring criteria in children, since significant differences have been demonstrated between the two scoring systems: N1 (AASM) percentage is significantly higher than stage 1 (Rechtschaffen and Kales) while N2 and R percentages are significantly lower than stage 2 and REM (Rechtschaffen and Kales). Additionally, there is relatively low concordance for scoring stage shifts per hour between the two systems.
Sleep in Older Children
In prepubertal and pubertal children, slow-wave sleep (stage 3 and stage 4 non-REM sleep) undergoes further changes. The slow-wave sleep seen in the first sleep cycle in this age group appears resistant to arousal compared with that found in any other age group. For example, in one study, no sign of arousal with 123-dB tone stimuli presented during stage 3 or stage 4 non-REM sleep could be found in a group of 10-year-olds. This finding is interesting because most parasomnias during childhood (particularly sleepwalking and night terrors) occur during the first slow-wave cycle. In specific experiments, children of this age were moved into an upright position during slow-wave sleep without being awakened fully. In most cases, sleepwalking episodes were triggered. These experiments indicate that deambulation (automatic walking) may be induced in normal children. The children will go back to sleep immediately if helped back to bed and will have complete amnesia for the event the following morning (Guilleminault, unpublished data). A decrease in slow-wave sleep of nearly 40 percent during the pubertal years has been reported. It has been hypothesized that the age-related decline in slow-wave sleep may run parallel to a loss of cortical synaptic density. The AASM 2007 manual has suggested grouping stages 3 and 4 together as N3 sleep, even in children. However, objections have been raised, as reduction in stage 4 percentage may affect hormonal secretion significantly, while N3 percentage may appear normal or minimally reduced. Growth hormone secretion is much more linked to stage 4 sleep in prepubertal children.
Sleep in the Elderly
In a meta-analysis of sleep parameters by age, Ohayon and colleagues showed that, in healthy adults, total sleep time, sleep efficiency, REM latency, percentage of slow-wave sleep, and percentage of REM sleep all decline with age, whereas sleep latency, wake after sleep onset, and percentages of stages 1 and 2 non-REM sleep increase. However, after age 60 years, only sleep efficiency continues to decrease significantly. The major change in sleep in the elderly is the decreased percentage of slow-wave sleep and increased stage 1 sleep, which is more pronounced in men. The amplitude of slow-wave sleep declines with age, but in parallel to EEG waves of other frequencies. The Visual Scoring Task Force and the Geriatric Task Force of the AASM voted to retain the 75-μV criterion for all ages and recommended that the frontal derivation be utilized for scoring slow-wave sleep. N3 sleep is scored when 20 percent or more of an epoch consists of 0.5- to 2-Hz frequencies with peak-to-peak amplitude exceeding 75 μV in the frontal derivation.
Sleep and its pathology
Disturbances of sleep lead to three different complaints:
- 1.
“Tiredness,” “fatigue,” and “sleepiness” at times when the subject would like to be awake and alert
- 2.
Difficulty in falling asleep or in maintaining sleep; or complaints of unrefreshing sleep, associated with fear of a decrease in performance during wakefulness because of lack of sleep
- 3.
Complaints by spouses or roommates that their own sleep is disturbed by the abnormal behavior presented by the index case during sleep.
These three very different complaints have led to the creation of three major subcategories of pathology: excessive daytime sleepiness, insomnias, and parasomnias.
Epidemiologic studies in many Westernized countries have revealed that sleep disorders are a dominant health problem. Investigations performed in Italy, Finland, Israel, and several metropolitan areas of the United States have found that excessive daytime sleepiness affects between 3.7 and 4.2 percent of the general population. By comparison, Parkinson disease has an overall incidence of 1 percent among the population older than 50 years of age, and the prevalence of epilepsy is calculated at 0.5 percent.
The incidence of complaints of insomnia varies with age and gender, with prevalence ranging from 10 to 28 percent. Chronic hypnotic usage has varied between 10 and 16 percent in Western civilizations. In general, surveys have found that chronic and frequent usage of hypnotics increases with age, and that the most common consumer is a widowed, divorced, or separated woman older than 50 years. However, in surveys in the San Francisco Bay area and in San Diego, 58 percent of those aged 6 to 14 years and 56 percent of those aged 15 to 19 years reported a sleep problem at the time of the survey; few sought medical attention. Such data indicate that many subjects with sleep problems go unrecognized.
The epidemiology of parasomnias has not been studied adequately in the general population. Arousal disorders such as sleepwalking, confusional arousals, and sleep terrors occur primarily during childhood and rarely persist during adulthood. The prevalence of sleep terrors in childhood ranges from 1.0 to 6.5 percent, whereas the prevalence in adults is not known. Sleepwalking has a prevalence in adults ranging from 0.5 to 3 percent and is not gender related.
Diagnostic tests
Different approaches have been used to investigate sleep and its pathologies. Subjective ratings, validated scales, and questionnaires such as the Stanford Sleepiness Scale and Epworth Sleepiness Scale have often been used to determine levels of sleepiness. Sleep disorders can be evaluated utilizing polygraphic monitoring of sleep and wakefulness. Currently, the most commonly utilized tests include the following:
- 1.
Nocturnal or 24-hour polygraphic monitoring, i.e., PSG
- 2.
Portable monitoring
- 3.
The multiple sleep latency test (MSLT)
- 4.
The maintenance of wakefulness test (MWT)
- 5.
Psychomotor vigilance testing
- 6.
Videopolysomnography
- 7.
Pupillography
- 8.
Actigraphy.
Polysomnography
The term polysomnography denotes the nocturnal or 24-hour monitoring of sleep and wakefulness and their impact on biologic functions. PSG generally is performed under conditions conducive to natural sleep, with the patient monitored remotely from a separate room. The ideal setting for the patient is a private, quiet room that can be darkened as necessary. Numerous variables are recorded during sleep including EEG (sleep staging), EOG, submental EMG, nasal or oral airflow, respiratory effort, oximetry, electrocardiogram (ECG), tibialis anterior EMG, and position monitoring. Depending upon the clinical diagnosis and protocol implemented, other measurable factors such as transcutaneous carbon dioxide (CO 2 ) or end-tidal gas analysis, extremity muscle activity, motor activity/movement, extended video-EEG, penile tumescence, esophageal pressure, gastroesophageal reflux, continuous blood pressure, and snoring activity may be added. To determine the type of study required, it is important to know the reason that the PSG is being undertaken.
Indications
PSG is indicated routinely in the following circumstances :
- 1.
Diagnosis of sleep-related breathing disorders (a full-night PSG is recommended).
- 2.
Titration of positive airway pressure in patients with sleep-related breathing disorders (a full night of PSG is recommended). PSG with titration is indicated for patients with respiratory disturbance index (RDI) of greater than 15 or patients with RDI greater than or equal to 5 who are excessively sleepy. A cardiorespiratory PSG (without EEG leads) should not be utilized for titration. A split-night study (initial diagnostic followed by titration of continuous positive airway pressure, or CPAP, during PSG on the same night) is an alternative to the full-night diagnostic followed by a full night of titration under the following four criteria:
- a.
An apnea-hypopnea index of at least 40 is documented during a minimum of 2 hours of diagnostic PSG.
- b.
An apnea-hypopnea index of 20 to 40 (based on clinical judgment) is documented during a minimum of 2 hours of diagnostic PSG.
- c.
At least 3 hours of CPAP titration is carried out and PSG documents that CPAP eliminates or nearly eliminates the respiratory events during REM and non-REM sleep, including during supine REM sleep.
- d.
A second full night of PSG for CPAP titration is performed if the diagnosis of sleep-related breathing is confirmed but criteria (b) and (c) are not met.
- a.
- 3.
Preoperative evaluation for obstructive sleep apnea (OSA) in all patients prior to undergoing surgery of the upper airway for snoring or sleep apnea.
- 4.
Assessment of treatment results of oral appliance therapy.
- 5.
Assessment of treatment results after sleep apnea surgery in patients with moderate to severe OSA.
- 6.
Evaluation of patients with sleep apnea whose symptoms recur after initial good response to either surgical or dental treatment.
- 7.
Assessment of treatment results in patients on CPAP therapy who have either gained or lost substantial weight to determine the need for continued therapy or for the assessment of adequacy of CPAP pressures in symptomatic patients.
- 8.
Evaluation of patients with systolic or diastolic heart failure with nocturnal symptoms of sleep-related breathing disorders (snoring, disturbed sleep, nocturnal dyspnea) who remain symptomatic despite optimal medical management of congestive heart failure. Additionally, as guidelines, the AASM recommends that patients with coronary artery disease be evaluated for sleep apnea and a sleep study be performed if OSA is suspected. Patients referred for evaluation of significant tachyarrhythmias or bradyarrhythmias should be questioned about symptoms of sleep apnea. A sleep study is indicated if questioning results in a reasonable suspicion that OSA or central sleep apnea (CSA) is present.
- 9.
Evaluation of patients with neuromuscular disorders and sleep-related symptoms.
- 10.
Evaluation of patients with suspected narcolepsy. (A whole night of attended PSG is followed the next day by a multiple sleep latency test.)
- 11.
Evaluation of periodic limb movement disorder.
- 12.
Evaluation of insomnia when the diagnosis is uncertain, when behavioral or pharmacologic treatment fails, or when precipitous arousals occur with violent or injurious behavior.
PSG with video and extended bilateral EEG montage is indicated in evaluating the following: sleep-related epilepsy and potentially injurious sleep-related behaviors; unusual or atypical parasomnias; situations with forensic considerations, and presumed parasomnia or sleep-related epilepsy that is unresponsive to therapy. PSG is not useful in differentiating insomnia associated with dementia from other forms of insomnia, including insomnia with depression, or for establishing the diagnosis of insomnia associated with fibromyalgia or chronic fatigue syndrome. It is not indicated for the routine evaluation of transient or chronic insomnia. PSG is not indicated routinely for the diagnosis or treatment of restless legs syndrome except when the diagnosis is uncertain.
Other Considerations
In choosing a protocol, various factors must be considered. First, subjects must be able to sleep despite the equipment used. As a result, several nights of recording may be needed to determine the overall problem and its severity. Lighter equipment should be used on certain nights to provide information on sleep and sleep-structure changes; on other nights, more invasive equipment (with poorer sleep) may be used to evaluate the “end-organ” changes during the different sleep states. Second, a general diagnostic protocol may not uncover the cause of a sleep disturbance but may indicate the disturbance and its frequency. Clinical information then refocuses the emphasis of the test so that subsequent nocturnal investigations can affirm the etiologic diagnosis
Third, clinical PSG implies monitoring during the total longest sleeping period, which for most people takes place during the night. Daytime nap testing periods are unacceptable because of the circadian distribution of REM and slow-wave sleep, with REM sleep peaking between 3 and 6 a . m . and slow-wave sleep peaking between 11 p . m . and 2 a . m . Care must be taken to avoid prior sleep deprivation and the use of any pharmacologic agent to induce sleep. Sleep deprivation has an impact on the arousal threshold. Moreover, several investigations performed after one night of sleep deprivation have shown significant changes in respiratory control. Pharmacologic agents affect the control of sleep states and stages.
AASM (2007) Scoring Rules
Controversies have arisen since the establishment of the AASM 2007 rules. Comparison of AASM scoring to Rechtschaffen and Kales scoring in adults has shown significant differences in time in minutes and percentage of total sleep time, such that stage 1 increased compared to N1, stage 2 decreased significantly compared to N2, and wake after sleep onset increased significantly, while stage R changes were age-dependent. Such significant differences in results utilizing the two scoring systems imply that previously collected age-dependent normative values for these parameters using Rechtschaffen and Kales methodology cannot be applied and underscores the need to gather new normative data utilizing AASM criteria from large and representative control groups. In children, comparison of the two scoring systems has also shown significant differences: N1 was significantly greater than stage 1, whereas N2 and R were significantly less than stage 2 and REM sleep. Additionally, there was a relatively low concordance between the two systems for scoring the number of stage shifts per hour, and minutes and percentages of N1/stage 1 and N2/stage 2. The most important controversy involves grouping stages 3 and 4 under the label “N3,” particularly in children As mentioned above, association with specific physiologic activities, such as secretion of growth hormone in children, is linked to delta sleep and such linkage is described much better by separating stage 3 from stage 4 non-REM sleep. There is also controversy over the description of sleep-disordered breathing in children. In nonoverweight children with mild hypopneas but important daytime complaints, N3 may be within the expected range, but tabulation of stage 3 and stage 4 separately showed a disappearance of stage 4 in these cases with hyperinflation of stage 3 non-REM sleep. A final commentary is on the absence of a more robust definition of arousals in the 2007 AASM scoring manual, which has been linked to low inter-rater reliability correlation for N2 staging. Understanding arousals may give us a better understanding of the organization of sleep and how it is affected by various disorders.
By contrast, the Cyclic Alternating Pattern (CAP) atlas, which is utilized more in Europe than in North America, places greater emphasis on arousals. CAP is a normal physiologic EEG pattern that is observed commonly during non-REM sleep; periodic recurrence of phasic morphologic patterns are compounded by the alternation of activation phases (Phase A) followed by inhibition phases (Phase B). Three main Phase A EEG patterns are: A1 subtype, with predominantly synchronized EEG activity; A2 subtype, with synchronized and desynchronized EEG activity; and A3 subtype, consisting of primarily desynchronized EEG activity. The Phase A1 subtype is involved in the buildup and maintenance of deep non-REM sleep and may have a protective role in maintaining sleep continuity. Phases A2 and A3 are implicated in maintaining subject arousability. There is a significantly greater opportunity to see Phases A2 and A3 during stage 3 non-REM sleep and Phase A1 during stage 4 non-REM sleep. As Phases A2 and A3 involve scoring of an arousal not necessarily scored with AASM or the Rechtschaffen and Kales criteria, scoring stages 3 and 4 separately may still have advantages compared to combining them under N3.
Sleep Recording
Regardless of the subject’s age, the 10–20 electrode placement system recommended by the International Federation of Clinical Neurophysiology is used for polysomnographic recording during sleep. The 2007 AASM scoring manual recommends at least 6 hours of overnight recording for routine PSG and lists technical and digital specifications, including desirable and minimal sampling rates for various parameters and rules for PSG display and manipulation. Differential alternating-current (AC) amplifiers are utilized to record EEG, EOG, EMG, and ECG. Airflow and effort of breathing can be recorded using either AC or direct-current (DC) amplifiers. Oximetry and esophageal pressure monitoring utilize DC channels. EEG and EOG electrodes should have impedance less than 5,000 ohms.
Minimum parameters to be recorded include specified EEG derivations, EOG derivations, chin EMG, leg EMG derivations, airflow parameters, effort parameters, oxygen saturation, and body position. The recommended derivations are right frontal (F4), right central (C4), and right occipital (O2) electrodes referenced to the contralateral mastoid electrode (M1), with backup electrodes at the left frontal (F1), left central (C3), and left occipital (O1) sites referenced to the contralateral mastoid (M2). There is a small electropotential difference between the front and back of the eyeball, with the cornea positive relative to the retina. The EOG records the movement of the corneoretinal potential difference that occurs in the eye. The EOG derivations are: E1–M2 (E1 is placed 1 cm below the left outer canthus); E2–M2 (E2 is placed 1 cm above the right outer canthus). The eye electrodes allow monitoring of the slow, rolling eye movements commonly associated with sleep onset and the rapid eye movements of REM sleep. If needed, additional electrodes can be added infraorbitally or supraorbitally for either the right or left eye to allow detection of vertical eye movements. EMG from the chin is recorded from three electrodes (one in the midline 1 cm above the inferior edge of the mandible; another located 1 cm below the inferior edge of the mandible and 2 cm to the right; and a third electrode located 1 cm below the inferior edge of the mandible and 2 cm to the left).
PSG in children utilizes the same derivations for EEG, EOG, and chin EMG. However, in children and infants with small head size, the distance between the chin EMG electrodes is often reduced from 2 cm to 1 cm and the distance from the eyes in the EOG electrodes is often reduced from 1 cm to 0.5 cm.
The chin EMG electrodes are important for sleep staging, especially REM sleep. Bilateral leg EMG recordings are standard and are utilized to diagnose periodic limb movements of sleep (PLMS). Upper-extremity EMG electrodes are added if REM sleep behavior disorder, parasomnias, or nocturnal seizures are suspected. Snoring is recorded with a microphone. Airflow through the nose is recorded through a nasal pressure monitor and a thermistor monitors oral breathing. The thermistor measures changes in electrical conductance in response to temperature changes in the probe induced by inspiration or expiration. Several scientific bodies over the last 10 years have emphasized that usage of nasal thermistor or oronasal thermocouple should be forbidden when investigating abnormal breathing during sleep as they do not allow the appropriate scoring of hypopneas. However, when the nasal pressure sensor is not working, the AASM (2007) manual allows the use of an oronasal thermal sensor to score hypopneas.
Respiratory effort is monitored either through an esophageal pressure sensor or through calibrated or uncalibrated inductance plethysmography (RIP). The AASM recommends low-frequency filter settings of 0.1 and high-frequency settings of 15 for RIP. At times, a low-frequency setting of 0.05 to 0.01 Hz can allow visualization of possible flattening in the thorax belt, which can occur along with a flattening of the observed signal from a pressure transducer. In a RIP effort belt, the sensing element is a zigzagging wire that runs the entire length of the belt. Since the sensing element covers the entire circumference, all changes in breathing are detected, regardless of the patient’s position. The chest and abdominal signals can be summed mathematically or represented individually. Paradoxical breathing leads to a significant decrease of the summed signal. RIP effort sensors are better than Piezo sensors. However, poor signals in RIP effort can occur if the belts are applied either too tightly (leading to cross-sectional change of the chest or abdomen) or too loosely (leading to belt movement and overlapping). To obtain good RIP signals, the belts should be placed near the nipple line (midchest) and just above the umbilicus. An alternative sensor for effort is diaphragmatic/intercostal EMG. Integration of this EMG signal can give even better semiquantitative information on increased inspiratory effort. Monitoring of expiratory muscle EMGs can be useful in recognizing the presence of mild sleep-disordered breathing and neuromuscular disorders, and during nasal CPAP calibration as an indicator of the persistence of an abnormal active expiration.
Capnography is the continuous recording of the partial pressure of CO 2 in inspiratory and expiratory gas. A capnometer provides numeric PCO 2 values during inhalation and exhalation. A capnogram continuously depicts PCO 2 as a function of either time or volume. Carbon dioxide monitoring can be performed through either end-tidal capnography (PEtCO 2 ) or transcutaneous CO 2 monitoring. Mainstream or side-stream sampling of the gas is utilized with EtCO 2 . Side-stream sampling is utilized in most sleep laboratories because the mainstream sensor may not be well tolerated during sleep. Transcutaneous CO 2 monitoring (tcPCO 2 ) is an alternative and is accomplished with pH electrodes placed on the skin (usually the chest). The skin is warmed to 42 to 44°F to increase perfusion; CO 2 diffuses through the skin and is measured. The system has to be calibrated for each use and about 30 minutes of placement on the skin are required before stable measurements can be obtained. The sensor must be moved during the night to prevent skin burns. Capnography is useful in detecting pediatric OSA, alveolar hypoventilation, Cheyne–Stokes respiration, and central sleep apnea.
Body position is recorded continuously by an accelerometer but should be correlated with video or technologist notes (if no video recording is available) during calibration to verify that the position stated is confirmed. Single-lead ECG recording and oximetry are standard. Commercially available oximeters give accurate measurements down to 60 to 50 percent oxygen saturation. It is important to know the sensitivity of the equipment; today, good oximeters use 2 heart beats to provide 1 point on the oximetry curve. In infants, transcutaneous oxygen tension and CO 2 tension are measured with electrodes. It is as simple to derive from the finger oximetry signal a finger plethysmography curve that will show similar data and also identify artifactual pulse oximetry values, which commonly occur in association with body movements and artifactually lower oximetry values. By convention, a downward movement of the finger-plethysmography curve indicates sympathetic activation that may or may not be associated with an EEG arousal. Sympathetic activations are seen commonly with events disturbing sleep such as PLMS or sleep-disordered breathing (SDB) events. The potential negative impact on the cardiovascular system of these repetitive autonomic activations is unknown and is being studied.
Sleep Staging Rules
Sleep stages are scored in 30-second sequential epochs based on EEG, EOG, and EMG findings. If two or more stages are seen in one epoch, the epoch is assigned the stage comprising the greatest portion of the epoch. The reader is referred to the AASM criteria for details of scoring sleep in adults and children. Sleep in infants is variable and the following guidelines for sleep stage scoring are recommended. If there are no recognizable sleep spindles, K-complexes, or high-amplitude 0.5- to 2-Hz slow-wave activity, all epochs of non-REM sleep are scored as stage N. Stage N2 is scored for non-REM epochs that contain sleep spindles or K-complexes. If the remaining non-REM epochs have 20 percent or more of slow-wave sleep, they are scored as stage N. Stage N3 is scored for non-REM epochs with more than 20 percent slow-wave sleep. If the remaining non-REM epochs have no K-complexes or sleep spindles, they are scored as stage N. If non-REM sleep has some epochs containing sleep spindles or K-complexes and other epochs contain sufficient slow-wave activity, it is scored as either N1, N2, or N3. Arousals are scored during N1, N2, or N3, or R if there is an abrupt shift in EEG frequency, including alpha, theta, and/or other frequencies exceeding 16 Hz that last at least 3 seconds, preceded by at least 10 seconds of stable sleep. Arousal during stage R needs concurrent increase in chin EMG tone lasting at least 1 second.
Respiratory Rules
The AASM-specified sensor for detecting absence of airflow (apnea) is an oronasal thermal sensor, with a nasal pressure transducer designated as an alternate sensor, if the thermistor is malfunctioning. Determination of an apnea is more accurate when both oral thermistor and the nasal cannula pressure transducer waveforms are associated. The sensor for detecting absence of airflow (apnea) is an oronasal sensor, while the sensor for detection of airflow for identification of a hypopnea is a nasal air pressure transducer with or without square root transformation of the signal. If the nasal pressure sensor fails, then the oronasal thermal sensor should be used for scoring hypopneas. The sensor for detection of respiratory effort is either esophageal manometry or RIP. Piezoelectric sensors and strain gauges are qualitative monitors and are not recommended by the AASM. The sensor for detection of blood oxygen is pulse oximetry with a maximum acceptable signal averaging time of 3 seconds. This is because longer averaging times dampen the signal and lead to underestimation of oxygen saturation. Acceptable methods for assessing alveolar hypoventilation are either transcutaneous PCO 2 or end-tidal PCO 2 monitoring.
For adults, the AASM scoring manual classifies respiratory events based upon various parameters which include event duration, amplitude of the breath, associated desaturation, and/or arousal. An apnea refers to a reduction of 90 percent or more in flow. A hypopnea consists of at least a 30 percent reduction in flow associated with a 4 percent desaturation or a reduction in flow of at least 50 percent associated with a 3 percent desaturation. Apneas and hypopneas are classified as either obstructive, central, or mixed, depending upon the presence of effort in the thoracic or abdominal sensors. Respiratory event-related arousals (RERA) consist of a sequence of breaths lasting at least 10 seconds associated with increasing respiratory effort or flattening of the nasal pressure waveform associated with an arousal; the event does not meet criteria for either apnea or hypopnea. Apneas, hypopneas, and RERA should last at least 10 seconds. Cheyne–Stokes respiration refers to a crescendo–decrescendo pattern of breathing that lasts at least 10 minutes or recurs in at least 5 cycles per hour of sleep. Hypoventilation in adults refers to an increase of more than 10 mm Hg in PaCO 2 during sleep, in comparison to an awake, supine value.
Criteria for respiratory events during sleep for infants and children can be used for children younger than 18 years, but the individual physician can choose to score children aged 13 years or more using adult criteria. Definitions of apneas, hypopneas, and RERAs differ from the adult criteria. For mixed and obstructive apneas, the event duration in children is at least two missed breaths. Central apneas in children are either (1) 20 seconds or more or (2) two or more missed breaths in duration, associated with either arousal or awakening or a desaturation of 3 percent or more. A hypopnea is scored when there is a reduction of 50 percent or more in nasal pressure amplitude lasting at least the duration of two missed breaths and is associated with an arousal, awakening, or more than 3 percent desaturation. A RERA in children consists of flattening and an amplitude drop of less than 50 percent of the nasal waveform or an esophageal pressure (Pes) crescendo of inspiratory effort lasting at least the duration of two missed breaths and is associated with noisy breathing, snoring, and elevation in end-tidal PCO 2 or transcutaneous PCO 2 , or visual evidence of increased respiratory effort. Classifying hypopneas into obstructive, central, or mixed types requires the use of either esophageal manometry or calibrated RIP sensors. Sleep-related hypoventilation is diagnosed when 25 percent or more of the total sleep time is spent with either end-tidal or transcutaneous PCO 2 exceeding 50 mmHg. Periodic breathing is scored if there are more than three episodes of central apnea lasting more than 3 seconds, separated by no more than 20 seconds of normal breathing.
There are controversies concerning these rules, mainly regarding the definition of hypopnea. Limitations of the current definitions have led several groups to present different scoring systems, more particularly in Europe, but also in North America. The dissension is particularly important when scoring sleep-disordered breathing events in children.
Cardiac Event Scoring Rules
These rules provide definitions for various arrhythmias noted during the sleep period. (The ECG sensor is lead II. ) Unlike definitions during the wake period, sinus tachycardia during sleep refers to sustained heart rate exceeding 90 beats per minute, while sinus bradycardia during sleep refers to sustained heart rate of less than 40 beats per minute.
Movement Rules
These clarify definitions for scoring significant leg movement events and series of periodic leg movements (PLM). Electrode placement on the anterior tibialis, amplitude of the baseline deflection, impedance, and sensitivity settings are specified in the AASM scoring manual.
Periodic Leg Movements
A significant leg movement is characterized by an increase equal to or greater than 8 μV in EMG voltage over resting EMG with a duration of between 0.5 and 10 seconds. A PLM series consists of at least four consecutive significant leg movements with an interonset interval of 5 to 90 seconds.
Bruxism
Bruxism is a stereotyped disorder during sleep characterized by either tooth grinding or clenching. Jaw contractions can either be tonic, sustained jaw clenching, or phasic repetitive muscle contractions termed rhythmic masticatory muscle activity (RMMA). Bruxism is scored under the following circumstances: (1) if there are brief elevations of chin EMG that are 0.25 to 2 seconds in duration and if at least three elevations occur in a regular sequence; or (2) if sustained chin EMG duration exceeds 2 seconds.
REM Sleep Behavior Disorder
This disorder is characterized by either or both of the following: sustained muscle activity (tonic activity) in REM sleep in the chin EMG or excessive transient muscle activity during REM sleep in the chin or limb EMG (in a 30-second epoch of REM sleep divided into 10 sequential 3-second mini-epochs, at least 5 of the mini-epochs contain a burst of transient muscle activity). Amplitude is at least four times the amplitude in the background and phasic bursts are 0.1 to 5.0 seconds in duration.
Rhythmic Movement Disorder
This refers to a cluster of rhythmic movements (four or more individual movements of 0.5- to 2-Hz frequency with amplitude twice that of the background EMG activity). Time-synchronized video PSG in addition to polysomnographic criteria is needed for the diagnosis. Bipolar surface electrodes should be placed to record from the large muscle groups involved.
Optional Movements to Score
- 1.
Alternating leg muscle activation (ALMA) series consists of four or more ALMAs (discrete and alternating bursts of leg muscle activity with a frequency of 0.5 Hz to 3.0 Hz).
- 2.
Hypnagogic foot tremor (HFT) consists of a train of four or more bursts with a frequency of 0.3 to 4 Hz.
- 3.
Excessive fragmentary myoclonus (EFM) consists of five or more EMG potentials per minute with maximum EMG burst duration of 150 msec. At least 20 minutes of non-REM sleep with EFM must be recorded.
Portable Monitoring
There are three categories of portable monitoring devices: (1) type 2, comprehensive portable PSG with a minimum of seven channels (EEG, EOG, chin EMG, ECG, airflow, respiratory effort, and oxygen saturation); (2) type 3, modified portable sleep apnea testing that includes a minimum of four channels (at least two channels of respiratory movement or airflow and respiratory movement plus ECG and oxygen saturation); and (3) type 4, continuous single or dual biparameter recording. (Type 1 is the polysomnogram with all regular channels.) A high rate of data loss in the unattended setting has been reported with type 2 devices.
The AASM’s Portable Monitoring (PM) Taskforce issued updated clinical guidelines in 2007 for the use of portable monitoring in the diagnosis of OSA in adults. The taskforce limited their review to type 3 devices. The taskforce guidelines specifically recommend that these studies be performed only in conjunction with a comprehensive sleep evaluation by a board-certified or board-eligible sleep specialist. It is important to understand the indications, limitations, and contraindications for these various tests, as advised by the 2007 and 2003 taskforces.
Multiple Sleep Latency Test
The MSLT ( Fig. 33-6 ) is the gold standard for objective measurement of sleepiness by quantifying the time required for falling asleep. The AASM’s 2005 practice parameters detail the indications and protocol for the MSLT. The MSLT is used as part of the clinical evaluation of patients with suspected narcolepsy or idiopathic hypersomnia. The MSLT is not routinely indicated in the initial evaluation and workup of OSA or in the assessment of change following treatment with CPAP. The MSLT is not routinely indicated for evaluation of sleepiness in medical and neurologic disorders (other than narcolepsy), insomnia, or circadian rhythm disorders. Standardized MSLT protocols must be followed strictly.

The patient completes a 1-week sleep log prior to the MSLT so that the sleep–wake schedule can be assessed. This is important in that cumulative sleep deprivation could affect the MSLT results. Stimulants, stimulant-like medications, and REM-suppressing medications ideally should be stopped 2 weeks before the MSLT. The timing of the patient’s other medications (e.g., antihypertensives, insulin, etc.) should be reviewed by the sleep clinician before MSLT testing in order to minimize any undesired stimulating or sedating properties of the medications. A urine drug screen is performed on the day of the MSLT to detect the presence of drugs that could affect sleep. The MSLT must be performed immediately following PSG recorded during the individual’s major sleep period. The PSG is done in order to detect nocturnal sleep disorders that might artifactually produce short daytime sleep latencies. The test should not be performed after a split night recording. The total sleep time (TST) on the preceding night should be 6 hours or more. This point is crucial because abnormal nocturnal sleep negates the usefulness of the MSLT as a diagnostic tool (e.g., in narcolepsy).
The MSLT consists of five nap opportunities performed at 2-hour intervals. The initial nap opportunity begins 1.5 to 3 hours after termination of the nocturnal recording. A shorter four-nap test may be performed but this test is not reliable for the diagnosis of narcolepsy unless at least two sleep-onset REM periods have occurred. Standardization of test conditions is critical for obtaining valid results. Sleep rooms should be dark and quiet during testing. Room temperature should be set based on the patient’s comfort level. Smoking should be stopped at least 30 minutes prior to each nap opportunity. Vigorous physical activity should be avoided during the day and any stimulating activities by the patient should end at least 15 minutes prior to each nap opportunity. The patient must abstain from any caffeinated beverages and avoid unusual exposures to bright sunlight. A light breakfast is recommended at least 1 hour prior to the first trial, and a light lunch is recommended immediately after the termination of the second noon trial. The conventional recording montage for the MSLT includes central EEG (C3–A2, C4–A1) and occipital (O1–A2, O2–A1) derivations, left and right eye EOGs, mental/submental EMG, and ECG. Prior to each nap opportunity, patients should be asked if they need to go to the bathroom or need other adjustments for comfort. With each nap opportunity the subject is instructed to lie quietly, in a comfortable position, with eyes closed, and try to fall asleep. The same instructions should be given prior to every test. Immediately after these instructions, bedroom lights are turned off, signaling the start of the test. Between naps, the patient should be out of bed and prevented from sleeping. This generally requires continuous observation by a laboratory staff member.
Sleep-onset latency refers to the interval in minutes from lights out to the first epoch of any stage of sleep, including stage 1 sleep. Sleep onset is defined as the first epoch exceeding 15 seconds of cumulative sleep in a 30-second epoch. The absence of sleep on a nap opportunity is recorded as a sleep latency of 20 minutes. This latency is included in the calculation of mean sleep latency (MSL). In order to assess for the occurrence of REM sleep, the clinical MSLT continues for 15 minutes from after the first epoch of sleep. The duration of 15 minutes is determined by “clock time,” and is not determined by a sleep time of 15 minutes. REM latency is taken as the time of the first epoch of sleep to the beginning of the first epoch of REM sleep, regardless of the intervening stages of sleep or wakefulness. A nap session is terminated after 20 minutes if sleep does not occur. Events that represent deviation from standard protocol or conditions should be documented by the sleep technologist for review by the interpreting sleep clinician. The MSLT report should include the start and end times of each nap or nap opportunity, latency from lights out to the first epoch of sleep, mean sleep latency (arithmetic mean of all naps or nap opportunities), and number of sleep-onset REM periods (defined as greater than 15 seconds of REM sleep in a 30-second epoch).
The International Classification of Sleep Disorders categorizes sleepiness as mild (10 to 15 minutes sleep-onset latency), moderate (5 to 10 minutes), and severe (less than 5 minutes). Various physiologic factors affect mean sleep latency. Sleep deprivation, hypnotic ingestion, barbiturate disruption, or sleep fragmentation decreases mean sleep latency. Stimulant ingestion increases mean sleep latency. Age also influences mean sleep latency; young adults have the shortest MSL while prepubescent children have the longest latencies. Anxiety and stress can prolong sleep latency, whereas depression may shorten sleep latency in some patients. Moscovitch and associates reported that 85 percent of narcoleptic subjects have mean sleep latency scores of 5 minutes or less.
Although the MSLT is utilized primarily to evaluate complaints of sleepiness, it can also help to distinguish physical tiredness or fatigue from true sleepiness, because the former can show prolonged sleep latencies. In addition to the mean sleep latency, the number of sleep-onset REM periods, defined as REM sleep occurring within 15 minutes of sleep onset, is determined. The presence of two or more of such periods during an MSLT has been noted in 80 percent of narcoleptic subjects and in 6.6 percent of non-narcoleptic subjects. However, some subjects with typical narcolepsy-cataplexy show no sleep-onset REM periods at first testing; in some cases, the test must be performed for four successive days to demonstrate the presence of two or more sleep-onset REM periods in these subjects. Sleep-onset REM periods have been reported in non-narcoleptic adolescents who were phase-delayed and who were tested at a time when their circadian system had a high probability of initiating REM sleep. In addition, they have been described in 17 percent of normal young adults who were probably chronically sleep-deprived.
Maintenance of Wakefulness Test
The MWT measures the ability to stay awake under soporific conditions for a defined period of time. A valid MWT can be obtained only after the patient has experienced an adequate quantity and quality of nocturnal sleep during the night prior to the MWT and the patient reports feeling normally awake and alert on the day of the test. The findings on the test are correlated with the clinical history. The MWT 40-minute protocol is recommended when objective data are needed to assess an individual’s ability to remain awake. Performance of PSG the night before is optional. The MWT 40 can be used to assess an individual’s ability to remain awake when inability to remain awake constitutes a public or personal safety issue, and to assess the response to treatment in patients with excessive sleepiness.
The recommended 40-minute MWT protocol is published elsewhere. The test should be performed by an experienced technologist. In brief, the MWT consists of four trials performed at 2-hour intervals, with the first trial beginning about 1.5 to 3 hours after the patient’s usual wake-up time. The first trial usually starts at 0900 or 1000 hours. The clinician decides on whether to use sleep logs prior to the MWT or to perform a PSG prior to MWT (i.e., PSG on the night preceding the MWT is not mandatory). The room should be insulated maximally from external light. The light source should be positioned slightly behind the subject’s head such that it is just out of the field of vision, and should deliver an illuminance of 0.10 to 0.13 lux at the corneal level. (A 7.5-W night light can be used, placed 1 foot off the floor and 3 feet laterally removed from the subject’s head.) Room temperature should be based on the patient’s comfort level. The subject should be seated in bed, with the back and head supported by a bed rest (bolster pillow), such that the neck is not flexed or extended uncomfortably. The use of tobacco, caffeine, and any medications by the patient before and during MWT should be decided by the sleep clinician before MWT. Drug screening may be indicated to ensure that sleepiness–wakefulness on the MWT is not influenced by substances other than medically prescribed drugs. A light breakfast is recommended at least 1 hour prior to the first trial, and a light lunch is recommended immediately after the termination of the second noon trial. The conventional recording montage for the MWT includes central EEG (C3–A2, C4–A1) and occipital (O1–A2, O2–A1) derivations, left and right eye EOGs, mental/submental EMG, and ECG. Prior to each trial, patients should be asked if they need to go to the bathroom or need other adjustments for comfort. The subject is instructed to lie still and remain awake for as long as possible, looking directly ahead, and not directly at the light.
Sleep onset is defined as the first epoch exceeding 15 seconds of cumulative sleep in a 30-second epoch. Trials are ended after 40 minutes if no sleep occurs, or after unequivocal sleep, defined as three consecutive epochs of stage 1 sleep, or one epoch of any other stage of sleep. A record is made of start and stop times for each trial, sleep latency, total sleep time, stages of sleep achieved for each trial, and the mean sleep latency (the arithmetic mean of the four trials).
The MWT is most useful in assessing the effects of sleep disorders or of medications upon the ability to remain awake. Compared to the MSLT, the MWT may be more sensitive in detecting treatment effects and in detecting the effects of the manipulation of the previous night’s sleep quality and quantity on daytime alertness. The MWT has been utilized in clinical practice to help determine efficacy of therapy and for the determination of work status in individuals whose occupations carry a high risk of injury should the patient fall asleep (e.g., truck drivers, pilots). The average sleep latency for normal individuals for the 40-minute MWT is 35.2 minutes. Narcoleptic patients have a mean sleep latency of 10 minutes.
Pupillometry
Electronic pupillometry documents pupillary size and changes in diameter. Wakefulness is associated with a large, stable pupil, whereas a constricted, unstable pupil suggests sleepiness. There are no reference standards for this method. A study comparing narcoleptics with normal controls showed greater pupillary instability in narcoleptics with no difference in pupillary diameter.
Vigilance Performance Tests
The psychomotor vigilance task (PVT), a computer-based test, is a chronometric measure of an individual’s reaction to specified small changes in a labile environment. Subjects are instructed to respond to a digital signal on a computer terminal by pressing a key. Errors of omission and commission are recorded. The primary outcome measures of PVT performance, lapses, are defined as reaction times exceeding 500 msec or failure to react. The PVT lapses are believed to represent perceptual, processing, or executive failures in the central nervous system (CNS). Lapses constitute highly sensitive measures of the effects of sleep deprivation, or sleep restriction, on attention and vigilance. Impairment in executive functioning is reflected in another measure, the PVT count of false responses (responding when no stimulus is presented). PVT has been utilized as an objective indicator of cognitive impairment in conditions of chronic sleep restriction, partial sleep loss, sleepiness, and napping. Primary insomniacs responded faster on simple vigilance tasks and slower on complex vigilance tasks than controls. Sleep therapy effectively restored normal performance, suggesting that performance decline with increasing neurocognitive tasks may be an early measure of neurocognitive sequelae of insomnia. The PVT results also correlated with objective measures of sleepiness in studies of OSA patients and sleep-deprived patients. In patients with OSA, vigilance and sustained attention are impaired, as shown by PVT results. The decline in PVT performance is worse in older female subjects, subjects with higher body mass index, more sleep loss (total sleep deprivation, chronic partial sleep restriction, and sleep fragmentation), and higher apnea-hypopnea index. PVT performance may also be a good marker of fatigue.
Sleep deprivation leads to lapses in attention and to slowed responses during driving. Driving simulation tests measure the effects of sleep deprivation and alertness through the use of repetitive tasks and evaluation of declining performance. For instance, Steer Clear is a driving simulator that tests the ability to avoid obstacles on a monotonous drive, without steering or tracking. Using Steer Clear, Findley and co-workers reported that narcoleptic patients and patients with sleep apnea hit more obstacles than did control subjects. Patients who performed poorly on this test manifested decreased vigilance, and these patients actually had a significantly higher number of vehicular accidents. The divided-attention driving task simulator utilizes tracking and visual search in its performance evaluation. The subject must observe visual cues in the periphery and steer. Using this simulator, George and colleagues reported that, as a group, patients with untreated sleep apnea or narcolepsy performed worse than did the control group, although some patients performed as well or better than some controls. These findings indicate that vigilance is not always impaired in sleepy patients.
Videoelectroencephalography-Polysomnography
Videoelectroencephalography-polysomnography (VEEG- PSG) combines simultaneous PSG and video-EEG to evaluate nocturnal events in patients with sleep disorders such as epilepsy, rhythmic movement disorder, non-REM arousal disorders, REM sleep behavior disorder, and psychiatric disorders (e.g., panic attacks or dissociative disorders). This technique provides the capability to record, study, and correlate behavior with neurophysiologic and cardiorespiratory parameters and to detect ictal and interictal epileptiform abnormalities. The AASM Standards of Practice Committee stated that PSG, including video-EEG with an extended bilateral montage, is indicated in the diagnosis of paroxysmal arousals or other sleep disruptions that are thought to be seizure-related when the initial evaluation and results of a standard EEG are inconclusive.
Actigraphy
Actigraphy utilizes small, portable devices that record movement over extended periods of time. A minimum recording of three consecutive 24-hour periods is required. The indications for actigraphy were updated in the AASM 2007 practice parameters. Actigraphy is a valid tool to assist in determining sleep patterns in normal, healthy adult populations, normal infants and children, and patients suspected of certain sleep disorders. It is indicated in the evaluation of patients with advanced sleep-phase syndrome (ASPS), delayed sleep-phase syndrome (DSPS), and shift work disorder. Some evidence suggests its usefulness in evaluating other circadian rhythm disorders, such as jet-lag disorder and non–24-hour sleep–wake syndrome (including that associated with blindness). It can also characterize the circadian rhythms and sleep patterns and disturbances of patients with insomnia and hypersomnia. When PSG is not available, actigraphy can be utilized to estimate total sleep time in patients with OSA.
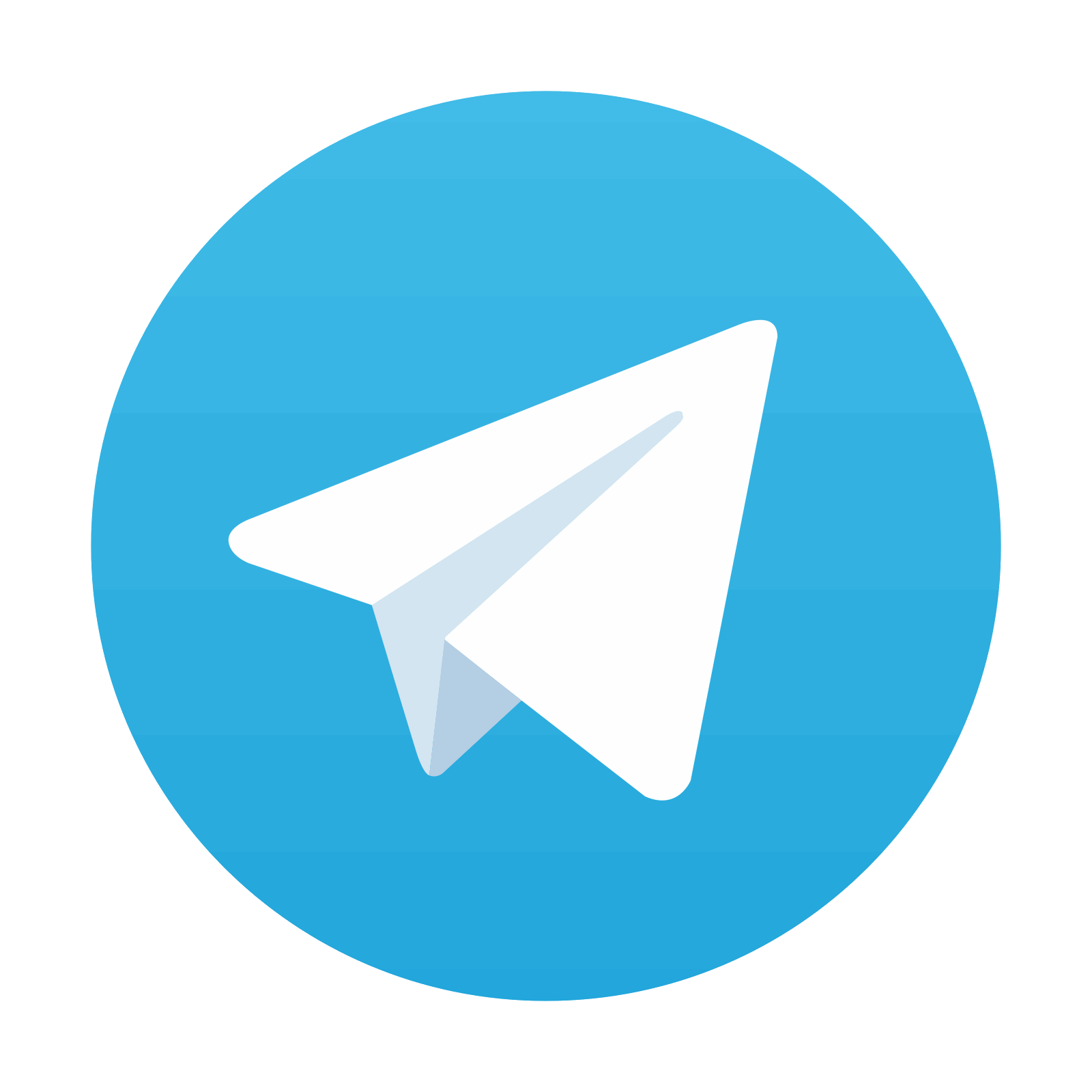
Stay updated, free articles. Join our Telegram channel

Full access? Get Clinical Tree
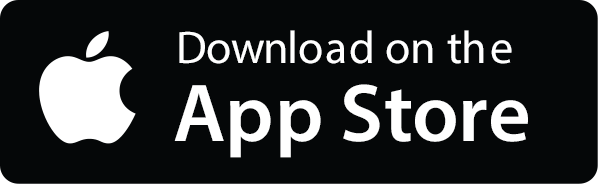
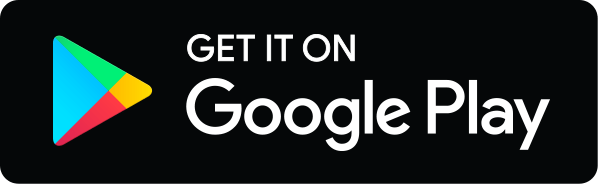