Although the annual incidence of head injury in the general population has not changed in the past 30 years, the number of survivors of severe head injuries has risen, leading to a higher prevalence of patients with PTE (15). Additionally, there has been a high percentage of head injuries of soldiers from the Iraq and Afghanistan wars, with recent studies reporting 7% to 15% of veterans being diagnosed with or having symptoms of TBI (16). Considering this, one can expect a markedly increased number of veterans with posttraumatic seizures.
PATHOPHYSIOLOGY OF POSTTRAUMATIC SEIZURES
Early Seizures
Early posttraumatic seizures, defined as seizures occurring within 1 week of the initial insult, are triggered by the acute trauma or subsequent complications and have to be differentiated from late, unprovoked posttraumatic seizures defining a diagnosis of epilepsy (11). A variety of mechanisms directly related to the initial trauma can trigger symptomatic seizures: The immediate insult after a head injury leads to diffuse axonal injury due to shearing forces and focal brain damage caused by the direct impact to the skull, movement of the brain within the skull (coup and contrecoup), or penetrating wounds (17–20). Secondary axonal injury ensues caused by retraction and swelling of the injured axons with distal wallerian degeneration (21). Subsequent brain necrosis may result from cytotoxic processes such as the release of free oxygen radicals and cytokines and the influx of calcium into open ion channels (18,22). Complications during the acute recovery phase, for example, hypoxia, increased intracranial pressure, hypotension, ischemia, cerebral edema, intracranial bleeding, electrolyte imbalances, or infections, can cause symptomatic seizures several weeks after the injury (Table 28.2) (7,11,23,24). Overall, 90% of seizures within the first 4 weeks after head injury will happen during the first week and more than half of them within the first 24 hours (25,26).
Table 28.2 Definitions
The incidence of early posttraumatic seizures depends on the severity of the injury and is seen in approximately 2.5% of head injury patients in population-based studies and in up to 16% of patients admitted after severe head trauma (7,11,27–33). Moderate to severe head injury, in particular the presence of a subdural or intracerebral hematoma, a depressed skull fracture, a penetrating brain injury, or a cortical contusion, increases the incidence of early seizures up to 30% (11,34,35). With mild head injuries, an early seizure often indicates other neurologic or systemic abnormalities and should warrant further evaluation and observation (23,36–38). A structural lesion from the acute injury—for example, an epidural or subdural hematoma—has to be excluded with imaging. On rare occasions, seizures after mild trauma are seen in the context of a preexisting brain pathology (39,40), known as pseudotraumatic epilepsy (41).
Approximately 10% of patients with early symptomatic seizures develop PTE. While early seizures are associated with an increased risk for late seizures, the increased risk for PTE is not a direct consequence of the early seizures (7,11,27–33). Multivariate analysis in a large population-based study demonstrated that the increased risk of late epilepsy can be explained by other factors, for example, the presence of a cerebral contusion or hematoma, in particular subdural hematoma, skull fracture, or age (11).
The presentation of early posttraumatic seizures is variable. Electrographic seizures without clinical correlate that are only detectable by continuous electroencephalogram (EEG) monitoring occur frequently. Based on a study of continuous EEG monitoring in the intensive care unit (ICU), which included mostly patients with severe head injury, 21 out of 94 patients (22%) had posttraumatic seizures within the first week of injury (42). Only six patients had clinically witnessed generalized tonic–clonic seizures, another four patients showed subtle myoclonic movements with an epileptiform EEG correlate, while more than half of the patients had electrographic seizure without associated clinical signs. Frequent posttraumatic electrographic seizures are associated with episodic increases in intracranial pressure and lactate/pyruvate ratio and may be a target for aggressive antiepileptic management (43).
Approximately 10% to 20% of early seizures evolve into status epilepticus, more commonly seen in children (25,44). Generalized convulsive status epilepticus often accompanies underlying secondary complications, such as ischemia or metabolic imbalance. Focal motor status is most common with subdural hematomas or depressed skull fractures and can be refractory to treatment. Patients with early status epilepticus may have a higher risk for late seizures than patients with self-limited early seizures according to one study (45). Patients with early status epilepticus had a 41% 10-year cumulative risk to develop late seizures compared to a 13% risk after brief symptomatic seizures. It remains unclear if this is related to the underlying lesion, the effect of status epilepticus, or a shared susceptibility for prolonged early seizures and epilepsy in some patients.
Late Seizures and Epileptogenesis
“Through trauma, the brain may be injured by contusion, laceration, compression, and it is well known that these insults may result in epilepsy after a ‘silent period of strange ripening.’ That period lasts for months or years, but these insults produce epilepsy in the case of one individual and not in the case of another… Our attention should therefore be directed toward the discovery of this mysterious difference” (46,47).
The development of PTE after a latent period has been an intriguing observation and challenge for more than half a century. The “strange ripening” in PTE is a rare example of human epileptogenesis, the transformation process of a nonepileptic brain into a brain able to generate recurrent, unprovoked seizures (48). The window between insult and occurrence of these unprovoked seizures offers a unique opportunity to investigate the potential mechanisms leading to epileptogenesis, to identify biomarkers, and to implement therapeutic interventions, which are able to prevent the development of the disease rather than merely suppress the seizures. Most of our understanding of the cellular and molecular mechanisms of epileptogenesis derives from experimental animal models, which are not specifically related to traumatic injury. These studies have shown immediate neuronal and glial responses following an injury, usually leading to significant cell loss in areas of the injured brain and over time. Additional changes involve long-term reorganization of neural circuits including disinhibition and selective loss of inhibitory γ-aminobutyric acid (GABA)ergic neurons and increased glutamatergic excitation. Ultimately, these changes lead to an imbalance between excitatory and inhibitory neurotransmission and increased risk for spontaneous seizures (49–51).
A better understanding of the processes between initial insult and development of epilepsy may provide potential treatment targets to prevent epilepsy. Example of a potentially treatable process are early symptomatic seizures or status after head trauma that could cause progressive changes in neural networks and lead to spontaneous and recurrent late seizures (52). Another example is the highly epileptogenic process of hemosiderin deposition and formation of free iron radicals typical for head trauma (53,54).
Several animal models of PTE—including the fluid percussion model, controlled cortical impact injury model, weight drop injury model, neocortical undercut model, and more recently the blast injury model—have been widely used to investigate epileptogenicity after TBI (33,51,55–57). Based on the fluid percussion model, either a selective loss of hilar interneurons in the dentate gyrus or a relative survival of irritable mossy fibers may lead to persistent granule cell hyperexcitability (58–60). More recent studies have shown that focal brain damage after a single episode of severe fluid percussion injury is able to trigger spontaneous seizures (51,61), which originate from the site of the lesion and become clinically and electrographically more severe over time (62,63).
The controlled cortical impact model utilizes an electronically controlled impactor to apply a focal contusion injury to the cortical surface, allowing for consistent and reproducible results without risk of secondary rebound injuries. Studies have shown that injury to the dentate gyrus leads to mossy fiber sprouting with structural reorganization leading to increased spontaneous excitability (64,65). Recently, a new model of penetrating TBI in rats was developed to more closely mimic wartime TBI. However, it is unclear if this model results in long-term spontaneous seizures (51).
Isolation of a small cortical region by transecting the white matter with a needle leads to epileptiform activity of the pyramidal cell layer in slice recordings in the “cortical undercut” model. Axonal sprouting of the isolated pyramidal cells is associated with an increased number of excitatory connections (66). Furthermore, recent studies have shown a decrease in the GABA output of neocortical fast spiking interneurons that synapse on excitatory postsynaptic targets (67). Early application of tetrodotoxin after the injury blocks action potentials and prevents the development of evoked and spontaneous epileptiform activity in the neocortical slices (68), suggesting that posttraumatic epileptogenesis may be an activity-dependent process. Tetrodotoxin given within 3 days of injury prevented the occurrence of late seizures in an animal model of PTE (69). Several seizure medications have also shown antiepileptic properties in both kindling and posttraumatic animal models of epilepsy including valproate and topiramate with levetiracetam showing antiepileptic properties in a kindling model only (70,71).
The process of epileptogenesis and postinjury recovery overlaps in time and seems to share some basic mechanisms, including neurogenesis, axonal sprouting, and activity dependence (49). Disease-modifying agents and antiepileptic drugs may, therefore, have a positive (or negative) effect on recovery or epileptogenicity. TBI drug trials aimed to improve injury recovery have not looked at late seizures as an outcome measure or as an adverse effect. There are at least data that seizure medications—including remacemide, topiramate, talampanel, lacosamide, and carisbamate—seem to cause no major benefit nor harm on posttraumatic recovery in animal models (49). Additionally, a recent phase II study of levetiracetam in children suffering severe TBI showed it to be safe and well tolerated (72). Effective prevention of epileptogenicity after trauma will require a clear target, appropriate timing, and should not interfere with adaptive processes necessary for functional recovery (50).
TREATMENT OF EARLY AND LATE SEIZURES
A meta-analysis (70) demonstrated effectiveness of phenytoin (RR 0.33; confidence interval [CI], 0.19 to 0.59) and carbamazepine (RR 0.39; CI 0.17 to 0.92) in the prevention of early seizures after head trauma. A Cochrane Database Review of six trials also concluded a beneficial effect of antiepileptic drugs (RR 0.34; 95% CI 0.21 to 0.54) for prevention of early seizures. Based on the Cochrane estimate, for every 100 patients treated, 10 would be kept seizure free in the first week after TBI (73,74). However, seizure control was not associated with a reduction in mortality, neurologic disability, or diminished occurrence of late seizures (pooled RR 1.28; 95% CI 0.90 to 1.81).
On the basis of an analysis of prospective studies (16,75), the American Academy of Neurology has published recommendations on the use of antiepileptic drug prophylaxis in adults with TBI. Their current recommendation is to use short-term phenytoin prophylaxis in adults only with severe brain injury with the goal to prevent early posttraumatic seizures. Phenytoin may be initiated as an intravenous loading dose as soon as possible after the injury. Data on newer antiepileptic medications for the prophylaxis of early seizures after severe head trauma are limited. Levetiracetam has been used for this indication, and several studies have shown similar effectiveness as phenytoin, with fewer side effects (74,76,77). There are limited data to make definite recommendations on the use of antiepileptic drugs to prevent seizures in children. However, there is level III evidence suggesting that prophylactic phenytoin may be considered in children with severe TBI to reduce the risk of early posttraumatic seizures (78–82). There are no data to support the routine use of antiepileptic drugs beyond the first 7 days after the injury (16). Administration of glucocorticoids after brain injury does not prevent late posttraumatic seizures, and early treatment with steroids has been shown to actually increase seizure activity (83).
Medical prophylaxis of late posttraumatic seizures is currently not recommended. For a long time, phenytoin and phenobarbital were thought to be useful in the prevention of late seizures based on observational studies (84,85). Therefore, these medications were routinely prescribed as prophylactic treatment for the most severely injured patients or those experiencing early posttraumatic seizures for 6 months or more (86). However, more recent randomized, double-blind, prospective evaluations of antiseizure prophylaxis with phenytoin, phenobarbital, carbamazepine, and valproic acid consistently found no benefit (75,87–89), irrespective of the choice of antiepileptic drug (89,90). On the contrary, phenytoin can impair cognition in posttraumatic patients (91), and benzodiazepines and barbiturates may interfere with injury recovery (92).
Late posttraumatic seizures beyond the period of the acute insult reflect permanent changes in the brain and signal the onset of PTE (27,93–95). After a first late unprovoked posttraumatic seizure, the vast majority of patients (86%) experienced a second seizure within the following 2 years, with the highest risk after a focal injury or coma for over 7 days (95). Treatment initiation in patients after a first late posttraumatic seizure seems appropriate, even if the traditional diagnostic criteria for epilepsy requiring at least two unprovoked epileptic seizures have not been met (16), and the recent move toward a broader epilepsy definition by the International League Against Epilepsy can be interpreted in support of this approach.
POSTTRAUMATIC EPILEPSY RISK FACTORS
Severity of Head Trauma
The risk of developing PTE depends on the severity of the head trauma and the presence of a penetrating injury. The most commonly used criterion for the severity of closed head trauma employed by epidemiologic studies examining civilian populations is based on the duration of LOC or amnesia and the presence of structural brain damage. The extent of the structural brain damage can be assessed by focal features on the neurologic examination, x-rays showing a depressed skull fracture, or findings on computed tomography (CT) scan (11,13). Correlating with the severity of the TBI and an increased risk of PTE are prolonged coma or amnesia (longer than 24 hours), brain contusion, and intracranial hematoma, in particular subdural hematoma, depressed skull fracture, and dural penetration, and, to a lesser extent, linear skull fractures (93,96,97).
The incidence of late posttraumatic seizures after closed head injury depends on the study population and varies between 1.9% and 25.3% (7,11,27–32). In the series reported by Annegers and Coan (see Table 28.1) (11,98), the RR of seizures was 1.5 (95% CI 1.0 to 2.2) after mild injuries with no increase after 5 years, 2.9 (95% CI 1.9 to 4.1) after moderate injuries, and 17.2 (95% CI 12.3 to 23.6) after severe injuries. In this study, mild trauma was defined by the presence of coma or amnesia for <30 minutes and the absence of a skull fracture; moderate head trauma was classified as coma or amnesia lasting between 30 minutes and 24 hours and the presence of a skull fracture in patients without contusion or intracranial hemorrhage; and severe trauma was classified as coma or amnesia for more than 24 hours and/or brain contusion or intracranial hemorrhage (see Table 28.2). According to a recent multicenter study, the highest cumulative probability for PTE after a 2-year follow-up is seen with biparietal contusions (66%), dural penetration with bone and metal fragments (63%), multiple intracranial operations (37%), multiple subcortical contusions (33%), subdural hematoma (28%), midline shift >5 mm (26%), and multiple or bilateral contusions (25%) (32).
In military personnel who survive high-velocity penetrating head injuries during warfare, the long-term risk of PTE is consistently estimated at 50% in series examining the major wars of the 20th century (99,100). The most recent wars in Iraq and Afghanistan have seen an increase in the numbers of head and neck injuries compared to prior conflicts. However, there has been a lower percentage of penetrating head injuries with an increased number of closed head injuries (101). In combination with the better injury survival to death ratio (7.0 compared to 1.6 for World War II and 2.8 for the Vietnam War), the high percentage of patients with head injury will likely amount to a significant incidence of posttraumatic seizures.
Early Seizures
An early seizure increases the risk to develop late epilepsy by more than 25% after moderate and severe head injury (30,35,93,94,97). Mild TBI with early seizures may not carry an increased risk for late epilepsy (98). Late seizures are more likely to begin early (within the first year) if there has been an early seizure.
Age
The influence of age on the development of early seizures is well documented (2,31,93,94). Children younger than age 5 years are more likely than adults to have seizures within the first hour after mild head injury (28,29,44,102). However, in children, unlike adults, early seizures are less predictive of late seizures (7,94).
Patients older than age 65 years are highly vulnerable to more severe brain damage and late PTE from any type of head injury (11,103). Earlier reports suggested an increased vulnerability for the development of late seizures associated with posttraumatic hippocampal sclerosis in children younger than 5 years of age (104,105). These findings have not been confirmed in more recent case series (106–108).
Genetic Factors
The evidence for genetic influences on posttraumatic seizures is conflicting. Some studies (109) reported a higher incidence of seizures in family members of patients with posttraumatic seizures. Other research has failed to demonstrate a similar relationship (12,110). According to a recent report, a family history of epilepsy and mild brain injury independently contributed to the risk of epilepsy (14), which supports the concept that genetic factors play a role even in symptomatic focal epilepsies (111,112). Additionally, specific polymorphisms in the glutamic acid decarboxylase genes were associated with an increased risk of posttraumatic seizures, both during the early and late phases, further implicating genetics in the development of posttraumatic seizures (113).
DIAGNOSIS
Clinical Seizures
The full spectrum of seizure semiology can be seen after head trauma. The site of injury and the underlying structural damage determine the type of focal manifestations (9,12,114,115). Early posttraumatic seizures are likely to present as generalized tonic–clonic convulsions even in the presence of focal brain damage (28,36,116). Late seizures mostly have a focal onset (9,114,115) and may develop subsequent to early generalized seizures (12). An interaction between the site of injury and the time when seizures are first noticed has been described. Seizures appear earliest after lesions of the motor area, followed by temporal lobe and those in the frontal or occipital areas (117).
Diagnostic Pitfalls
Nonepileptic Posttraumatic Seizures
Head trauma is a risk factor for epilepsy but is also strongly associated with nonepileptic seizure disorders—presenting in the setting of a somatoform disorder, factitious disorder, or malingering (118,119). The diagnosis can be challenging, particularly in patients with mild head injury and normal routine EEG and magnetic resonance imaging (MRI).
A series of 127 adults diagnosed with intractable posttraumatic seizures who underwent video-EEG (VEEG) monitoring (107,108) had typical events captured in 104 patients (82%) during an average length of stay of 4.6 days (standard deviation [SD] 2.4, median 4). Thirty-six out of the 104 patients (35%) were found to have nonepileptic seizures. There was no difference in the mean duration (19.2 years; SD 11.06, median 18) between onset of seizure-like events and diagnostic referral of patients with epileptic and nonepileptic events. Trauma is a shared risk factor for epileptic and nonepileptic events, but only two patients (1.9%) had both epileptic and nonepileptic seizures. A similar study of 72 children who suffered TBI had paroxysmal events captured with VEEG monitoring, with 17% of children having nonepileptic seizures. Nonepileptic seizures were much more common in the mild TBI group with epileptic seizures more likely in the moderate to severe TBI group (120).
The majority of patients had focal-onset epilepsy: 54% presenting with temporal, 33% with frontal, 5% with parietal, and 3% with occipital lobe epilepsy. Secondary generalized convulsions were more common for extratemporal compared to temporal lobe–onset epilepsy (19% vs. 33%, RR 1.25, P = 0.01). Half of the patients with temporal lobe epilepsy had mesial temporal lobe sclerosis, most of them with a head injury after the age of 5 years. Interestingly, six patients who were initially thought to have symptomatic focal epilepsy for many years were subsequently diagnosed with generalized epilepsy, and four out of five had features of idiopathic generalized epilepsy. This illustrates that the onset of idiopathic generalized epilepsy during teenage years and the high frequency of minor head injuries during the same age can easily delay the diagnosis of the underlying epilepsy syndrome with significant impact on the medical management and outcome (Fig. 28.1).
Figure 28.1. A–D:Idiopathic generalized epilepsy versus secondary bilateral hypersynchrony. A and B (upper panel): A 39-year-old male diagnosed with PTE after he was hit with baseball bat at age of 13 years. This patient started to have seizures 1 year later and was diagnosed with PTE. He continued to have sporadic generalized convulsions throughout his life and was treated with phenobarbital and phenytoin and later switched to carbamazepine. After starting pregabalin, he developed concentration difficulties, stuttering speech, and poor concentration and was referred for presurgical workup. VEEG showed interictal generalized spike and waves (A) and polyspikes (B). Myoclonic jerks associated with generalized EEG pattern were recorded. After initiation of valproic acid, he became seizure free. Impression: juvenile myoclonic epilepsy. C and D (lower panels):A 29-year-old female with minor head trauma at age 13 years when she fell on ice and briefly lost consciousness. Onset of epilepsy was at age 14. Outside EEG were reported as generalized spike-and-wave discharges consistent with idiopathic generalized epilepsy. She remained seizure free on carbamazepine for many years. As part of pregnancy planning, she was switched to levetiracetam, and this lead to subacute onset of frequent staring and confusion. EEG showed a generalized spike-and-wave pattern more prominent over the right hemisphere (C) consistent nonconvulsive epileptic stupor, and this nonconvulsive status persisted despite loading her with Valproic acid and levetiracetam, with only transient improvement with Ativan. EEG status eventually resolved after carbamazepine was resumed. Interictal EEG showed generalized and right frontal spikes and polyspikes (D). Impression: posttraumatic focal epilepsy with secondary bilateral hypersynchrony on EEG.
Nonepileptic seizures after head trauma pose a particular challenge to the medical community. The risk of developing epilepsy after a mild head injury is low, and even patients with a normal interictal EEG and MRI may develop epilepsy (Fig. 28.2A). Additionally, nonepileptic seizures are not uncommon after minor head injuries, and a delay in diagnosis and antiepileptic therapy not only interferes with rational treatment but also negatively affects long-term prognosis (121). Similar rates of nonepileptic events were found in both civilian and military veterans undergoing VEEG monitoring over a 10-year period. Interestingly, there was a 5-year delay to diagnosis in veterans compared to 1 year for civilians, resulting in veterans receiving more frequent and prolonged unwarranted antiepileptic drug therapy than do civilians (122).
Figure 28.2. A and B: Epilepsy after minor head trauma. A: A 50-year-old right-handed male presented with a mild head injury in 2002 when he hit his head on an iron beam at work. There was no LOC. He suffered from a first seizure 6 days later and a second event 10 months later. Both were described as generalized convulsions without warning. Three MRI studies including gradient echo sequences for trauma were normal. Several routine EEGs and prior VEEG monitoring (on medication, no events recorded) were normal, and the concern of nonepileptic seizures was raised. The patient was admitted for a second VEEG monitoring assessment. Within 1 day of discontinuing his medications, carbamazepine and phenobarbital, he developed nonconvulsive status epilepticus. The EEG shows prolonged episodes of generalized slowing with superimposed paroxysmal fast activity in the bifrontal region, clinically associated with staring, occasional lip smacking, and diffuse myoclonic jerks. EEG and clinical seizure activity resolved after administration of Ativan. Impression: PTE after concussion without LOC. B: A 50-year-old male with a first seizure 25 years after a mild head trauma. Subsequent CT scan of the brain showed remote left inferior frontal and bilateral temporal contusions. He was treated with antiepileptic medications for 3 years. Two years after stopping the medication, the patient presented with difficulty speaking, brief episodes of unresponsiveness, and ongoing headaches for several days. Routine EEG showed left temporal electrographic seizures lasting around 30 seconds, seen twice during a 30-minute recording, without noticeable clinical changes. Subsequent VEEG showed 6 to 12 seizures per hour, which subsided after temporary burst suppression with midazolam. Impression: late PTE after minor head trauma presenting with nonconvulsive status epilepticus.
There is no clear relationship between the presence of preinjury mental disorders and posttraumatic nonepileptic seizures. However, a high incidence of new psychiatric conditions including posttraumatic stress disorder (PTSD), depression, and anxiety in up to 75% of patients can be noted, often associated with dissociative symptoms and complaints. In some cases, symptoms are not be related to a dissociative problem but may occur in the context of a factitious disorder or due to malingering.
Up to one-third of patients with nonepileptic seizures have a history of head injury, including 78% to 91% having mild injuries (118,119). In the setting of workman’s compensation, a diagnostic distinction between epileptic and nonepileptic events is paramount to establish a likely causal relationship between seizures and injury. Secondary gain from disability or workman’s compensation has a tendency to perpetuate nonepileptic seizure disorders. In regard to disability estimation, dissociative nonepileptic seizures can be as disabling as epileptic events. However, the disability claim is based on a completely different diagnostic entity, which may influence the chance of approval.
Differentiating epileptic and nonepileptic seizures poses a diagnostic challenge in veterans of the wars in Iraq and Afghanistan. The high number of mild head injuries is associated with a small but definitive risk for PTE and also bears a high risk of PTSD, which is often combined with somatic complaints including seizure-like episodes (123,124).
Nonconvulsive Seizures and Status Epilepticus
There is limited information on the incidence of late posttraumatic nonconvulsive seizures and status (Fig. 28.2B). Particularly, patients with persistent cognitive impairment after head injuries are at risk of subclinical seizures, for a variety of reasons. These patients have a 20% risk to develop epilepsy and may not be able to communicate clinical manifestations of seizures. The caregivers may confuse seizure activity with other causes of impaired or fluctuating cognition and consciousness (23). Even patients who are not cognitively impaired do not recognize more than half of their seizures. The accuracy of a seizure account is even lower in patients with focal seizures with impaired consciousness and with nocturnal seizures. These aspects raise further concerns about the accuracy of seizure reporting in the high-risk patient population with cognitive impairment (125).
Testing
Imaging
Imaging of the brain has been extremely helpful to predict the risk of seizures after head injury. CT and MRI of the brain aid in the determination of the underlying etiology, support the diagnosis in patients presenting with seizure-like events, and may delineate a focal lesion amenable to surgery.
The presence of a focal brain lesion is a risk factor for the development of early seizures as well as late seizures (94,126). The odds ratio of PTE with a focal lesion on CT or MRI scan lies between 2 and 6 (30,31). However, the severity and localization of traumatic brain lesions on MRI do not correlate with the risk for late seizures (127). The presence of cortical or subcortical hemosiderin alone was also not associated with an increased risk of late seizures (30). Only more detailed characteristics of the lesion itself, for example, the relation of hemosiderin deposition to surrounding gliosis or a history of a surgical intervention, may provide additional information regarding epileptogenesis and risk of PTE (128). Functional studies, either with diffusion-weighted MRI or with tomography using radioactive tracers may be more specific in predicting the epileptogenic potential of a structural abnormality (129,130). Recent studies have shown the utility of using quantitative diffusion MRI in detecting hippocampal abnormalities ipsilateral to the site of TBI in animal models that correlate with increased seizure risk (131). Additionally, high-field MRI and diffusion tensor imaging may offer a higher sensitivity to detect diffuse axonal injury in patients with mild head trauma (132,133). Furthermore, longitudinal disease evolution in patients with mild TBI is important. A recent study followed 28 patients with mild TBI with interval MRI scans 1 year later and found statistically significant degrees in atrophy in patients with TBI. The changes were most prominent in the anterior cingulate white matter bilaterally, left cingulate gyrus, and right precuneal gray matter (134).
In spite of a diffuse injury to the closed skull, the epileptogenic process manifested itself more often in a vulnerable brain region such as the hippocampus (135). Surgical series of anterior temporal resections report that 10% of their patients presented with trauma as the major risk factor for epilepsy (104–106,136). Approximately half of the patients with PTE undergoing epilepsy surgery present with hippocampal sclerosis, and in one-third of these, additional focal neocortical abnormalities are presents (106,107). However, diffuse abnormalities, often global cerebral atrophy, are common in the cases with hippocampal sclerosis. In surgical patients with PTE, there is always the concern that the obvious MRI lesion only represents the “tip of the iceberg” and that neighboring or remote sites, not visible on MRI, are the actual or future culprit for focal epileptogenicity (137). Patients with a neocortical temporal and extratemporal posttraumatic encephalomalacia who are thought to have surgically amenable focal epilepsy based on surface VEEG recording usually require an invasive evaluation to define the precise focus and extent of the epileptogenic lesion. Orbital frontal and anterior temporal–polar cortices are predisposed to neocortical injury after closed head injuries and seem to represent areas particularly susceptible to epileptogenic brain injury.
Diagnostic EEG and Video-EEG Monitoring
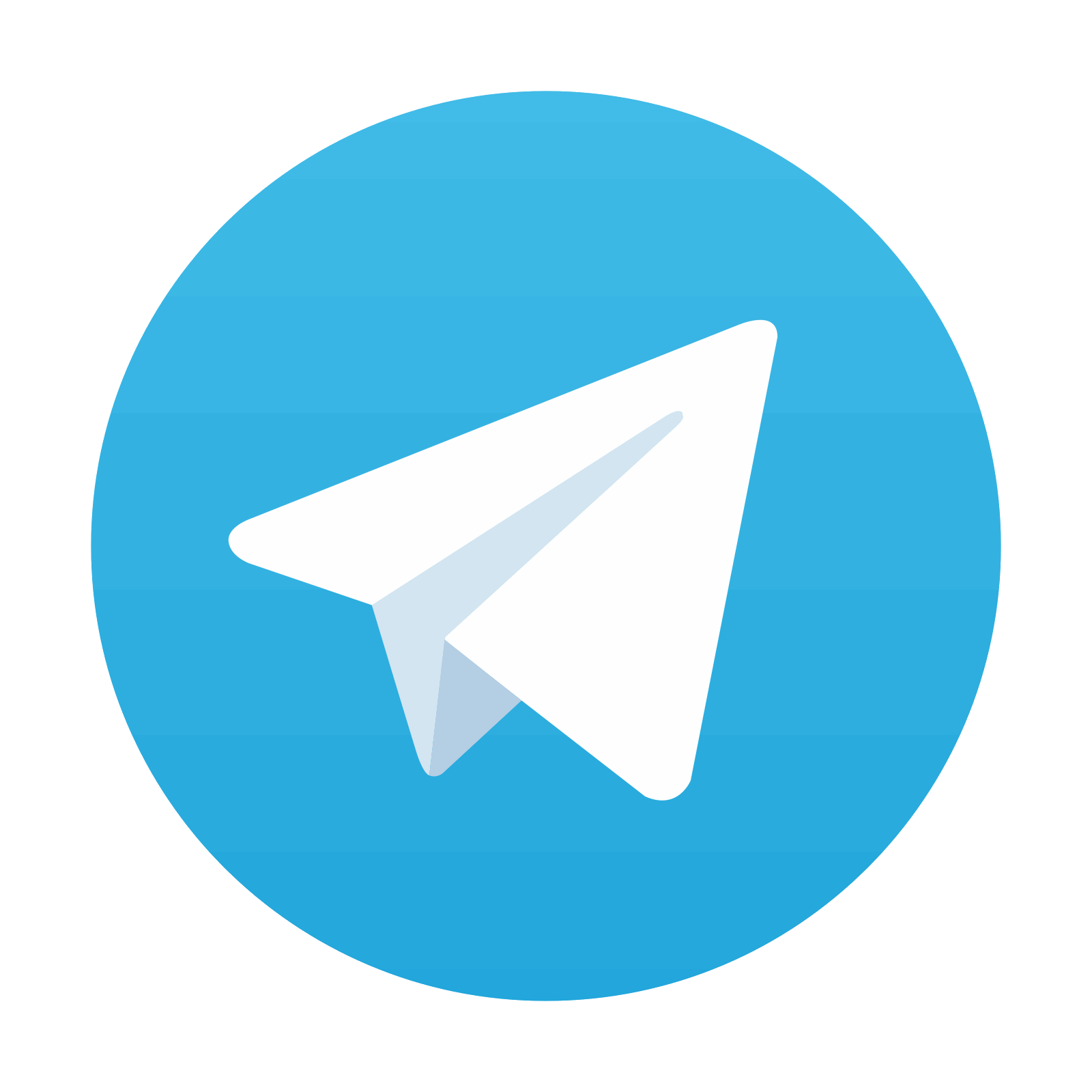
Stay updated, free articles. Join our Telegram channel

Full access? Get Clinical Tree
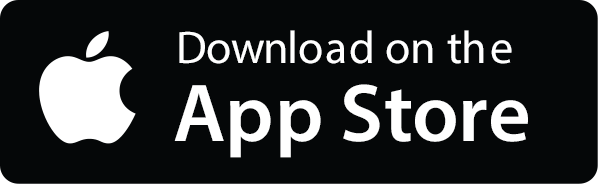
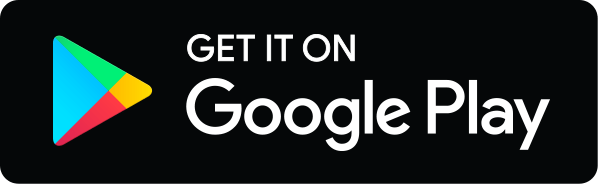