Hypoxia–ischemia
Cytokines
Trophic/growth factors
Drugs/pharmacological agents
Hypoxia (0.5 to 3% O2)
IL-6
EPO, SDF-1
Minocycline/doxycycline
Cobalt
Autologous stroke serum
Diazoxide
Table 11.2
Hypoxic preconditioning increases “potency” of cells
In vitro | In vivo |
---|---|
Greater survival, reduced apoptosis | Increased cell survival and engraftment |
Increased migration in migratory assays | Increased migration in the brain |
Increased angiogenesis | Increased angiogenesis |
Reduction of tissue injury | |
Facilitates functional recovery |
Pathways of Protection
The mechanism by which hypoxia increases cell survival and “potency” involves HIF-1. HIF-1, discovered and characterized by Semenza, is a transcription factor involved in hypoxic adaptation and functions as a master regulator of oxygen homeostasis expressed in all metazoan species analyzed [51–53]. HIF-1 controls oxygen delivery by regulating angiogenesis and oxygen utilization, regulates glucose metabolism, and is involved in redox homeostasis. HIF-1 consists of HIF-1α and HIF-1β subunits, which each contain basic helix–loop–helix-PAS (bHLH-PAS) domains. After heterodimerization, they bind DNA leading to transcription of downstream genes such as VEGF, EPO, sodium–calcium, exchanger −1 (NCX-1). HIF binds to hypoxia-responsive elements and modulates up to 200 genes involved in angiogenesis, mitochondrial biogenesis, cell proliferation, and apoptosis [49, 54]. HIF cytoprotective pathways involve VEGF, EPO, and an HIF-1-sphingosine kinase-sphingosine 1 phosphate-CCl 2 (MCP-1) signaling pathway that mediates HP-induced ischemic tolerance in the brain [55, 56].
HIF-1α protein levels determine HIF-1 transcriptional activity as HIF-1β heterodimerizes with other bHLH-PAS proteins and are present in excess (Semenza et al. 1996). Under conditions of high oxygen tension, HIF-1 is bound by to Von Hippel–Lindau (VHL) protein which targets HIF1a for degradation by the proteasome. The binding of VHL to HIF 1 is dependent upon hydroxylation of a specific proline residue in HIF-1α by the prolyl hydroxylase PHD2 which uses O2 as a substrate. Therefore, its activity is inhibited under hypoxic conditions. One attractive target for pharmaceutical companies is to develop prolyl hydroxylase inhibitors (PHIs) to induce HIF activity for treatment of disorders in which HIF mediates protective responses. For example, a small molecule inhibitor of HIF prolyl hydroxylases was neuroprotective if administered prior to cerebral ischemia in an MCA occlusion model; however, it was not protective if given at the time of ischemia, limiting its clinical usefulness [57].
SDF-1/CXCR Axis
SDF-1/CXCL12 belongs to the CSC chemokine family and is involved in angiogenesis and stem and progenitor cell migration [58]. SDF-1 had two known receptors, CXCR4, the best known, and CXCR7. The CXCR4 receptor is expressed on lymphocytes, monocytes, HSC, and ES cells. SDF-1/CXCR4 is involved in progenitor and stem cell trafficking and homing to sites of injury. Hypoxia increases the expression of CXCR 4 and CXCR7 and the migration, adhesion, and survival of MSCs. CXCR4 mediates the increased migration while both CXCR4 and CXCR7 mediate adhesion and CXCR7 resistance to oxidative stress [35] This effect is mediated via HIF-1 and Akt.
Preconditioning of MSC with SDF-1 decreases their apoptosis, improves their survival and engraftment, and reduces infarction in an MI model [59]. Preconditioning EPCs with SDF-1 increases their angiogenic potential in vitro and in vivo in a hind-limb ischemia model [60]. To date, there are no studies of preconditioning cells with SDF-1 in a stroke or brain injury model.
Other “Preconditoning” Agents
IL-6
IL-6 is a proinflammatory cytokine and, as part of the acute phase response, is associated with recurrent stroke, although it is doubtful that IL-6 per se is causal in recurrent stroke [61, 62]. IL-6 promotes a survival pathway through activation of signal transducer and activator of transcription 3 (STAT3) and is neuroprotective in some stroke models [63]. NSC preconditioned with IL-6 and transplanted into mouse brain 6 h or 7 days after stroke increased angiogenesis and improved functional recovery compared to nonconditioned cells [8]. Transplantation at 6 h also reduced infarct size. These effects were abolished when the preconditioned cells were treated with a small interfering RNA to STAT 3.
Minocycline
Minocycline is an effective neuroprotectant and has been tested in early-phase clinical trials in stroke in humans [64–66]. Sakata et al. preconditioned NSC with minocycline and reported protection against in vitro oxygen glucose deprivation (OGD) [9] Minocycline preconditioning upregulated the transcription factor nuclear factor erythroid 2-related factor 2 (Nrf2) and the antioxidant genes, NQ01 and HO-1, and increased the secretion of the paracrine factors , BDNF, GDNF, nerve growth factor, and VEGF. Minocycline-preconditioned NSC also survived better in ischemic brain when transplanted at 6 h post stroke than nonconditioned cells. Moreover, the minocycline preconditioned NSC reduced infarct size and improved functional outcome at 28 days as measured by the rotarod and beam balance test. Treatment of the preconditioned cells with NRf2 small inhibitory RNA before transplantation blocked this neuroprotective effect. NSC preconditioning with doxycycline, another tetracycline similar to minocycline, also improved survival of NSC in a hypoxia reoxygenation model and also induced upregulation of Nrf2, similar to minocycline [67]. Minocycline is also effective at increasing graft survival when administered to the host. Intracerebral transplantation of porcine fetal neurons cells in the striatum resulted in no graft survival in any animal at 62 days; however, if the animals were treated daily with minocycline, cells engrafted in 40 % of the animals [68].
Autologous Stroke Sera
Another approach is to precondition cells with autologous sera prior to transplantation. This approach leverages the reparative effects of trophic factors in the blood after stroke. In the STARTING clinical trial of autologous MSC in stroke patients, MSC will be ex vivo expanded in culture with autologous stroke patient sera obtained as soon as possible after the stroke and then administered IV [69].
Pre- and Postconditioning the Host Tissue
While there has been extensive work on preconditioning the donor cells prior to transplantation, there has been less work on “conditioning” the host or the recipient tissue. With this approach, the aim is to reduce the acute inflammatory response of the host that impairs engraftment and promotes the death of the graft. Since we are transplanting cells into tissue after an ischemic episode or potentially after a traumatic brain injury, the precise “temporal” term for this type of conditioning would be “postconditioning.”
Remote Ischemic Conditioning
While direct pre- and postconditioning would require access and repetitive occlusion of the middle cerebral artery or other major cerebral artery in patients with stroke, conditioning can be applied at a “distance” or a “remote site” and still provide protection to a distant organ like the brain [70]. Remote ischemic conditioning (RIC) allows a more accessible site like the limb to be used. This can be accomplished with a simple tourniquet or blood pressure cuff repeatedly inflated and deflated on the arm or leg. The intervention is feasible, safe, and well tolerated. There is a large body of evidence that ischemic conditioning is effective in animal models for stroke at reducing infarct size and improving functional outcome when applied before (pre), during (per), and after ischemia–reperfusion (post) [70, 71]. This effect may be mediated by improved cerebral blood flow and cytoprotection of the organ. RIC also seems to dampen and modulate the immune response. There is already clinical trial data suggesting the efficacy of RIC in humans in a chronic conditioning setting to reduce recurrent stroke in high-risk patients with intracranial stenosis and in the prehospital setting in acute ischemic stroke [72, 73].
To date, there has been little published work on this approach. In a rodent MI model, remote ischemic postconditioning achieved by four cycles of 5-min occlusions of the abdominal aorta 1 week post MI, increased the retention of intravenously administered MSC 1 day later in the heart and improved cardiac function at 1 month as measured by echocardiography compared to controls [74]. The remote postconditioning increased SDF-1 in the ischemic heart and transiently in the blood; the beneficial effect of postconditioning was blocked by antibodies to CXCR4, suggesting that the SDF-1-CXCR4 axis was at least partially mediating the effect.
We propose that RIC be tested in preclinical models of stem cell transplantation. Testing with appropriate “sham” conditioning controls should be performed with intravenous, intra-arterial, and intracerebral delivery routes. Another related innovative approach would be to combine HP of transplanted stem/progenitor cells with RIC so that both the donor cells and the host would be conditioned. If preclinical models suggest efficacy, these approaches could be easily translated to patients (Fig. 11.1).
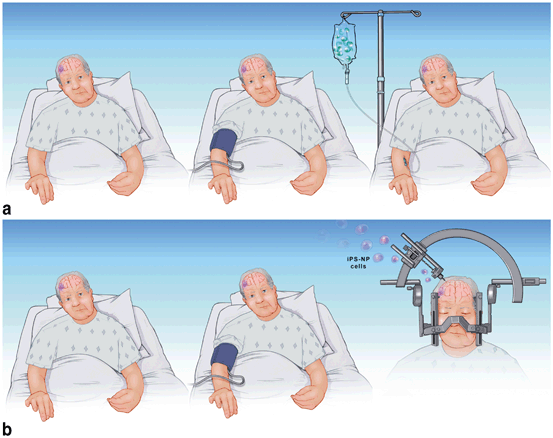
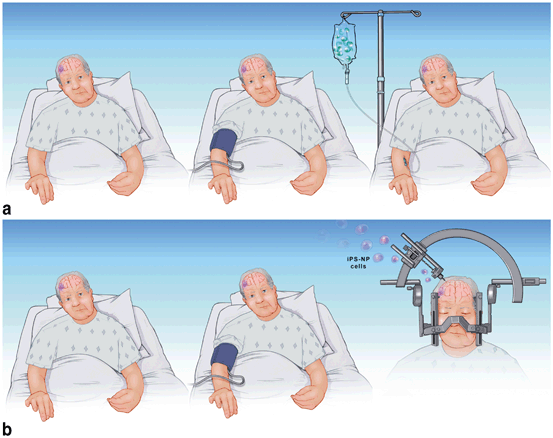
Fig. 11.1
a Remote limb conditioning (RIC) in patient with stroke. In a, the patient undergoes remote limb conditioning with a blood pressure cuff repeatedly inflated and deflated on the arm, 24–48 h after stroke (middle pane) The following day, bone-marrow-derived stem cells are administered intravenously (right pane). b In b, the RIC is applied at around day 5–7 post stroke, then the patient is taken to the operating room (OR, right pane) for stereotactic transplantation of induced pluripotent stem cells differentiated into neural progenitor cells (iPS-NP). The RIC could be continued in the OR and thereafter
Summary
HP of stem and progenitor cells prior to transplantation increases their “potency” in terms of both in vitro and in vivo survival and function. One of the rationales for this approach is the finding that stem and progenitor cells normally reside in a “hypoxic” stem cell niche. Moreover, other “preconditioning agents” such as IL-6, minocycline, and SDF-1 represent alternative approaches. In the future, more attention will be given to “conditioning” the host to prepare the tissue to receive a transplant. Remote limb ischemic conditioning is a safe and feasible approach that might best be combined with hypoxic conditioning of transplanted cells to optimize cell-based therapeutics.
References
1.
2.
Petit GH, Olsson TT, Brundin P. The future of cell therapies and brain repair: parkinsonʼs disease leads the way. Neuropathol Appl Neurobio. 2014;40(1):60–70.CrossRef
3.
4.
5.
Hicks AU, Lappalainen RS, Narkilahti S, Suuronen R, Corbett D, Sivenius J, et al. Transplantation of human embryonic stem-cell-derived neural precursor cells and enriched environment after cortical stroke in rats: cell survival and functional recovery. Eur J Neurosci. 2009;29(3):562–74.CrossRefPubMed
6.
Darsalia V, Allison SJ, Cusulin C, Monni E, Kuzdas D, Kallur T, et al. Cell number and timing of transplantation determine survival of human neural stem cell grafts in stroke-damaged rat brain. J Cereb Blood Flow Metab. 2011;31(1):235–42.CrossRefPubMedCentralPubMed
7.
Rota Nodari L, Ferrari D, Giani F, Bossi M, Rodriguez-Menendez V, Tredici G, et al. Long-term survival of human neural stem cells in the ischemic rat brain upon transient immunosuppression. PLoS One. 2010;5(11):e14035.CrossRefPubMedCentralPubMed
8.
Sakata H, Narasimhan P, Niizuma K, Maier CM, Wakai T, Chan PH. Interleukin 6-preconditioned neural stem cells reduce ischaemic injury in stroke mice. Brain. 2012;135(Pt 11):3298–310.CrossRefPubMedCentralPubMed
9.
Sakata H, Niizuma K, Yoshioka H, Kim GS, Jung JE, Katsu M, et al. Minocycline-preconditioned neural stem cells enhance neuroprotection after ischemic stroke in rats. J Neurosci. 2012;32(10):3462–73.CrossRefPubMedCentralPubMed
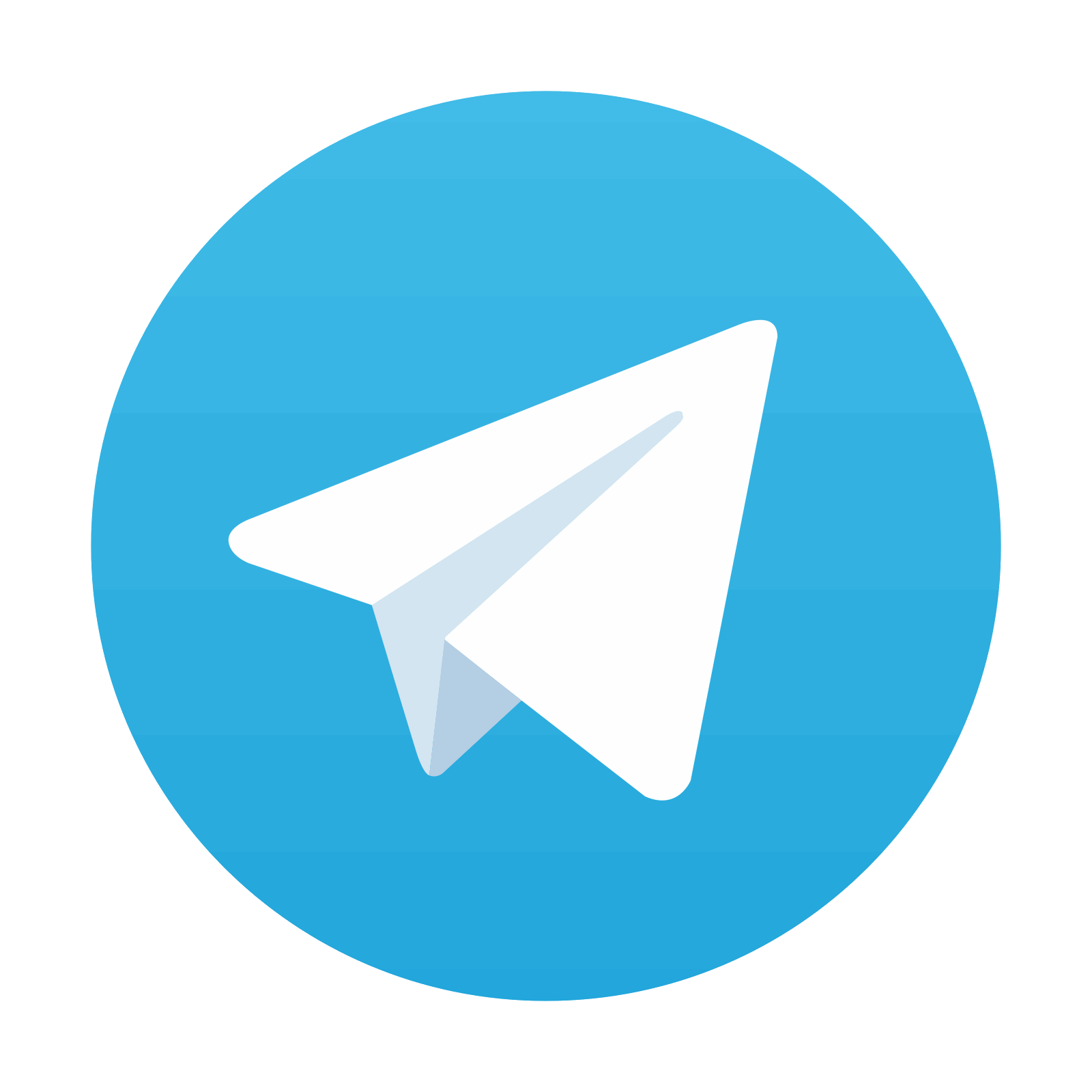
Stay updated, free articles. Join our Telegram channel

Full access? Get Clinical Tree
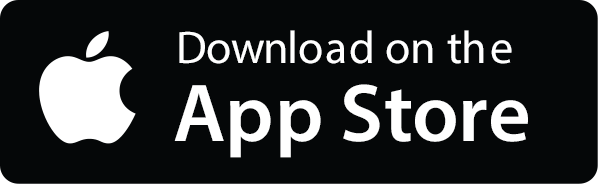
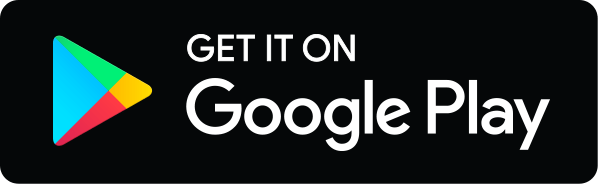