Fig. 1
Salted Tilapia (“koobi”) on sale at a Ghanaian market stall
Dietary salt intake plays a critical role in blood pressure regulation. However the question as to whether high dietary salt intake increases risk of stroke, either indirectly via effects on blood pressure or directly via alternative mechanisms, has received limited attention (Perry 2000).
Hypertension is the major risk factor for stroke in the tropics with rates in excess of 70 % (Wiredu and Nyame 2001; Cappuccio et al. 2000; Akpalu and Nyame 2009). A positive correlation between salt intake and hypertension has been shown consistently (Cappuccio et al. 2006). Diet is a major contributor to variation in the occurrence of hypertension and cardiovascular disease, including stroke, worldwide (Goldstein et al. 2011). A possible direct effect between stroke and salt intake has been evaluated, with data consistent with the hypothesis that a high intake of salt may increase the risk of stroke, independent of effects on blood pressure (Perry and Beevers 1992).
It is well known that there is a direct link between reduction in salt intake and drop in both the systolic and diastolic blood pressure (Cappuccio et al. 2006; Sacks et al. 2001). In West Africa, the lower the salt intake, the lower the blood pressure: a reduction in the average salt intake in the whole community may lead to a small but significant reduction in population systolic pressure (Cappuccio et al. 2006; Adeyemo et al. 2002).
On the basis of the overall evidence from relevant studies, a modest reduction in salt intake of ~3 g/day (50 mmol of sodium) in non-hypertensive individuals would reduce blood pressure by 2 to 4/1 to 2 mmHg (He and MacGregor 2003). In an international study of salt and blood pressure, it was estimated that with a 100 mmol lower daily sodium intake the average decrease in blood pressure from age 25 to 55 would be by 9.0 mmHg for systolic and 4.5 mmHg for diastolic pressure (Intersalt 1988).This reduction, if sustained long-term, would be responsible for a reduction in stroke events of 12–14 % per year (He and MacGregor 2002, 2003). The efficacy of salt reduction in lowering blood pressure levels has been confirmed recently in two small short-term clinical trials in Nigeria (Adeyemo et al. 2002) and Jamaica (Forrester et al. 2005), respectively.
From prospective studies, randomized clinical trials of blood pressure lowering treatment with drugs and short term trials of salt restriction, it is estimated that stroke rates fall by at least 10 % in non-hypertensive individuals whose salt intake was reduced by 50 mmol/day and systolic blood pressure by 1.3 mmHg (He and MacGregor 2003). In hypertensive individuals, the benefit would be much greater. There is no evidence so far to guide to extrapolate these benefits to African populations. It can be argued, however, that the presumptive preventive action could be greater, given the less competing cardiovascular risk in African populations (Cappuccio et al. 2006).
WHO regards population-wide strategies as an integral part of the overall approach to the prevention of cardiovascular disease worldwide, especially those that include dietary modification (WHO 2002; Sacks et al. 2001). There is good evidence that a reduction in salt intake reduces blood pressure (Sacks et al. 2001) and that people of black African origin living in Africa respond well (Cappuccio et al. 2000).
In the western world, it is very difficult to implement successful salt reduction strategies in the population since most of the salt is ingested in processed food. Therefore, interventions should involve the participation of the local indigenous food industry and an attempt by food research institutes in the tropics to look at strategies of desalinating these tasty ingredients in the local cuisine, by soaking salty fish overnight or boiling to remove excess salt (Campbell et al. 2012; Webster et al. 2011).
A recent Cochrane review concludes that despite collating more event data than previous systematic reviews of “Random Controlled Trials” (665 deaths in about 6,250 participants), there is still insufficient power to exclude clinically important effects of reduced dietary salt on mortality or cardiovascular disease morbidity. Estimates of benefits from dietary salt restriction are consistent with the predicted small effects on clinical events attributable to the small blood pressure reduction achieved (Taylor et al. 2011).
Many national and international agencies have acknowledged the role of lifestyle and diet, in particular sodium intake, on blood pressure levels. Diets high in salt are now recognized as one of the leading risks to cardiovascular health in the world as they increase blood pressure in both children and adults (Sacks et al. 2001). Furthermore, a recent meta-analysis of randomized trials has demonstrated that modest reductions in dietary sodium intake are associated with significant reductions in blood pressure in both normotensive and hypertensive subjects and a 20 % reduction in cardiovascular accidents (He and MacGregor 2002, 2011a, b; Asaria et al. 2007).
Furthermore, sodium reduction is noted as one of the most cost-effective and most easily implemented strategies to improve population health (WHO 2007; He and MacGregor 2011a, b; Bibbins-Domingo et al. 2010). Reducing dietary salt is recommended by WHO and many national governmental and nongovernmental health organizations. Some agencies, however, do not promote a reduction in dietary sodium, namely, nongovernmental or commercial organizations such as the Salt Institute, as they are sponsored by either the food or salt industries (WHO 2007, 2010; Webster et al. 2011).
Exposure to excess salt in utero may increase blood pressure in offspring or sodium may increase vascular and cardiac disease in the absence of changes in blood pressure (Bibbins-Domingo et al. 2010; He and MacGregor 2010). The burden of disease associated with excess dietary salt is not only high, but may also be underestimated (He and MacGregor 2003; Campbell et al. 2012). WHO currently recommends a daily consumption of less than 5 g of salt, although some agencies recommend that no more than 1,500 mg of sodium should be consumed per day calculated as 2/3 tsp of table salt (CDC 2009; WHO 2010; Goldstein et al. 2011). In most populations, sodium intake is 5.7 g or more/day after age 5, with many populations consuming and average of over 10 g/day (Brown et al. 2009; Anderson et al. 2010).
Excess sodium intake results in adverse effects beyond blood pressure increase. For example, in a population of overweight adults, a daily intake of sodium greater than 2,300 mg/day was associated with 61 % increase in coronary heart disease mortality, 89 % increase in stroke mortality, and 39 % increase in all-cause mortality over a 19-year period (He et al. 1999). Along with the other sodium-related illnesses (i.e., gastric cancers, kidney stones, etc.), it is clear that the economic costs associated with such illnesses can be substantial. This suggests that even a relatively affluent, well-educated population may have difficulty identifying and avoiding high-salt foods even if they perceive it is a health issue and have chosen to follow a low-salt diet (Campbell et al. 2012).
One factor that may increase hypertension in urban areas may be changes in diet, particularly an increase in salt intake (Poulter et al. 1984, 1990; Simmons et al. 1986). Africans are more sensitive to salt than other ethnic groups, with higher consumption in urban areas (Wilson 1986). Understanding patterns and sources of salt intake may help develop strategies of health promotion that aim to reduce salt intake in sub-Saharan Africa. This strategy is now one of the priorities recognized by WHO to reduce cardiovascular disease in developing countries (WHO 2002).
A universal reduction in salt additives during the manufacturing process has a strong potential to reduce health disparities in vulnerable populations while improving overall population health (Campbell et al. 2012).
2 Snake Venom and Stroke
Most snake bite victims are among the poorest people in the world, so this condition poses an economic threat to populations whose misery is already stretched to the limits. WHO recently recognized snake bites as a neglected tropical disease (Williams et al. 2010).This will hopefully allow a better recognition of cases and reduce the impact burden of this human threat (Williams et al. 2010).
Venomous snake species are distinguished by characteristic morphology, including the size, color patterns, and shape of their head, tail, and fangs. However, misidentification of the offending snake by the victim or even by experts is common. When available, antigen detection in the serum of the victim by immunodiagnostic tests may be of value for indirect identification of the snake (Habib et al. 2001; Del Brutto and Del Brutto 2012).
Venomous snakes are classified into four families: Viperidae, Atractaspididae, Elapidae, and Colubridae. In Africa, the most dangerous snakes are the mambas (Dendroaspis sp.), the Egyptian cobra (Naja haje annulifera), the puff adder (Bitis arietans), and some carpet vipers (Echis sp.) (Enwere et al. 2000; Habib et al. 2001; Michael et al. 2011) (Fig. 2).
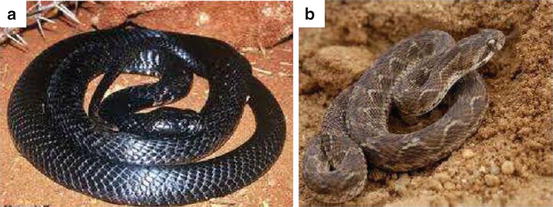
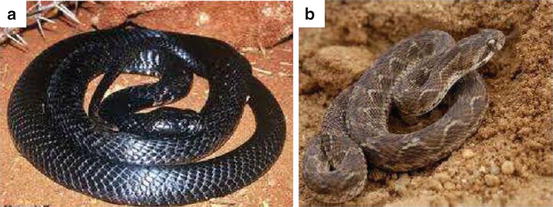
Fig. 2
(a) Naja nigricollis nigricollis; (b) Carpet viper. Reproduced with permission from “Amphibians & Reptiles of Morocco and Western Sahara”, available from https://www.moroccoherps.com (accessed January 03, 2013)
In resource-poor countries, snake envenoming has been considered an occupational disease mainly affecting farmers and hunters. Most bites occur when snakes are inadvertently stepped on and they feel threatened, as few snake species are aggressive to humans (Njoku et al. 2008; Michael et al. 2011; Del Brutto and Del Brutto 2012).
2.1 Snake Venom Composition
Snake venom is a mixture of proteins, carbohydrates, and other substances (Doley and Kini 2009). The venom is produced in the venom apparatus, consisting of glands, muscles, ducts, and fangs, which are located behind and below the eyes of the snake (Warrell 2010). Most venom components are primarily directed to immobilize and kill the prey. Relevant components of the venom have cytotoxic, hypotensive, neurotoxic, or anticoagulant effects (Del Brutto and Del Brutto 2012). The composition of the venom is species-specific, i.e., neurotoxins most often predominate in the venom of elapids, while cytotoxic and anticoagulant/procoagulant substances are most often found in the venom of vipers and colubrids (Doley and Kini 2009).
Cytotoxic enzymes (phospholipases A2, metalloproteinases) activate pro-inflammatory mechanisms that cause edema, blister formation, and local tissue necrosis, which are common occurrences at the site of a venomous snake bite. These enzymes also favor the release of bradykinin, prostaglandin, cytokines, and sympathomimetic amines, which are also responsible for pain (Vaiyapuri et al. 2010; Teixeira et al. 2009).
Other venom toxins, like aminopeptidases, can affect physiological functions causing, for example, systemic hypotension (Vaiyapuri et al. 2010). Some peptides of the venom act as angiotensin-converting enzyme inhibitors, causing a drop in arterial blood pressure (Joseph et al. 2004). Other toxins, such as safarotoxins and endothelins, are potent vasoconstrictors of coronary arteries and may cause myocardial ischemia or cardiac arrhythmias (Kochva et al. 1993).
Neurotoxins are major components of some snake venoms, particularly elapids. These toxins do not cross the blood–brain barrier, but cause paralysis by affecting the neuromuscular transmission at either presynaptic or postsynaptic levels (Lewis and Gutmann 2004). Presynaptic neurotoxins are phospholipase A2 complexes, called β-neurotoxins, which inhibit the release of acetylcholine from the presynaptic terminal. Such inhibition may be irreversible as these toxins interfere with the formation of new acetylcholine-containing synaptic vesicles (Lewis and Gutmann 2004).
Examples of β-neurotoxins include taipoxin, paradoxyn, trimucrotoxin, viperotoxin, pseudocerastes, textilotoxin, and crotoxin (Doley and Kini 2009). On the other hand, postsynaptic neurotoxins are three-finger protein complexes, called α-neurotoxins, which have a curare-like mechanism of action, causing a reversible blockade of acetylcholine receptors. The best characterized α-neurotoxins are irditoxin. Some venoms contains both α- and β-neurotoxins, producing complex blockade of neuromuscular transmission (Doley and Kini 2009; Del Brutto and Del Brutto 2012).
Snake venom toxins may also interfere with blood coagulation and cause hemorrhages or thrombosis. Metalloproteinases activate factor X and serine proteases are potent prothrombin activators. In addition, a number of non-enzymatic proteins, called snake venom C-type lectins and some of the three-finger toxins, have anticoagulant or procoagulant activity and may be either agonists or antagonists of platelet aggregation (Doley and Kini 2009).
Paradoxically, some of the components of the venom of snakes may also have immunomodulatory, anti-inflammatory and anti-tumoral effects and are currently under investigation as potential therapeutic agents for human diseases (Koh et al. 2006).
Another toxin, ancrod, a serine protease derived from the venom of the Malayan pit viper (Calloselasma rhodostoma), has been used for years for therapy of patients with acute ischemic stroke because of its defibrinogenating properties in vivo by converting plasma fibrinogen into a soluble, non cross-linked form of fibrin (Levy et al. 2009; Olinger et al. 1988). Because of this property, the toxin ancrod has been used in clinical trials for the treatment of thrombotic stroke. Some other snake venom toxins are used in clinical laboratories for the assay of hemostatic parameters (Marsh and Fyffe 1996).
2.2 Local Symptoms and Signs of Snake Bite
Considering the systemic manifestations of snake bite, and specifically stroke, an understanding of the local features of envenomation is important. Pain is the earliest symptom after a viper’s bite, but may be minor or absent after envenoming by many elapids (Teixeira et al. 2009). This is followed by swelling, blister formation, and necrosis of the skin and subcutaneous tissue. These manifestations may be complicated by tourniquet applications. The entire limb may become edematous, and a compartmental syndrome may develop (Vigasio et al. 1991). Pain and local necrotic symptoms are especially severe after viper and colubrid bites (Warrell 2010; Njoku et al. 2008). Venom ophthalmia is a particular syndrome characterized by ocular pain, hyperemia, blepharitis, and corneal erosions, which may occur when the venom of spitting cobras enters the eye of the victims (Chu et al. 2010).
2.3 Systemic Manifestations of Snake Bite
Some venom components cause vasodilatation and capillary leakage, which, by themselves or together with the hypovolemia resulting from acute bleeding, may cause arterial hypotension and shock (Otero-Patiño 2009). Cardiac ischemia or arrhythmias because of constriction of coronary arteries may occur after the bite of burrowing vipers due to safarotoxins in their venom (Kochva et al. 1993). Acute renal failure may also occur in severely envenomed snake bite victims and may be related to hypovolemic shock, consumption coagulopathy, rhabdomyolysis, and direct nephrotoxicity causing tubular necrosis (Sitprija 2008).
Pituitary hemorrhages, causing acute hypopituitarism, may occur after the bite of Russell’s vipers due to the presence of hemorrhagins in their venom (Antonypillai et al. 2011).
Clinical manifestations related to thrombotic and hemorrhagic complications are common in envenomed snake bite victims, particularly in those bitten by vipers and colubrids. The venom of these snakes contains toxins that alter the coagulation system and the function of platelets in different ways, representing the basis for thrombosis and hemorrhages at different locations, including the upper and lower digestive tract, the lungs, the retroperitoneal space, the genitourinary tract, and the nervous system (Markland 1998).
2.4 Neurological Complications of Snake Bite
Neurological signs and symptoms after a venomous snake bite are most often related to the toxic effects of the venom, i.e., anticoagulant/procoagulant activity or neurotoxicity. As noted previously, both effects may be combined in the same venom causing complex and severe neurological damage (Doley and Kini 2009).
Some patients develop neurological complications related to cerebral hypoxia, which, in turn, is related to the hypotensive shock that may accompany some snake bite envenoming (Del Brutto and Del Brutto 2012). The neurological symptoms include drowsiness, confusion and convulsions (Bashir and Jinkins 1985). Subarachnoid hemorrhage has been reported in three of 115 patients from Nigeria, two of whom died (Warrell et al. 1977). A flaccid dysarthria has been reported after snake bite amongst other rare manifestations (Vir et al. 2010).
2.5 Cerebrovascular Accidents Following Snake Bite
Little is known about the prevalence of stroke among snake bite victims. Much of the available information on the occurrence of stroke following a venomous snake bite is derived from case reports or small cohorts. Most reports of stroke cases following snake bites derive from rural areas of developing countries where neuroimaging is not available (Bashir and Jinkins 1985; Del Brutto and Del Brutto 2012; Warrell et al. 1975, 1977).
Seven patients with cerebral infarction have been reported among 109 snake bite victims (6.4 %) from Martinique, but there is no information on the computerized tomography (CT) findings of these patients (Thomas et al. 1998). Fifteen patients with intracranial hemorrhages among 294 cases of snake bites (5.1 %) have been reported from Ecuador; though the diagnosis was only clinical (Kerrigan 1991).
A more recent hospital-based study showed a 2.6 % prevalence of cerebrovascular complications among 309 snake bite victims attending a general hospital in Ecuador (Mosquera et al. 2003). This report may be relatively accurate because neuroimaging studies were performed in every patient presenting with deterioration of consciousness or focal neurological signs. Brazilian studies including 322 patients bitten by venomous snakes reported only one stroke case (0.3 %) (Bucaretchi et al. 2001).
In Africa, data is limited and numbers of stroke event following venomous bites are few but lethal. It is possible that cases of cerebrovascular accidents die before getting the necessary help (Warrell et al. 1977; Habib et al. 2001; Njoku et al. 2008; Michael et al. 2011) and data is lost as traditional norms are followed by burying the victims without a post-mortem examination.
2.6 Cerebral Infarction
Well documented cases (25) of cerebral infarctions following snake bites have been reviewed (Del Brutto and Del Brutto 2012). The offending snake was identified as a viper in 23 of these cases, and only one patient was bitten by an elapid. All but two patients had cortical infarcts which were located in multiple cerebral arterial territories in 16; only two patients had hemorrhagic transformation of the infarcts. All patients had pain and local signs at the bite site, but only five developed systemic manifestations (hypotensive shock, renal failure, rhabdomyolysis). Laboratory tests were abnormal in 13 of 16 cases. Most common abnormalities included low platelet counts, increased fibrinogen levels, and prolongation of prothrombin and thromboplastin times. Snake antivenom was administered to 24 of the 25 patients. Only three patients died, 16 were left with mild to severe sequelae, and six recovered completely (Lee et al. 2001; Numeric et al. 2002; Thomas et al. 2006; Mosquera et al. 2003; Cole 1996; Hung et al. 2002; Narang et al. 2009; Bashir and Jinkins 1985; Boviatsis et al. 2003; Merle et al. 2005).
The cause of ischemic stroke in snake bite victims is controversial. Proposed aetiopathogenesis include the presence of venom toxins causing hypercoagulability and endothelial damage, immune-mediated vasculitis, systemic hypotension (Hoskote et al. 2009; Mugundhan et al. 2008) and arterial thrombosis (Bashir and Jinkins 1985).
That infarctions are most likely related to prothrombotic effects of the venom and to endothelial damage is supported by the finding of multiple cerebral infarctions in more than 60 % of cases. None of the patients had infarctions in watershed areas, which are common in hypotensive shock, and vasculitis seems unlikely as many patients developed the infarct within the first few hours after the envenoming (Gawarammana et al. 2009). Angiography, performed in some of these cases, has shown occlusion of large and medium size intracranial arteries, a finding that is uncommon in immune-mediated vasculitis or in hypotensive shock (Bashir and Jinkins 1985; Lee et al. 2001).
2.7 Cerebral Haemorrhage
Well-documented cases of snake bite causing bilateral intracranial haemorrhage have been reported (see for review Del Brutto and Del Brutto 2012; Bartholdi et al. 2004; Kitchens and Eskin 2008; Kouyoumdjian et al. 1991; Midyett 1998; Yap and Ihle 2003).
Nine patients had lobar haemorrhages, which were multiple in five cases. Some of the lobar bleedings extended into the ventricular system or the subarachnoid and subdural spaces. One patient had a cerebellar haemorrhage, one an epidural hematoma, and the other a subarachnoid haemorrhage. Of interest, in CT scans the attenuation coefficient of many of these bleedings was rather low, and haemorrhages appeared almost isodense to the brain parenchyma on CT, a finding that could be explained by the associated severe anemia (Del Brutto and Del Brutto 2012).
The offending snake was a viper in ten patients and an elapid in the remaining two. Eleven patients had abnormal laboratory tests, including low platelet counts, increased clotting times, increased prothrombin and thromboplastin times, and decrease fibrinogen levels. Antivenin was given to all but one patient. Despite therapy, nine patients died and the other three were left with irreversible sequelae. In all cases, intracranial haemorrhages were related to abnormalities in hemostatic factors ranging from decreased platelets to a severe consumption coagulopathy (Del Brutto and Del Brutto 2012).
2.8 Treatment of Cerebrovascular Complications Following Snake Bite
The use of first aid measures in the management of snake bite by patients in rural communities in Africa is a popular practice. Common first aid measures employed include tourniquet (ropes, pieces of cloth), use of the “black stone” (se further), and application of traditional medicine and incision of site of bites (Madaki et al. 2005; Njoku et al. 2008; Michael et al. 2011). The use of first aid measure did not prevent spread of the venom. However, the use of the tourniquet, traditional herbs and the “black stone” appears to have beneficial effects by reducing the average antivenom requirement of patients (Madaki et al. 2005). On the other hand, current evidence suggests that, in addition to the general measures, ancient practices such as the use of tight tourniquets, as well as incision, sustained suction, or the use of herbal medicines to the wound should be discouraged (Warrell 2010; Gold et al. 2002; Michael et al. 2011).
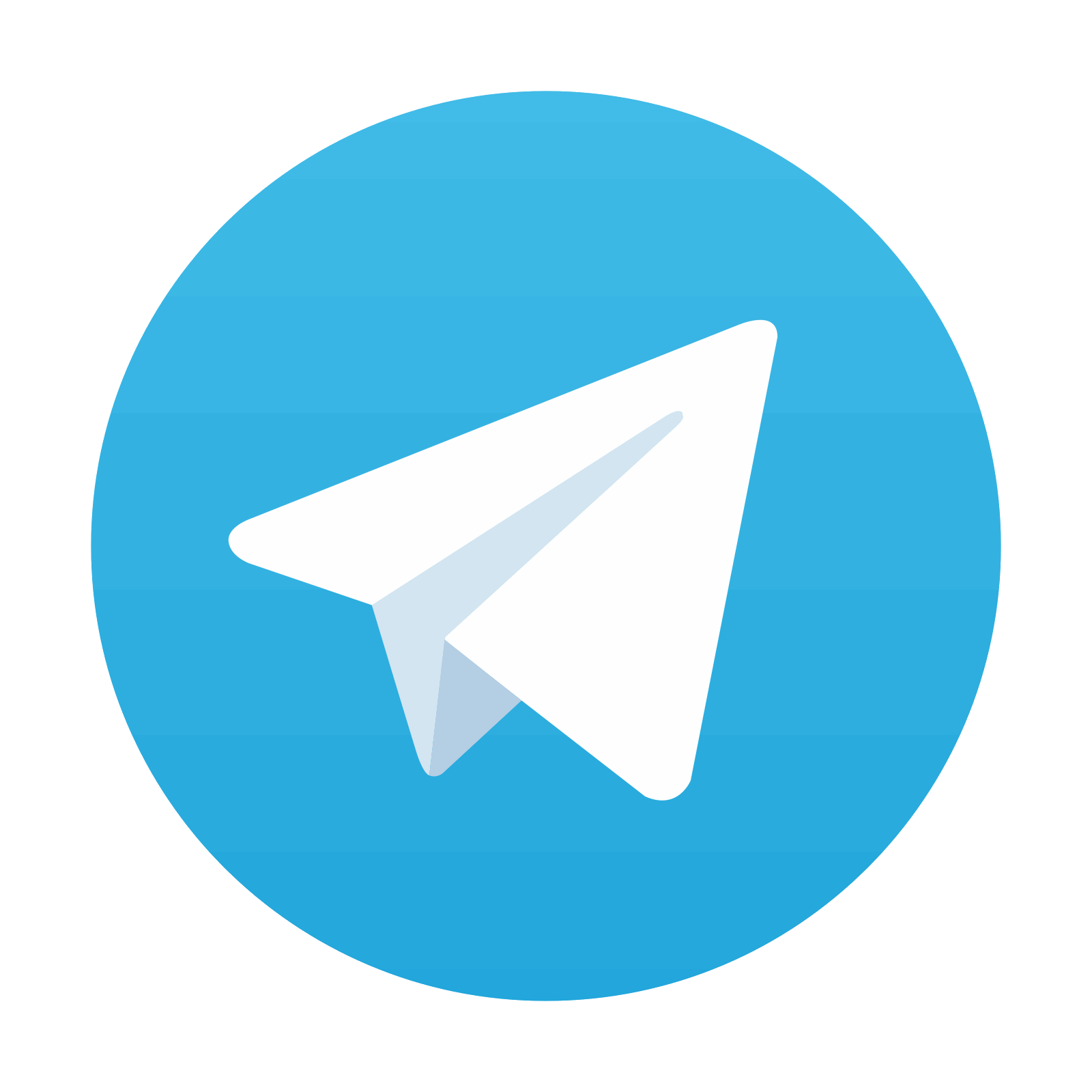
Stay updated, free articles. Join our Telegram channel

Full access? Get Clinical Tree
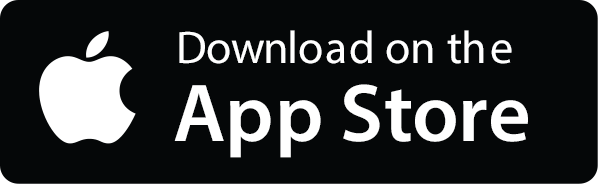
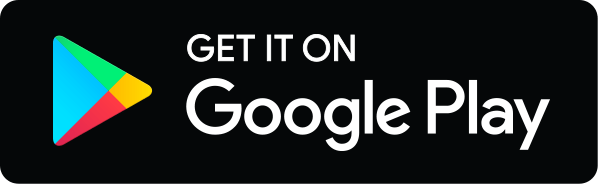