Keywords
Nerve root, radiculopathy, plexus, plexopathy, brachial, lumbosacral
Anatomy
In total, there are 31 spinal nerve root pairs: 8 in relation to the 7 cervical vertebrae, 12 thoracic, 5 lumbar, 5 sacral, and 1 coccygeal. In general, one nerve root is adjacent to each vertebra, but the cervical spine has an extra set of nerve roots accompanying the seven cervical vertebrae. At the cervical level, the first seven roots exit the spinal column above their respective vertebrae. The C8 root exits below the seventh cervical vertebra, and all nerve roots caudal to this level leave the spinal canal below their respective vertebrae.
At each spinal level there is a ventral (anterior) and dorsal (posterior) root exit or entry zone, from or to the respective portions of the spinal cord. As shown in Figure 12.1 , the anterior horn of the homologous spinal cord level contains the cells of origin for the anterior motor root, while the primary sensory neuron cell bodies are within the dorsal root ganglion. The anterior or ventral (somatic motor) and posterior or dorsal (somatic sensory) roots join in the intervertebral foramen to form a combined motor and sensory spinal nerve, which then bifurcates into anterior and posterior primary rami. The larger anterior primary rami are the most important, particularly those in the cervical and lumbosacral regions, which innervate the muscles of the extremities (myotomes) and are the source for the sensory innervation of the skin (dermatomes). The posterior primary rami innervate the paraspinal musculature and the skin of the neck and posterior trunk.

Diagnostic Procedures
Magnetic Resonance Imaging
Magnetic resonance imaging (MRI) is the procedure of choice to evaluate and characterize lesions affecting spinal nerve roots. With disc protrusions, MRI enables visualization of effacement of the nerve root by the herniated nucleus pulposus. In children, MRI imaging is useful to identify and characterize intra- and extramedullary lesions causing nerve root compression and radiculitis.
Electromyography
Nerve conduction studies are generally normal in radiculopathies. Intraspinal lesions, such as those seen with a herniated nucleus pulposus, occur prior to the bifurcation of the spinal nerve, affecting both its anterior and posterior primary rami. With compression of a single nerve root, motor nerve conduction studies tend to be normal because typically only a fraction of nerve fibers in the root undergo axon loss, and because of the overlapping segmental innervation of many muscles. At the same level the sensory nerve action potentials (SNAPs) are also normal, because the dorsal root ganglion is distal to the site of the damage, residing within the intervertebral foramen ( Figures 12.2 and 12.3 ). This is true for all cervical and lumbosacral root levels except L5, where up to 30% of dorsal root ganglia are intraspinal and therefore vulnerable to compression from disc extrusion, resulting in an abnormal superficial peroneal SNAP amplitude. Electromyography (EMG) in radiculopathies is more sensitive to changes affecting a single nerve root, showing signs of denervation (spontaneous discharges such as fibrillation potentials), in the paraspinal muscles as well as other muscles of the myotome corresponding to that spinal level.


In contrast, when the lesion is distal to the intervertebral foramen, the posterior ramus is spared and EMG of the paraspinal muscles is normal, while EMG of limb muscles shows the characteristic changes of denervation. One important caveat to the EMG assessment of radiculopathies in children is that, when they are anxious or upset, it can be difficult to obtain enough paraspinal muscle relaxation to adequately evaluate for abnormalities.
Children with peripheral nerve trauma may have both nerve root avulsion and plexus injuries. In the pediatric age group, therefore, EMG findings compatible with a primary plexus lesion (i.e. absent SNAPs) do not exclude additional damage at the nerve root level ( Figure 12.4 ). A combined process should always be considered in patients with possible proximal nerve lesions.

Cervical Radiculopathies
Cervical nerve root lesions in children are rare, but tend to mimic the signs and symptoms of cervical radiculopathy in adults: local or radiating pain and focal weakness, with or without focal loss of the deep tendon reflexes. In adults C7 root lesions are most common, followed by C6 root lesions, but in children there are too few such lesions to judge their relative frequency.
Etiology
Most cervical radiculopathies in childhood follow trauma or traction injuries (see Case Example 12.1 ), but tumors, inflammatory lesions and other extramedullary lesions can also cause nerve root compression or injury ( Box 12.1 ).
A 15-year-old boy, who participated on his high school wrestling team, developed intermittent numbness and tingling in his right shoulder, radiating down his upper lateral arm, and lasting 15 minutes at a time. Three months earlier he had had an episode of “whole arm” numbness, and he had a history of multiple right shoulder dislocations requiring surgery some years earlier. No specific neurologic abnormalities were identified on examination.
He was referred to the EMG laboratory. The right median, ulnar, radial, medial, and lateral antebrachial cutaneous sensory amplitudes and distal latencies were normal. Similarly his right median and ulnar motor conduction studies and associated F-latencies were normal. Concentric needle EMG demonstrated mildly reduced recruitment in several C6–7 innervated muscles including biceps, deltoid, triceps, and brachioradialis. There was no abnormal spontaneous activity in these muscles or in the right C5–6 paraspinals. These findings were suggestive of a mild patchy chronic right C6–7 radiculopathy with no significant signs of active or chronic denervation. He was restricted from wrestling, as this was thought to be the pathophysiologic mechanism for his injury, and his symptoms resolved.
Comment
Cervical radiculopathies are very rare in the pediatric population but may be triggered by athletic activities. These injuries have to be differentiated from traumatic lesions at the level of the brachial plexus, including the so-called stinger injury. The two major proposed etiologies for these athletic injuries are either cervical nerve root compression and traction or a mild stretch injury to the upper trunk of the brachial plexus. If weakness persists, and either MRI or EMG evidence of a nerve root lesion is identified, one may need to consider cervical laminectomy.
- •
Trauma: athletics injuries.
- •
Inflammatory lesions: pseudoneoplasm.
- •
Extramedullary lesions: hematoma.
Clinical Presentation
It is important to be familiar with the clinical characteristics of radiculopathies at each spinal level, because these lesions may mimic much more common clinical entities, such as mononeuropathies or plexopathies. Most individuals with cervical radiculopathy have cervical or periscapular pain, which often radiates into the arm. With traumatic radiculopathies, this pain may precede the onset of numbness by 4 to 8 weeks, can spontaneously improve, and can then be followed by paresthesia and weakness a few weeks later. C7 or C6 nerve root lesions typically present with numbness affecting digits two or three, in a similar distribution to a median neuropathy. In contrast, C8 radiculopathies cause sensory symptoms involving the fifth digit, mimicking the distribution of the ipsilateral ulnar nerve or medial cord of the brachial plexus. When there is weakness, the triceps muscle is particularly affected in C7 lesions and the pronator teres and flexor carpi radialis in C6 radiculopathies; and the intrinsic hand muscles, flexor pollicis longus, extensor pollicis longus, and extensor indicis proprius are typically weakened by C8 nerve root lesions.
Differential Diagnosis
Refer to Box 12.2 for a list of differential diagnoses, discussed in the following subsections.
- •
Congenital cervical stenosis
- •
Hirayama disease
- •
Motor neuron disease
- •
Neck–tongue syndrome
- •
Grisel syndrome
- •
Neurogenic thoracic outlet syndrome.
Congenital Cervical Stenosis
Although the authors’ experience has not included any typical cervical radiculopathies secondary to either spinal disk or osteophyte-induced foraminal stenosis, we have seen occasional cases of pain in neck, shoulders or arms, or of painless progressive upper extremity weakness, with changes of chronic denervation on EMG and imaging evidence of congenital spinal stenosis, often in association with segmentation anomalies affecting the cervical spine.
Hirayama Disease
This cervical myelopathy causes progressive painless wasting and weakness of the distal upper extremities in adolescence. Most affected children are teenage males, often of western Pacific Rim heritage. Weakness progresses for a few years then arrests spontaneously, for reasons which are poorly understood. Neuropathologic and radiologic findings in this condition suggest forward displacement of the posterior cervical dural sac during neck flexion, causing compression of the cervical cord and ischemic changes in the anterior horn. Because Hirayama disease usually initially affects hand function, it can be confused with ulnar neuropathy, a medial cord brachial plexus lesion, or C8 radiculopathy.
Motor Neuron Disease
The authors have seen only one case of juvenile motor neuron disease, in a teenager with a one-year history of progressive painless weakness of one arm. In this adolescent the median and ulnar compound muscle action potentials were absent, the SNAPs normal, and needle EMG demonstrated diffuse fibrillation potentials with almost no definable motor unit potentials (MUPs). Eighteen months later, when she died, an autopsy demonstrated classic ALS. In a major review of 123 ALS patients there was only one similar case of symptoms of MND commencing before age 20 years.
Grisel Syndrome
Grisel syndrome is an atraumatic atlanto-axial subluxation which may follow inflammatory or infection conditions in the head and neck. Affected children typically present with unrelenting neck or throat pain, followed by torticollis, usually with a preceding history of a flu-like illness, tonsillitis, or pharyngitis. Other neurologic manifestations may include greater occipital nerve paresthesias and development of long-tract signs due to cervical myelopathy. Occasional patients develop cervical radiculopathy. The physical findings include tenderness to spinal percussion and restriction of neck movement leading to a torticollis. Plain X-rays and MRI imaging demonstrate atlanto-axial subluxation. Treatment with antibiotics, anti-inflammatories, immobilization and/or traction is important in order to prevent cord injury.
A similar condition, neck-tongue syndrome, presents with paroxysmal attacks of sharp unilateral neck pain associated with ipsilateral tongue numbness, which may be precipitated by rotation of the neck. Some cases are associated with torticollis, tongue deviation, and dysarthria. Symptoms are presumed related to intermittent compression of the C2 nerve root, and respond in most cases to conservative treatment.
Neurogenic Thoracic Outlet Syndrome
This disorder is caused by a congenital aberrant fibrous band extending from an elongated C7 transverse process to the first thoracic rib, and can come to clinical attention in late adolescence or early adulthood. Patients describe atrophy and weakness of hand muscles, aching in the medial forearm, and occasionally loss of sensation along the ulnar aspect of the hand. The initial consideration is of ulnar neuropathy or C8 radiculopathy. However, close inspection shows predominant weakness and atrophy of the thenar eminence. The pathology stems from progressive extraspinal stretching of the T1 anterior primary ramus over the fibrous band. Nerve conduction studies (NCS) show the classic pattern of severe reduction of the median CMAP to the thenar eminence (abductor pollicis brevis muscle), less reduction of the ulnar compound muscle action potential (CMAP) to the hypothenar eminence (abductor digiti minimi muscle), severe reduction of medial antebrachial cutaneous SNAP and less reduction of the ulnar SNAP to digit 5. Based on this electrical pattern, imaging should be performed to identify the elongated transverse process and band, and surgery is indicated to section the band and release the T1 nerve trunk.
Lumbosacral Radiculopathies
Etiology
On the rare occasions that intervertebral disk herniation occurs in children, it usually occurs in the lumbosacral area. See Box 12.3 for reported causes of pediatric lumbar radiculopathies.
- •
Trauma: sport-related, falls, heavy lifting.
- •
Postoperative: direct trauma, or traction.
- •
Developmental anomalies: vertebral anomalies, synovial cysts, diskal cysts, arachnoid cysts.
- •
Tumors: schwannoma, osteblastoma, osteosarcoma, leukemia, synovial sarcoma, giant cell tumor, aneurysmal bone cyst.
- •
Immunosuppression: chemotherapy, bone marrow transplantation, radiotherapy.
Most cases are in adolescent boys. Trauma, related to sporting activities, lifting, falls, or occupational injuries, accounts for at least one-third to one-half of pediatric lumbosacral radiculopathies. The youngest such case was of a 27-month-old boy who fell from his crib. A significant proportion (13–57%) of adolescents with lumbosacral disc disease has a first-degree relative with a similar history, suggesting a genetic component to this condition.
Scoliosis surgery also predisposes to development of pediatric lumbosacral radiculopathies. One series attributed a 29% incidence of these lesions postoperatively to either surgical trauma or traction in the lumbosacral area. Intraoperative spinal monitoring may aid prevention of this surgical complication.
Developmental anomalies involving intramedullary and extramedullary structures can impinge on the spinal roots, causing pain, weakness and sensory changes.
Nerve root tumors occasionally present with radicular pain radiating into the lower extremities, with or without sensory changes and focal weakness. Reported causes of this syndrome include schwannoma, leukemia, synovial sarcoma, osteoblastoma, osteosarcoma, and aneurysmal bone cysts. Treatment of childhood malignancies with chemotherapy, radiotherapy, and bone marrow transplantation may also be followed by radiculitis.
Clinical Presentation
Most children present with back pain and sciatica. Pain is not invariable; occasional children develop a painless scoliosis. Children aged less than 10 years present with irritability, paravertebral muscle spasm, and leg weakness.
Neurologic deficits are usually relatively subtle and apparent at only one spinal level. A mild degree of focal weakness is seen in 32–60% of adolescents, most often involving the L5 musculature (tibialis anterior, extensor hallucis, and/or extensor digitorum). This is not generally severe enough to cause a foot drop. Well-defined sensory loss may or may not be present. In addition, paravertebral muscle spasm with concomitant limitation of spinal motion, cough-sneeze exacerbation (76%), and/or a positive Lasègue sign are often apparent. Most adolescents have pain on straight-leg raise or back motion. Disturbances of bladder and bowel function, however, are very rare and should prompt consideration of intrinsic cord dysfunction.
Evaluation
Routine spinal radiographs may demonstrate congenital bony and spinal segmentation anomalies, but the significance of these is often uncertain. A combined diagnostic approach including lumbosacral X-rays, lumbosacral spine MRI, CT imaging in certain cases, and—when these studies are nondiagnostic—EMG, has been recommended for diagnosis of lumbar nerve root disease in teenage children.
Differential Diagnosis
Infections, malignancies, and spinal injuries or developmental disorders such as spondylolisthesis should be considered in all children with spinal pain, lumbar nerve root symptoms, and an abnormal gait. As in adults, acute back pain is usually a self-limited musculoskeletal disorder that does not require investigation, and which responds to mobilization exercises and nonsteroidal anti-inflammatory medications. However, there are red flags that should trigger more aggressive evaluation. These include fever; pain that does not improve at night, at rest, or when supine; weight loss; known malignancy; or when symptoms extend beyond several weeks. See Box 12.4 for a list of differential diagnoses.
- •
Infections: diskitis.
- •
Inflammatory conditions: ankylosing spondylitis, sacro-iliac misalignment.
- •
Developmental anomalies: Chiari malformation, tethered cord, cord lipoma.
- •
Mass lesions: angiolipoma, arteriovenous hemangioma, cavernous hemangioma.
- •
Sacral lesions: Ewing’s sarcoma, osteoblastoma, hemangiopericytoma, chordoma.
- •
Pelvic lesions: hematocolpos.
Orthopedic Conditions
Diskitis, or intervertebral disk inflammation, is an uncommon and poorly understood disorder that generally presents in childhood with back pain, limp, and refusal to walk. It can be associated with decreased muscle strength and/or hyporeflexia. Although diskitis is believed to be infectious, positive bacterial cultures are rarely obtained. Imaging studies show narrowing of the intervertebral disk space and/or increased uptake in the area on bone scan, and most authors advocate antibiotic therapy. Disk protrusion into the spinal canal in diskitis may underpin radicular pain and focal weakness in a small proportion of children with this condition.
In older children, especially adolescent boys, ankylosing spondylitis is an important consideration in the differential diagnosis of sciatica. There may be a history of early morning stiffness and “jelling,” associated with diminished chest excursion on examination. Sacroiliac joint misalignment can also cause back pain.
Intraspinal Lesions
Angiolipomas, arteriovenous hemangiomas and cavernous hemangiomas are benign extradural tumors which are occasionally seen around the spine. (See Case Example 12.2 .) Occasionally congenital anomalies, such as Chiari malformations and cord tethering, may present with increasing weakness and foot drop mimicking radiculopathy.
A 10-month-old girl was evaluated for failure to crawl and progressive inability to use her legs since age 6 months. Neurological examination showed hypotonia, weakness, and hyporeflexia in the legs, with relative preservation of motor function in the arms. She was referred for electrodiagnostic studies to exclude spinal muscular atrophy. NCS of the leg and arm were within normal limits, including sensory responses. The needle EMG showed fibrillation potentials in muscles below the knees bilaterally, associated with reduced recruitment of motor unit action potentials with neurogenic morphological changes. Because of sparing of L3–4 muscles and arm muscles, the pattern suggested an active cauda equina syndrome affecting the L5 and S1 roots bilaterally. Subsequently, spine MRI showed an enhancing paraspinal mass extending into the spinal canal from T12 to S1, with severe effacement of the thecal sac. Biopsy showed a poorly differentiated neuroblastoma.
Sacral and Pelvic Lesions
Primary sacral bone tumors or pelvic lesions may present with radicular pain, paresthesiae, and progressive weakness mimicking a lumbosacral radiculopathy. Standard X-rays may be normal; MRI and CT are the best diagnostic studies. (See Case Example 12.3 .)
This 13-year-old boy developed a slowly progressive painless foot drop over a 2-year period, with development of pes cavus and mild calf atrophy. Neurologic examination at the Lahey Clinic demonstrated 3/5 weakness, primarily affecting his left tibialis anterior muscle. He was referred to the EMG laboratory for evaluation of a suspected peroneal neuropathy.
Peroneal and tibial NCS and sural SNAPs were normal. Needle EMG demonstrated findings of both S1 and L5 nerve root involvement including abnormal insertional activity in the lumbar paraspinal muscles. Spinal MRI demonstrated a tethered cord and congenital tumor affecting the L5 and S1 nerve roots.
Comment
The neurophysiologic examination in this case was vital, excluding a (much more common) peroneal neuropathy and indicating a proximal polyradiculopathy, which guided imaging and diagnostic surgical exploration.
Treatment
Conservative management of lumbosacral radiculopathy—bed rest, analgesia and antiinflammatories, and physiotherapy—may relieve pain and weakness, although these symptoms may later recur. Surgery is indicated in children with severe pain refractory to conservative treatment, or with progressive neurological deficits. Surgical approaches include percutaneous endoscopic diskectomy or open diskectomy, with or without laminotomy or laminectomy. Outcomes of surgery are generally very good, but reoperation is needed in about 10 to 25 percent of cases.
Plexopathies
Brachial Plexopathies
Pediatric brachial plexus lesions include birth-related lesions and other acquired disorders sustained from infancy through late adolescence. Neonatal brachial plexus palsy (NBP) complicates a very small proportion of births, but continues to be a challenge in the setting of complicated labor and childbirth. Most brachial plexopathies in older children result from trauma.
Brachial Plexus Anatomy
There are five major components of the brachial plexus; the roots, trunks, divisions, cords, and terminal nerves ( Figure 12.5 ). Cervical roots C5–8 and the first thoracic root provide the primary source for the three trunks (upper, middle, and lower); two divisions (consisting of six elements in all); three cords (lateral, posterior, and medial); and the various terminal branches. In the arm and hand, these include the axillary, musculocutaneous, radial, median, and ulnar nerves.

The long thoracic and dorsal scapular nerves are early (supraclavicular) derivatives of the brachial plexus, which form from the anterior primary rami proximal to the brachial plexus. The dorsal scapular nerve arises from the C5 anterior primary ramus near the intervertebral foramen, and innervates the rhomboid muscle. Denervation of the rhomboids is generally a poor prognostic sign in a child with a neonatal brachial plexopathy, as it suggests a very proximal lesion, possibly including C5 root avulsion. The long thoracic nerve originates from the C5, C6, and C7 anterior primary rami, and innervates serratus anterior.
Most peripheral nerves supplying the upper extremity originate from the infraclavicular portion of the brachial plexus. The three trunks each divide into an anterior and posterior division at the level of the clavicle. The three posterior divisions form the posterior cord, the anterior divisions of the upper and middle trunks form the lateral cord, and the anterior division of the lower trunk continues distally as the medial cord. The three major cords each provide the primary source for the individual nerves of the arm, with the exception of the conjoint origin of the median nerve from both the lateral and medial cords.
The lateral cord (C5–6) gives off the lateral pectoral nerve to the pectoralis major muscle, and then gives rise to the musculocutaneous nerve, innervating the biceps brachii and the brachialis muscles, and contributes to the median nerve, innervating pronator teres and the forearm flexor muscles.
Nerves formed from the posterior cord (C6, 7, 8) include the subscapular nerve (to subscapularis), thoracodorsal (to latissimus dorsi), lower subscapular (to teres major), axillary (to deltoid and teres minor), and the radial nerve (supplying the triceps, anconeus, and all wrist and finger extensors).
The medial cord (C8–T1) gives rise to the medial pectoral, medial brachial, and medial antebrachial cutaneous nerves, and contributes to both the median nerve (innervating flexor pollicis longus and most thenar intrinsic hand muscles) and the ulnar nerve (which innervates the hypothenar hand intrinsics and adductor pollicis).
At or very near its origin, the anterior primary ramus of each nerve root receives a contribution from the corresponding sympathetic trunk ganglion, the gray ramus communicans. In addition, the first thoracic anterior primary ramus contributes preganglionic sympathetic fibers to the inferior cervical (stellate) ganglion via a white ramus communicans ( Figure 12.6A ). A lesion affecting these fibers produces a Horner syndrome (unilateral miosis, ptosis, enophthalmos, and facial anhidrosis) ( Figure 12.6C ).

Diagnostic Procedures
Imaging
Magnetic resonance imaging (MRI) is one of two primary diagnostic modalities for evaluation of the child with a suspected brachial plexus lesion. This evaluation must differentiate between (preganglionic) nerve root lesions, and (postganglionic) brachial plexus lesions. Avulsion of ventral or dorsal nerve rootlets from the spinal cord results in preganglionic nerve root injury with no potential for spontaneous nerve regeneration ( Figure 12.6 ). The diagnosis of nerve root avulsion is typified by the finding of a pseudo-meningocele, documented by demonstration of deformity of the subarachnoid space, most often at the C7 level. MRI is noninvasive, gives excellent depiction of pseudo-meningoceles, and also assesses the spinal cord and soft tissue surrounding the brachial plexus ( Figure 12.7 ). Although there is a strong association between nerve root avulsion and development of pseudo-meningoceles, this association is not invariable: a significant percentage of avulsions are not associated with pseudo-meningoceles, and vice versa. Imaging studies have variable sensitivity in the diagnosis of root avulsion. CT myelography provides higher spatial resolution and may be necessary in some situations to confirm the presence of a pseudo-meningocele. CT myelography can be especially useful in demonstrating absent rootlets within the pseudomeningocele, a better predictor of nerve root avulsion and absent intraoperative evoked responses. In older children, combined spinal and brachial plexus MRI is often useful in differentiating brachial plexus and nerve root lesions.

With the continued advances in instrumentation and improvement in resolution, MR imaging has supplanted CT myelography for investigation and preoperative evaluation of NBP.
Electrodiagnosis
When evaluating the infant or child with a possible brachial plexus lesion there are three specific questions to address. These include: (1) establishment of the level and extent of plexus involvement; (2) accurate identification of the presence of a preganglionic lesion and/or root avulsion; and (3) defining the nature of the lesion, in terms of its underlying pathophysiological response to injury as described by Seddon and Sunderland (i.e. neurapraxia versus axonotmesis/neurotmesis). These results provide information important in identifying patients who might benefit from surgery.
The electrodiagnostic evaluation of brachial plexopathies typically includes median and ulnar sensory and motor nerve conduction studies (NCS), radial sensory NCS, and musculocutaneous motor NCS. The lateral anterior brachial cutaneous nerve sensory response is informative but technically difficult to obtain in infants. With all these studies, comparison with the response on the opposite side may provide useful information. CMAP and SNAP amplitudes provide some indication of the degree of axonal damage. CMAP and SNAP amplitudes drop progressively after axonotmesis. In adults, loss of CMAP amplitude is apparent by day 3 and maximal at day 5–8. SNAP amplitude loss begins at day 5 and is maximal by day 9–11. Data for infants and young children have not been published.
Needle electromyographic examination (NEE) provides additional information. The NEE can assess the presence and severity of nerve injury in muscles from which CMAPs cannot be recorded, and can identify more subtle degrees of motor axon loss than is apparent from determination of CMAP amplitude. The precise timing of the onset of positive waves and fibrillation potentials after axonal injury in the older child is similar to that of the adult (10–14 days). There are very few studies specifically defining the temporal profile of EMG signs of axonal damage in the neonate. Because of the very short distances from the site of injury to the muscle, as well as the immature, poorly myelinated peripheral nervous system, the timing of these changes is likely to be very different from that in adults. Several case reports have suggested that EMG findings suggestive of acute motor axonal injury in neonatal brachial plexopathy can be seen as early as four days after delivery. In other cases nerve injury prior to delivery has also been associated with very early EMG abnormalities.
Paraspinal muscle denervation is the primary criterion for differentiation between a nerve root and plexus lesion. Because of the baby’s inherent inability to relax, however, needle EMG of the paraspinal muscles is usually not technically satisfactory in infants. Preservation of SNAPs in the absence of clinical sensory function is a classic finding suggesting avulsion of the corresponding preganglionic nerve root(s), but in infants and toddlers identification of clinical sensory loss can be very difficult. Additionally, it is not uncommon for neonatal plexus lesions to have combined preganglionic and postganglionic components. An avulsion, with its inherently poor prognosis, may be present despite the absence of SNAPs in the corresponding segment ( Figure 12.6 ). These factors illustrate the technical limitations of electrodiagnosis. In addition, precise localization of the root(s) affected is limited by the overlapping segmental innervation of many muscles in the arm. To achieve accurate localization complementary testing, such as magnetic resonance imaging, is required.
Although somatosensory evoked potentials (SSEPs) were initially thought to be valuable in the evaluation of neonatal brachial plexopathy (NBP), particularly for the assessment of nerve root continuity, they have not proven to add more to diagnosis than EMG. SSEPs may be helpful in the intraoperative assessment of BP lesions, but their clinical utility is limited by the fact that they assess only dorsal (sensory) root integrity. Intraoperative transcranial electrical motor evoked potentials have been used to evaluate the functional status of anterior spinal roots and spinal nerves during brachial plexus repair in adults, but their value in pediatric practice is unclear.
Neonatal Brachial Plexus Lesions
Historical Aspects
Robotti et al. provided an excellent historical review of this subject. They attribute the first citation of NBP to Smellie in 1768. Duchenne de Boulogne described the typical unilateral NBP in his 1872 treatise on localized electrical stimulation of four neonates, and attributed the injury to traction on the arm at the time of birth. In 1874, Erb reported electrical stimulation of the brachial plexus in NBP, and acknowledged Duchenne’s prior descriptions. Thus, the classic upper brachial plexus palsy, described first by Duchenne, bears the name of “Erb’s palsy” although some still refer to this entity as Erb-Duchenne palsy. Klumpke, in 1885, described the paralysis of the lower portion of the BP with associated Horner sign secondary to concomitant sympathetic fiber involvement. When the entire plexus is involved, this is referred to as an Erb-Klumpke paralysis ( Figure 12.5 ).
Incidence and Risk Factors
Sever’s seminal 1925 study of 1100 newborns with brachial plexopathies provided the first detailed description of neonatal brachial plexus palsy. A decreasing incidence of NBP was reported between 1938 (1.56 per 1000 live births) and 1963 (0.38 per 1000 live births) in New York, possibly reflecting improved obstetric care. The incidence of NBP incidence in industrialized countries now varies from 0.5 to 2 per 1000 live births.
The greatest risk factor for NBP is macrosomia. Brachial plexopathy is almost three times more common in newborns weighing >4500 g. Other major risk factors include gestational diabetes, shoulder dystocia, and delivery by vacuum or forceps extraction ( Box 12.5 ). (See Case Example 12.4 .) Children with a combination of these risk factors have up to a 52–76-fold increase in risk of NBP in comparison to the baseline population rate. Less significant risk factors include preeclampsia, hypertension, multiparity, duration of labor, breech presentation, and previous pregnancy with NBP. Parents may not be able to provide a full account of the delivery details, but other relevant postnatal information, including respiratory difficulties, evidence of fracture (particularly clavicular), Horner syndrome, and the extent of the infant’s paralysis during the first days of life, contribute to an objective assessment of risk and severity of NBP.
Major
- •
Macrosomia (birth weight >4500 gm)
- •
Gestational diabetes
- •
Instrumented vaginal delivery
- •
Shoulder dystocia.
Minor
- •
Preeclampsia
- •
Hypertension
- •
Multiparity
- •
Duration of labor
- •
Breech presentation
- •
Previous pregnancy with NBP.
A 4300-gram baby girl was born to a gravida V, para III mother via a vaginal delivery complicated by shoulder dystocia. The mother’s previous babies weighed over 4000 grams at birth. One had a transient Erb’s palsy and the other cerebral palsy. At birth, the infant had a flaccid left arm, Horner syndrome, and absent muscle stretch reflexes, and was diagnosed with Erb-Klumpke palsy (Group IV).
An EMG was performed at 5 days of age. No CMAPs or SNAPs could be elicited from the left median and ulnar nerves. NEE demonstrated active denervation with fibrillation potentials or positive waves in the deltoid, biceps, triceps, extensor carpi radialis, and flexor carpi radialis. There were no voluntary MUPs in any of these muscles.
Four years later the child had significant shoulder girdle improvement but impaired (4/5) finger grip and finger extension (2/5).
Comment
There were typical predisposing factors for NBP, including birth weight over 4000 grams, shoulder dystocia, and a sibling with a similar history. Her course was one of gradual improvement with significant residual weakness. The question of a possible intrauterine onset of nerve injury remains unresolved, as does nerve root avulsion as an explanation for the early-onset fibrillation potentials, as no MRI was performed to confirm that diagnosis.
Prenatal diagnosis of macrosomia, and prediction of the risk of shoulder dystocia and NBP, is difficult and imprecise. Bryant et al. evaluated a policy of elective caesarean delivery for macrosomic infants. In mothers without diabetes, they found that, at an estimated fetal weight of 4500 grams, 155–588 caesarean deliveries were required to prevent a single case of permanent injury. Although McFarland et al. demonstrated a 52-fold increased risk of NBP in macrosomic newborns of diabetic mothers, 92% of these babies were delivered vaginally without complications. Mathematic modeling of forces associated with shoulder dystocia demonstrates that the pressures generated from endogenous forces (uterine and maternal expulsive efforts) are 4 to 9 times greater than exogenous forces (applied traction to the fetal head).
NBP rarely occurs in the setting of caesarean section. When it does, consideration of other mechanisms for limb paralysis is appropriate. Intrauterine causes of NBP include fetal positioning and amniotic bands.
Classification
Neonatal brachial plexopathies are best classified into four anatomic groups on the basis of the neurological examination at age 2 to 3 weeks ( Table 12.1 ). Paralysis of the upper roots (group I, Erb’s palsy) is by far (81–98%) the most common form of neonatal brachial plexopathy. In contrast, Klumpke’s palsy (pure C8–T1 with Horner syndrome) is seen in only 0.6% of all cases of NBP.
Level | Clinical Deficit | |
---|---|---|
Group I | C5–6 | Paralysis of shoulder and biceps |
Group II | C5–7 | Paralysis of shoulder, biceps and forearm extensors |
Group III | C5–T1 | Complete paralysis of limb |
Group IV | C5–T1 | Complete paralysis of limb with Horner syndrome |
An infant with an Erb’s palsy (proximal upper trunk and lateral cord lesion) typically lies with his/her affected arm limp at the side with the shoulder adducted and internally rotated, elbow extended, forearm pronated, and fingers and wrists flexed—a posture commonly referred to as “waiter’s tip” ( Figure 12.6B ). The shoulder adduction results from paralysis of the deltoid and supraspinatus muscles. There may also be scapular winging due to serratus anterior muscle weakness from concomitant long thoracic nerve injury, but in newborns this is often difficult to recognize. Internal rotation of the shoulder occurs due to the unopposed contraction of the pectoralis and latissimus dorsi and the unopposed pull of subscapularis, in the setting of teres minor and infraspinatus weakness. Elbow extension is due to both the effects of gravity and weakness of the elbow flexors [biceps, brachialis, and brachioradialis (C5/6)]. The pronated forearm is due to paralysis of the supinator and biceps muscles (C5/6). The flexed wrist and fingers result from weakness of the wrist extensors (C7).
Horner syndrome (ptosis, miosis, and decreased facial sweating) can accompany involvement of the T1 (and sometimes C8) nerve roots, accompanied by severe intrinsic hand weakness typical of Klumpke-type NBP ( Figure 12.6C ). Involvement of the long thoracic, dorsal scapular, and C8–T1 sympathetic nerves suggests a very proximal injury, such as a lesion at the nerve root entry zone from complete avulsion of the preganglionic spinal nerves. Children with this constellation of findings have a poor prognosis for recovery, in contrast to those with postganglionic lesions, in which nerve regeneration is more likely.
Management of Neonatal Brachial Plexopathies
Nonsurgical
Affected infants should be followed at a clinic with expertise in peripheral nerve injuries, and should be assessed regularly over a period of months in order to assess the extent of recovery, if any. A reliable and reproducible method of quantifying motor function is probably the most challenging aspect of assessing the newborn with a brachial plexopathy. The MRC muscle grading system is insufficient for evaluation of infants. Several groups have established their own grading scales. One scale ranges from M-0 (no contraction) to M-3 (complete movement against the weight of the corresponding segment of the extremity). Mallett proposed an 8-item scale designed to capture subtle but significant changes in movement of the arm ( Figure 12.8 ). This system focuses on joint movement, in contrast to individual muscle testing, and is helpful in both the pre- and postoperative settings, providing a practical means of following infants longitudinally. Alternatively the standardized clinical examination defined by the Hospital for Sick Children, Toronto, evaluates extension of the elbow, wrist, fingers and thumb, and flexion of the elbow. A positive score on this clinical exam is associated with a 95% confidence for prognosis of recovery by one year of age ( Table 12.2 ).

Motion | Score | Muscle Grade Score |
---|---|---|
Gravity eliminated | ||
No contraction | 0 | 0 |
Contraction (no motion) | 0.3 | 1 |
Motion<{1/2} range | 0.3 | 2 |
Motion>{1/2} range | 0.6 | 3 |
Full motion | 0.6 | 4 |
Against gravity | ||
Motion<{1/2} range | 0.6 | 5 |
Motion>{1/2} range | 1.3 | 6 |
Full motion | 2 | 7 |
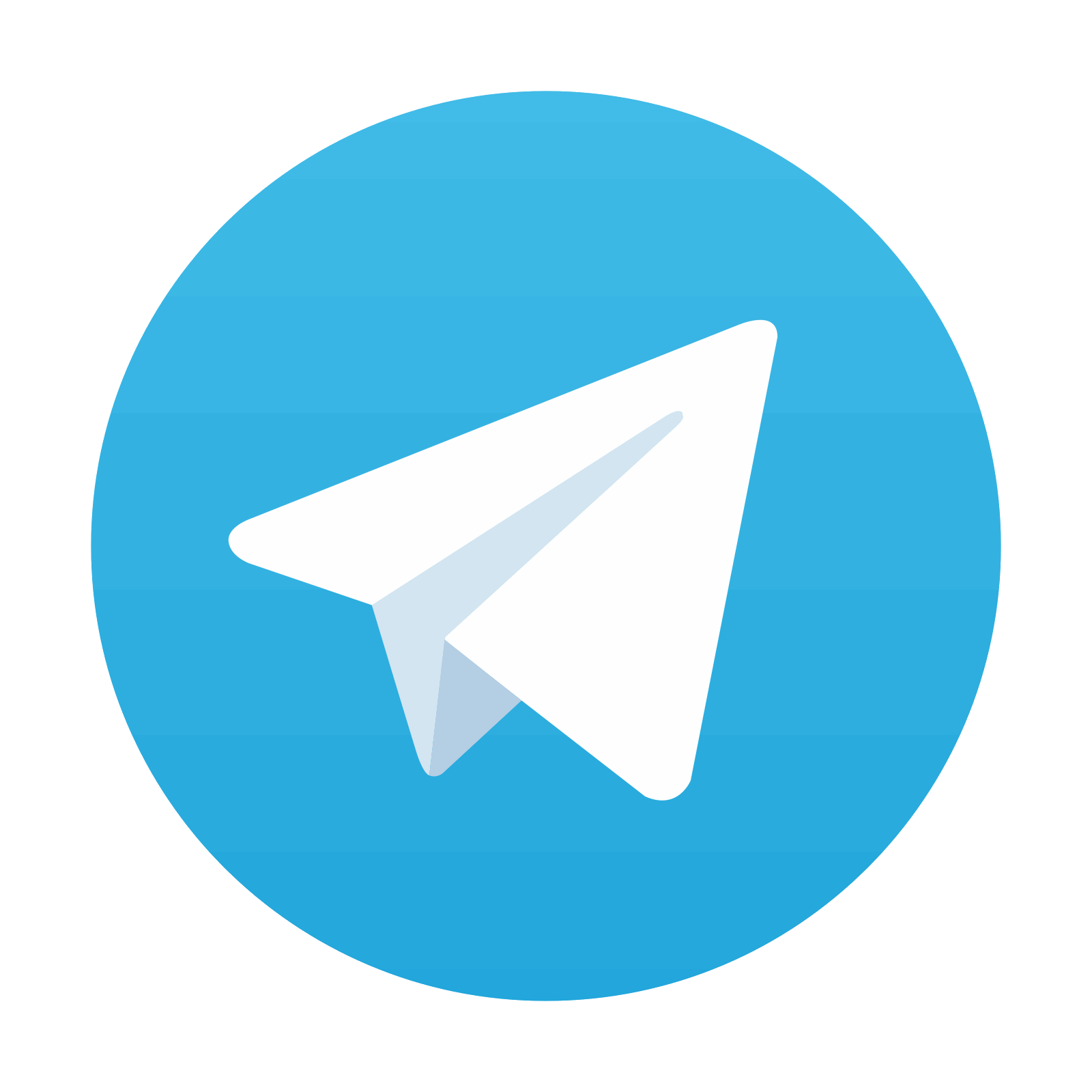
Stay updated, free articles. Join our Telegram channel

Full access? Get Clinical Tree
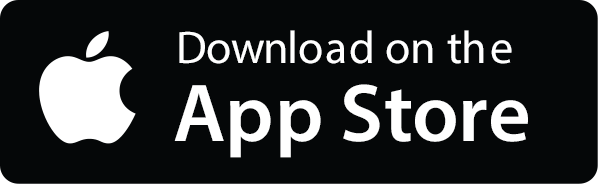
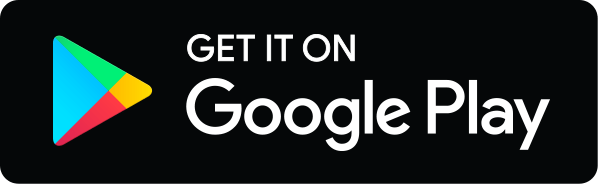
