Resection for Uncontrolled Epilepsy in the Setting of Focal Lesions on MRI: Tumor, Vascular Malformation, Trauma, and Infarction
Dennis D. Spencer
Alexandre C. Carpentier
Advances in neuroimaging techniques, particularly high-resolution magnetic resonance imaging (MRI), have proved invaluable in identifying structural brain lesions in patients with epilepsy. Identification of focal lesions is particularly important when patients with medically refractory partial seizures are considered for epilepsy surgery, because the best outcomes after surgery are usually seen when focal MRI lesions can be identified and are concordant with electrographic findings. The assumption that all such focal lesions invariably predict the site of seizure origin may not be correct, however. Because the electroencephalogram (EEG) is the only readily available clinical method of assessing epileptogenic function, the identification of structural lesions in epilepsy often requires correlation with electrographic studies.
Guidelines for neuroimaging evaluation of patients with uncontrolled epilepsy (1) suggest obtaining T1, T2, three-dimensional volume acquisition, gadolinium contrast enhancement, fluid-attenuated inversion recovery (FLAIR), hippocampal volumetry, and fast spin-echo inversion recovery sequences and computed tomography (CT) for calcified lesions. Ictal single-photon-emission computed tomography (SPECT) and interictal [18F]fluoro-2-deoxyglucose ([18F]FDG) positron emission tomography (PET) also appear to be sensitive to epileptogenic regions. Using three-dimensional postprocessing software for coregistration of images from different modalities with those obtained on MRI can provide more precise information regarding localization.
Beside developmental abnormalities and mesial temporal sclerosis (discussed in another chapter), adult epileptogenic lesions can be distinguished by their histology and encompass the following:
Neoplasms (oligodendroglioma, oligoastrocytoma, astrocytoma, epidermoid cyst, astroblastoma, dysembryoplastic neuroepithelial tumor [DNET], ganglioglioma, xanthoastrocytoma)
Vascular abnormalities (“angiographically visible” or “occult” arteriovenous malformations [AVMs], cavernous angiomas)
Acquired gliosis and encephalomalacia: posttraumatic gliosis and hemosiderin deposition, inflammatory diseases, and ischemic lesions
Different lesions leading to intractable epilepsy have individual histologic, physiopathologic, and EEG properties
that may be important to know about when a surgical treatment is planned.
that may be important to know about when a surgical treatment is planned.
When a lesion is proved to be the epileptogenic region by concordant data from scalp EEG, MRI, neuropsychological findings, and, if needed, PET and SPECT, surgical resection is often proposed. The volume of the resection, however, is still controversial. Should interictal spikes seen on electrocorticography direct the extent of the resection? Should the surrounding marginal cortex be excised along with the lesion? Should visual evidence of abnormal tissue be the only criterion for focus resection, or should preoperative imaging abnormalities guide the resection? If MRI is concordant with standard preoperative evaluations, can surgery be performed directly, or is there a need for a cortical mapping to avoid appreciable functional loss? Should resection be accompanied by multiple subpial transections in functional areas?
Surgical management is challenging, because it often requires separating resectable epileptogenic regions from cortex with appreciable function. The location of the functional cortex in relation to epileptogenic activity can interfere with a complete resection. However, many patients show worthwhile improvement or become seizure free after this “functionally limited” surgery.
Even when MRI studies document structural brain lesions, these are not invariably the sites of seizure origin. In fact, some patients’ ictal onsets seen on scalp EEG recordings arise from a different location from the focal structural lesion seen on MRI. Epilepsy is, after all, fundamentally a problem of abnormal physiology. When noninvasive evaluations are discordant, intracranial monitoring is required. The invasive evaluation may or may not correlate the lesion with the epileptic focus. If not, the study may reveal dual pathology (2,3).
This chapter will describe the evaluation of adult epileptogenic lesions visible on MRI (except developmental abnormalities and mesial temporal sclerosis) and will provide a decision tree for invasive monitoring.
NEW MAGNETIC RESONANCE IMAGING AND FUNCTIONAL IMAGING TECHNIQUES FOR LESIONAL EPILEPSY
In patients undergoing presurgical evaluation, neuroimaging should provide data on the putative epileptogenic region, especially delineation of structural and functional abnormalities, prediction of the nature of the structural pathology, and detection of abnormalities distant from the epileptogenic zone. Emerging goals include functional magnetic resonance imaging (fMRI) identification of such brain regions as the primary sensorimotor, vision, and language and memory areas and the relation of these to the epileptogenic region.
In 1998, the Commission on Neuroimaging of the International League Against Epilepsy provided guidelines for neuroimaging evaluation of patients with uncontrolled epilepsy considered for surgery (1). However, minimal requirements for the number and type of tests have not yet been established.
CT scanning can detect certain structural lesions with calcification (e.g., cysticercosis); however, MRI has largely replaced its use. Even when a CT scan reveals an epileptogenic lesion, MRI often adds new and important data.
MRI examination is integral to presurgical planning. T1- and T2-weighted images, with thin contiguous slices and no interslice gap, should be obtained. Coronal and axial slices along with a three-dimensional volume acquisition constitute the standard protocol in most epilepsy centers. In some instances, gadolinium contrast enhancement may provide useful additional information. Other sequences such as FLAIR and fast spin-echo inversion recovery may have a role in selected cases (e.g., if standard imaging is normal or to clarify the significance of a possible focal abnormality). A careful qualitative and volumetric quantitative side-to-side ratios evaluation of the hippocampus corrected for intracranial volume may show evidence of dual pathology and/or bilateral disease.
In addition to static anatomic acquisitions, functional imaging has a crucial role in presurgical evaluations. Ictal and interictal SPECT, PET, fMRI, magnetic resonance spectroscopy (MRS), and diffusion MRI for fiber tracking are techniques that can help to define abnormal function, which may add correlative data in defining the epileptic region.
ELECTROENCEPHALOGRAPHIC FEATURES OF SYMPTOMATIC EPILEPSIES
A potential epileptogenic lesion may be identified far from where scalp ictal discharges seem to arise. False localization can be caused by propagation of ictal discharges, for example, from a parietal focus through the limbic system (4). Spread of epileptic activity from neocortical to medial temporal structures was proposed by Ajmone-Marsan and Gumnit (5) as being responsible for generation of seizures. Several subsequent observations were made regarding spread of seizures from temporal neocortex to the hippocampus (6,7). Walczak and colleagues (8) found that the majority of seizures originating in the temporal neocortex spread to mesiobasal temporal regions before behavioral expression.
For example, somatosensory auras followed by unilateral clonic and tonic motor seizures are well-recognized features of parietal lobe foci, especially of the perirolandic area (9). Many clinical findings have been linked with parietal lobe seizures, including epigastric sensations, vestibular and visual hallucinations, automatisms, and arrest reactions (9, 10, 11). These may be produced by the spread of discharges to surrounding areas. Ictal discharges can originate in the
posterior cingulate gyrus and precuneus of the medial parietal lobe, but it is only when the ictal discharges propagate into the temporal lobes that symptoms of complex partial seizures of temporal lobe type occur (11).
posterior cingulate gyrus and precuneus of the medial parietal lobe, but it is only when the ictal discharges propagate into the temporal lobes that symptoms of complex partial seizures of temporal lobe type occur (11).
The cingulum (which shares fibers with the limbic system) and the corpus callosum participate in propagation of ictal discharges to both temporal lobes and can offer a complex electrical spread pattern (4). This may complicate identification of a focal epileptogenic zone in the cingulum in cases with unremarkable MRI findings.
CORRELATION OF MAGNETIC RESONANCE IMAGING CHARACTERISTICS WITH COMMONLY IDENTIFIED PATHOLOGIES
Eriksson and coworkers (12) reported that a blinded review of MRI scans in their surgical series showed correlation with histopathologic findings in all cases of vascular malformation, in more than 75% cases of tumor or hippocampal sclerosis, and in 28.6% of major cortical malformations. A detailed description of the epileptogenic lesions most often identified on MRI is provided in the following sections.
Neoplasms
Epilepsy is a common presentation in patients with cerebral tumors. Low-grade cerebral neoplasms, including astrocytoma, mixed glioma, oligodendroglioma, and DNET, constitute the pathologic substrates in some 10% to 30% of patients with chronic intractable partial epilepsy (13,14). Epilepsy is the first symptom in 50% of astroglial neoplasms at presentation, and ganglioglioma may be the most common cause of tumor-related chronic epilepsy, with the highest incidence of seizures (88%) (15, 16, 17).
Oligodendroglioma
The morphologic spectrum of oligodendrogliomas includes tumors that are traditionally misinterpreted as “diffuse fibrillary astrocytomas.” These consist of isolated neoplastic oligodendrocytes that are entrapped in a background composed of axons and fibrillary reactive gliosis. In some cases, a genetic deletion is present in the 1p19q locus. A series of 153 “pure” supratentorial oligodendrogliomas composed of “classic” or pseudo-“diffuse fibrillary oligodendrogliomas” showed that two-thirds of the tumors were exclusively isolated tumor cells (type III) and that only one-third exhibited both isolated tumor cells and solid tumor tissue components (type II). The type II tumor destroys brain parenchyma and forms new micro-blood vessels, whereas isolated tumor cells do not destroy the parenchyma and are not associated with microangiogenesis. Clinically, a neurologic deficit occurs in about 57% of type II but in only 8% of type III oligodendrogliomas. On MRI, contrast enhancement is observed in type II oligodendrogliomas (64%) but is usually not seen in type III. The biologic behavior of these tumors seems to be closely related to the pattern of tumor growth. Emergence of microangiogenesis within a tumor that at first grows slowly with a type III pattern signals more aggressive behavior (18).
MRI typically reveals oligodendrogliomas to be hyperintense on spin density and T2-weighted images. Their signal is often heterogeneous owing to small cysts, calcification, or prior hemorrhage. Calcification is especially common and may be seen best on CT.
Statistics show anaplastic transformation in 50% of patients 10 years after diagnosis. Anaplastic transformation may be suspected on the basis of PET studies, because anaplastic oligodendrogliomas show a higher methionine and [18F]FDG fixation than low-grade oligodendrogliomas. Radiotherapy and chemotherapy with gadolinium enhancement are usually indicated for recurrences (19). Survival for patients with anaplastic oligodendroglioma is about 3 years.
Low-Grade Astrocytoma
Low-grade astrocytomas associated with chronic seizures constitute a distinct clinicopathologic group of tumors that arise in young hosts (20 to 40 years old). These low-grade gliomas usually have an indolent course. An important factor in achieving long-term seizure control is complete removal of the lesion to tumor-free margins. In addition, complete resection of the tumor may be the most effective treatment for control of low-grade glioma growth. Margins may be limited by functional cortex, leading to partial tumor excision. For low-grade gliomas in the temporal lobe, additional medial temporal resection may be required. This is most often predicted preoperatively by MRI that shows the lesion infiltrating the hippocampus or hippocampal atrophy. Decisions about extralesional hippocampal resection should be based on evaluation of this presumed dual pathology using several variables in addition to hippocampal atrophy, including seizure history, video-EEG, semiology, proximity of the tumor to the hippocampus, and the neuropsychological profile of the patient, especially with regard to memory function (20).
On MRI, low-grade astrocytomas usually appear homogeneous, although some tumors have cystic components. The signal is hyperintense on double-echo sequence and hypointense on T1-weighted images. Calcification is seen on CT in the minority of low-grade astrocytomas, compared with the strong majority of oligodendrogliomas; however, because astrocytomas are the most common overall, any tumor with calcification is most likely to be of this type.
There appears to be a significant decrease in the risk of tumor recurrence in patients who undergo aggressive resection (100% 10-year survival) compared with patients who have anything less than gross-total resection (21). Gender, type of symptoms, length of preoperative symptoms, and timing of radiation therapy (immediate postoperative versus delayed radiotherapy) are not known to be significant
in determining outcome, length of survival, recurrence, and/or incidence of transition to more malignant tumors. Within the population of patients with low-grade astrocytomas, those with chronic epilepsy usually have the best prognosis. Tumors associated with chronic epilepsy are much less likely to become more malignant over time (21). Irradiation can be used to reduce intractable seizure frequency in some patients with unresectable (biopsy-proven) low-grade astrocytoma (19). Focal radiation treatment may reduce seizures by more than 75% in 80% of cases, and the MRI often shows a tumor response to radiation that correlates with reduced seizure frequency (19). In patients who became seizure free, seizure recurrence is usually associated with tumor progression (14).
in determining outcome, length of survival, recurrence, and/or incidence of transition to more malignant tumors. Within the population of patients with low-grade astrocytomas, those with chronic epilepsy usually have the best prognosis. Tumors associated with chronic epilepsy are much less likely to become more malignant over time (21). Irradiation can be used to reduce intractable seizure frequency in some patients with unresectable (biopsy-proven) low-grade astrocytoma (19). Focal radiation treatment may reduce seizures by more than 75% in 80% of cases, and the MRI often shows a tumor response to radiation that correlates with reduced seizure frequency (19). In patients who became seizure free, seizure recurrence is usually associated with tumor progression (14).
Ganglioglioma
Ganglioglioma may be the most common cause of tumor-related chronic epilepsy (22,23). The current World Health Organization (WHO) classification defines ganglioglioma as a neoplasm composed of neoplastic neural (ganglion cells) and glial elements (24). Ganglioglioma accounts for 0.4% to 9% (17) of primary brain tumors diagnosed at different institutions. It has been described in many parts of the central nervous system but is most commonly found in the temporal lobe (25,26).
The tumor has been localized by MRI or CT scans in the temporal (70%), frontal (10%), parietal (8%), and occipital (3%) lobes. MRI is able to detect the tumor with a very high degree of sensitivity, but findings are infrequently specific for ganglioglioma. On MRI, a wide variety of signals may be found (23), such as gadolinium enhancement in 60%, mass effect in 45%, cystic change in 30%, and cerebral edema in 5%. CT scan, suggested by Tampieri and colleagues (23), shows abnormality in 70%, with calcification in 35% of these, but is reported as normal in the other 30%.
Histologically, the tumors are composed of large polymorphic ganglion cells (pyramidal-like neurons), with vesicular nuclei and prominent nucleoli admixed with a neoplastic glial element, most often astrocytic. Mild perivascular chronic inflammation and vascular endothelial proliferation are usually found. Cortical dysplasia—generally adjacent to but separate from the tumor—is present in 40% of the cases described in the literature (22).
Good seizure control is seen in patients with resected ganglioglioma, despite years of medically resistant seizures: 80% of patients are seizure free (Engel class I) at 6 months, 72% at 1 year, and 63% at 2 years (22). Good outcome may be achieved, despite scalp EEG findings that may conflict with tumor location, and is more likely when surgery is performed relatively soon after epilepsy onset. MRI features such as presence or absence of gadolinium enhancement, mass effect, and cystic change had no measurable influence on the seizure outcome after tumor resection. Malignant degeneration is a rare consequence of these tumors, but the glial component can become anaplastic. No strong statistical data are available to indicate the frequency of such transformations.
Dysembryoplastic Neuroepithelial Tumor
DNETs are a group of supratentorial cortically based lesions that superficially resemble mixed oligoastrocytomas, oligodendrogliomas, and astrocytomas. Clinically, these tumors are associated with complex partial seizures beginning before the age of 20 years, with no neurologic deficit and no stigmata of phakomatosis (27). In the revised WHO classification, DNETs have been incorporated into the category of neuronal and mixed neuronoglial tumors. This classification describes a histologic variant characterized by the following criteria: cortical location, multinodular architecture (the nodule consisting of astrocytoma-like variants, oligodendrogliomas, or oligoastrocytomas), and foci of dysplastic cortical disorganization, showing a columnar structure perpendicular to the cortical surface. Daumas-Duport (28) described a glioneuronal element that was demonstrated to be specific and sufficient for diagnosing DNETs. Even if some of these tumors show a high MIB-1 labeling index, the spectrum of DNETs includes a simple form with a unique glioneuronal element. DNET is a surgically curable tumor of young patients with intractable partial seizures (29).
Daumas-Duport and colleagues (29) reported a series of 39 cases of neuroepithelial tumors associated with medically intractable complex partial seizures. The age at onset of symptoms ranged from 1 to 19 years (mean, 9 years). In addition to the chronic nature of the seizures (range, 2 to 18 years; mean, 9 years), one third of the patients showed radiologic features such as focal cranial deformity, indicating that the tumors had an early onset and were long-standing. In most cases, CT scans showed a “pseudo-cystic,” welldemarcated, low-density appearance, associated in some cases with focal contrast enhancement (18%) or calcified hyperdensity (23%). MRI signals are hypo and hyper on T1 and T2 sequences, respectively. No edema, but possible gadolinium enhancement, can be seen. In a few cases, DNET was associated with neurofibromatosis type 1. The tumor involved primarily the temporal lobe (62%) but also occurred in the frontal lobe (31%). Although tumor removal was considered incomplete or subtotal in 17 patients (44%), long-term follow up (range, 1 to 18 years; mean, 9 years) showed neither clinical nor radiologic evidence of progression in any patient. Comparison of the survival data of the 13 subjects who had undergone postoperative radiotherapy with the 26 who had not indicated that radiation therapy was of no obvious benefit. The identification of DNET has therapeutic and prognostic implications because aggressive therapy can be avoided, thus sparing these young patients the deleterious long-term effects of radiotherapy or chemotherapy.
Pleomorphic Xanthoastrocytoma
Pleomorphic xanthoastrocytoma is a relatively rare brain tumor of adolescents and young adults, characterized by its superficial location, sometimes associated with a cyst, and by frequent involvement of the meninges. Temporal lobe localization helps explain why epilepsy predominates as a
presenting symptom. Histology shows intracellular lipid inclusions, hypervascularization, and in all cases an arachnoidal component. The tumor is slow-growing, despite features of histologic atypia that place it in WHO grade II or III on the basis of elevated mitotic counts and the presence of punctiform necrosis. Immunostaining with the proliferation marker MIB-1 is present in only 2% of cells in the grade II group, whereas 5% show labeling in grade III. All pleomorphic xanthoastrocytomas express some amount of glial fibrillary acidic protein and are shown to elaborate a characteristic pericellular reticulin network. Surgical treatment is considered to offer the best survival outcome. Tumor recurrence with confirmed anaplasia still occurs in about 20% of cases at a mean postoperative follow up of 5 years, and survival for patients with recurring anaplastic xanthoastrocytomas is about 2 years.
presenting symptom. Histology shows intracellular lipid inclusions, hypervascularization, and in all cases an arachnoidal component. The tumor is slow-growing, despite features of histologic atypia that place it in WHO grade II or III on the basis of elevated mitotic counts and the presence of punctiform necrosis. Immunostaining with the proliferation marker MIB-1 is present in only 2% of cells in the grade II group, whereas 5% show labeling in grade III. All pleomorphic xanthoastrocytomas express some amount of glial fibrillary acidic protein and are shown to elaborate a characteristic pericellular reticulin network. Surgical treatment is considered to offer the best survival outcome. Tumor recurrence with confirmed anaplasia still occurs in about 20% of cases at a mean postoperative follow up of 5 years, and survival for patients with recurring anaplastic xanthoastrocytomas is about 2 years.
Vascular Abnormalities
The term occult or cryptic cerebrovascular malformation has been used to describe any vascular malformation that cannot be detected angiographically (30,31). Angiographically occult vascular malformations (AOVMs) have been subclassified into four groups: AVMs, venous angiomas, cavernous angiomas, and telangiectasias. Lobato and coworkers (32) reported that 43.8% of AOVMs were thrombosed AVMs. Hematoma can obscure the underlying lesion, and CT and MRI may fail to identify specific pathologic subtypes of AOVMs (33). Seizures are the most common clinical presentation of occult AVMs (34). Wharen and colleagues (34) reported that 72% of patients with occult AVMs have seizures; the most common presentation is in the temporal lobe, producing complex partial seizures (35). In contrast, simple partial seizures can occur when occult AVM lesions are located in other neocortical areas (4,36). MRI of a lesion shows a serpiginous cluster of low-signal-intensity flow voids with areas of mixed signal intensity where the blood flow is slower or turbulent. Relation of feeding arteries and draining venous channels can be further appreciated by MR angiography. Arterial flow is visualized by three-dimensional time-of-flight techniques; the slower flow in areas of venous drainage is best seen by two-dimensional time of flight (37).
Intracranial hemorrhage is the most significant clinical manifestation of an AVM. An unruptured AVM carries a 4% yearly risk of hemorrhage; after a bleed the likelihood of recurrent hemorrhage is 6% in the first year and 4% in subsequent years (38, 39, 40). Morbidity caused by AVMs may also result from repeated minor hemorrhages or ischemia of surrounding brain as a result of cerebral steal. Therefore, consideration of treatment should be given to most patients with a diagnosed AVM.
Cavernous angiomas are frequently encountered in patients with intractable epilepsy. They are highly epileptogenic as a result of hemosiderin-induced gliosis. Histology shows fine vascular walls with no musculosa or elastin. Vascular growth factors are usually highly represented (Ki67, vascular endothelial growth factor, proliferating cell nuclear antigen). Cavernous angiomas have been implicated in inducing secondary epileptogenic foci, leading to dual pathology (41). The MRI appearance is characteristic and shows a central core of increased intensity with a surrounding rim of decreased intensity on T2-weighted sequences, and multiloculated rounded areas of increased signal on T1-weighted sequences (37). There is no edema and little mass effect. The small size of afferent vessels, presence of thrombosis, and relative paucity of flow through these lesions make them difficult to detect on angiography.
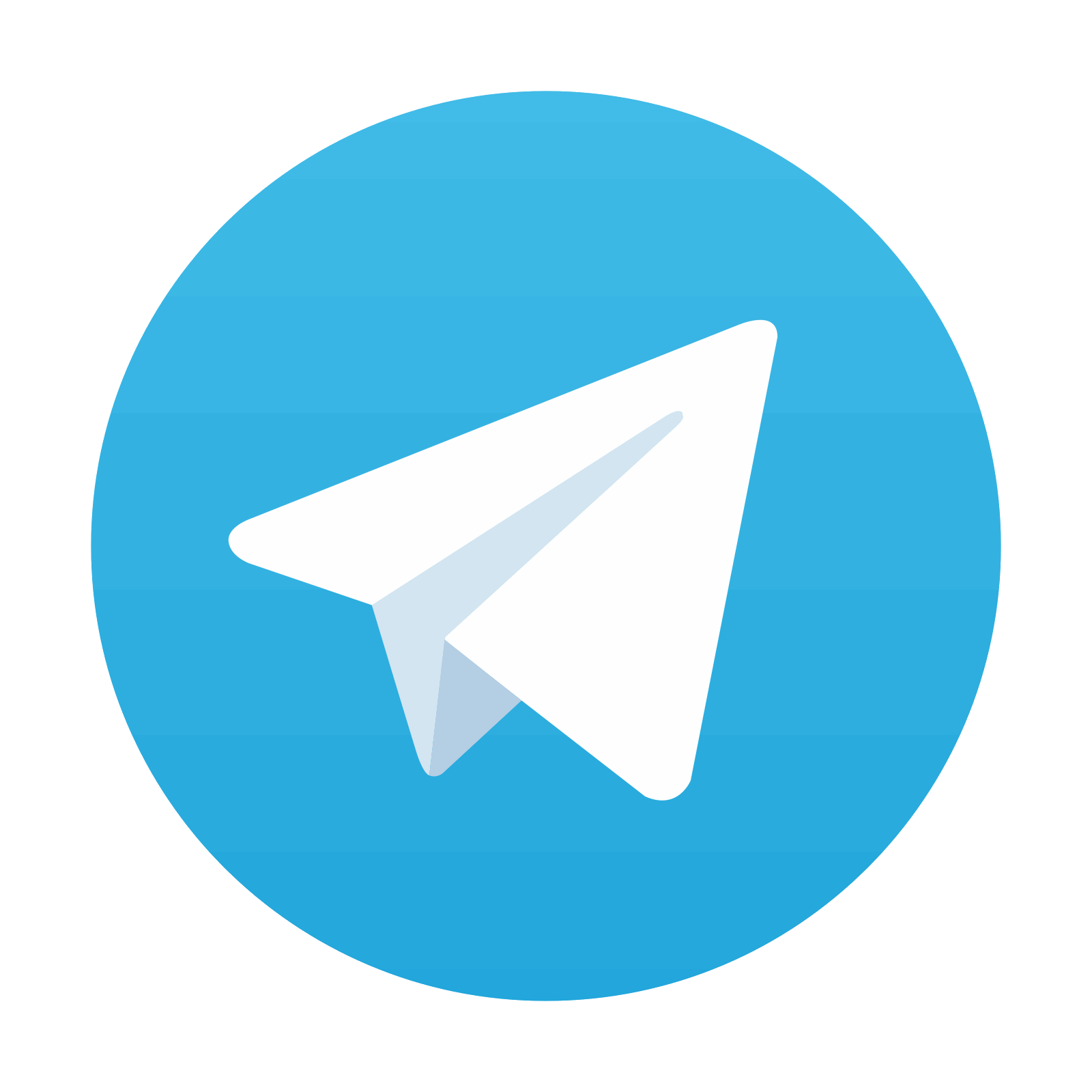
Stay updated, free articles. Join our Telegram channel

Full access? Get Clinical Tree
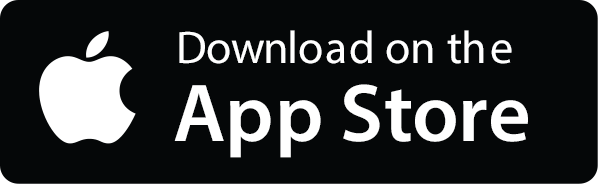
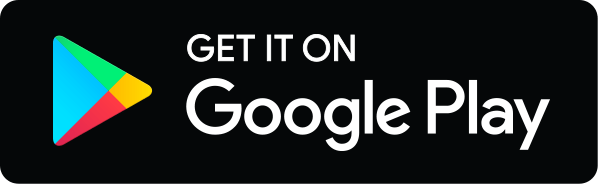