Chapter 21 Respiratory Physiology
Central Neural Control of Respiratory Neurons and Motoneurons during Sleep
Respiratory Neurobiology: Basic Overview
Medullary Respiratory Neurons and Motoneurons
There are bilateral columns of neurons in the medulla with activity patterns that vary in phase with some component of the respiratory cycle. The dorsal respiratory group (DRG) is located in the dorsomedial medulla, specifically in the ventrolateral nucleus of the solitary tract, and contains predominantly inspiratory neurons (Fig. 21-1).1,2 The DRG and the other subnuclei of the solitary tract are also the primary projection sites for vagal afferents from the lung, and afferents from the carotid and aortic chemoreceptors and baroreceptors, that exert important reflex influences on breathing. These projections indicate that the nuclei of the solitary tract, including the DRG, are key sites of integration of sensory information from the lung, as well as information regarding the prevailing levels of arterial PCO2, PO2, pH, and systemic blood pressure. The ventral respiratory group (VRG) extends from the facial nucleus to the first cervical segment of the spinal cord and contains both inspiratory and expiratory neurons (see Fig. 21-1).1,2 The nucleus ambiguus also consists of a rostrally to caudally extending column neurons expressing respiratory-related activity, with sub-regions regions containing motoneurons that innervate the muscles of the larynx and pharynx that are not considered part of the VRG.3 In addition to the nucleus ambiguus, rostrally to caudally the VRG is comprised of Bötzinger complex (expiratory) neurons, pre-Bötzinger complex (inspiratory) neurons, rostral retroambigualis (predominantly inspiratory) neurons and caudal retroambigualis (predominantly expiratory) neurons (see Fig. 21-1).1,2 The VRG and DRG contain both bulbospinal respiratory premotoneurons (i.e., neurons that project to spinal motoneurons, which in turn innervate the respective respiratory pump and abdominal muscles of breathing), and propriobulbar neurons (i.e., neurons that project to, and influence the activity of, other medullary respiratory neurons but themselves do not project to motoneurons) (see Fig. 21-1).1,2 The hypoglossal, trigeminal, and facial motor nuclei also innervate muscles important to pharyngeal motor control and the maintenance of upper airway patency (see Fig. 21-1).4 It is important to stress, however, that the expression of respiratory-related activity is not restricted to neurons of the DRG, VRG, and cranial motoneurons innervating the pharyngeal and laryngeal muscles. For example, neurons expressing respiratory-related activity in the pons (i.e., the pontine respiratory group; PRG in Fig. 21-1) are thought to play an important role in shaping the activity of medullary respiratory neurons during breathing.3
Pre-Bötzinger Complex
Pre-Bötzinger complex neurons have pacemaker-like properties that are thought important to the generation of the basic respiratory rhythm and the expression of rhythmic neuronal activity elsewhere in the respiratory network (see Fig. 21-1).5,6 Respiratory rhythm-generating pre-Bötzinger complex neurons coexpress mu-opioid and neurokinin-1 receptors (i.e., the receptors for Substance P) that slow and increase respiratory rate respectively.6 The presence of mu-opioid receptors on pre-Bötzinger complex neurons may explain a component of the clinically important phenomenon of respiratory rate depression with opioid drugs.7 The development of uncoordinated (ataxic) diaphragm breathing after lesions of neurokinin-1 expressing pre-Bötzinger complex neurons in animal studies, with this abnormal breathing first appearing in sleep,8 suggest that pre-Bötzinger complex neurons may contribute to normal breathing in vivo. Loss of pre-Bötzinger complex neurons, for example, with aging, may predispose a person to abnormal breathing and central apneas in sleep.6
Neuronal Connections
The anatomical connections between the neurons that comprise the essential respiratory network (i.e., respiratory propriobulbar, premotoneurons, and motoneurons), and the membrane properties of these cells, are ultimately responsible for the two key components of overall respiratory activity, that is, the generation of respiratory rhythm and the shaping of the central respiratory drive potentials that activate respiratory motoneurons (pattern generation). An analysis of the mechanisms involved in the generation of the basic respiratory rhythm is outside the scope of this chapter, and the interested reader is referred to excellent summaries of the concepts underlying pacemaker models (where respiratory rhythm is intrinsic to some cells, and these cells then drive others in the respiratory network), network models (where respiratory rhythm is dependent upon the inhibitory and excitatory synaptic connections between neurons, and the tonic excitation derived from both the respiratory chemoreceptors and the reticular formation), and hybrid models.1,3,6 With respect to the tonic drive to the respiratory system arising from the respiratory chemoreceptors, this would include both the peripheral and central chemoreceptors, the latter including neurons at the ventral medullary surface such as the retrotrapezoid nucleus, as well as inputs from CO2-activated sleep state–dependent neurons of the aminergic arousal system (e.g., serotonin and noradrenergic neurons, see the following section of this chapter).9 There are further aspects of the organization of the central respiratory network that are particularly relevant to understanding the effects of sleep on respiratory neurons and motoneurons and these concepts are discussed briefly later.
During inspiration the central respiratory drive potential is transmitted to phrenic and intercostal motoneurons via monosynaptic connections from inspiratory premotor neurons of the DRG and VRG (see Fig. 21-1).1 Bötzinger complex expiratory neurons have widespread inhibitory connections throughout the brainstem and spinal cord, and these neurons inhibit inspiratory premotoneurons and motoneurons during expiration (see Fig. 21-1). Caudal retroambigualis neurons also increase the excitability of spinal expiratory motoneurons in expiration (see Fig. 21-1), although this excitation does not necessarily reach the threshold to manifest as expiratory muscle activity.
Of physiological and clinical relevance, these fundamental aspects of the neural control of spinal respiratory motoneuron activity appear to be different than the mechanisms controlling the activity of pharyngeal motoneurons. For example, animal studies show that the source of inspiratory drive to hypoglossal motoneurons is different from the source of drive to phrenic motoneurons, being predominantly from the reticular formation lateral to the hypoglossal motor nucleus (lateral tegmental field) for the former, and from bulbospinal VRG and DRG neurons for the latter (see Fig. 21-1).1 Importantly, the reticular formation provides a significant source of tonic drive to the respiratory system, with this drive being particularly affected in sleep.3
Further differences in the functional control of pharyngeal and diaphragm muscles is shown by the observation that, unlike phrenic motoneurons, hypoglossal motoneurons are not actively inhibited in expiration.1 Accordingly, the activity of the genioglossus muscle in expiration is simply a manifestation of the prevailing tonic inputs. The practical implication of this circuitry is that the overall activation of hypoglossal motoneurons during breathing is comprised of an inspiratory drive adding to a continuous tonic drive that persists in expiration when the inspiratory activation is withdrawn. Moreover, this tonic drive to the pharyngeal muscles, which contributes to baseline airway size and stiffness, is most prominent in wakefulness but withdrawn in sleep, so leading to an upper airspace that is more vulnerable to collapse. A more detailed analysis of the neural mechanisms controlling the activity of respiratory neurons and motoneurons follows a brief overview of the brain mechanisms modulating the states of wakefulness, non–rapid eye movement (NREM) sleep and REM sleep.
Sleep Neurobiology: Basic Overview
Wakefulness
Figure 21-2 shows some of the main neuronal groups contributing to the ascending arousal system from the brainstem that promotes wakefulness. This ascending arousal system includes the cholinergic laterodorsal and pedunculopontine tegmental nuclei that promote cortical activation via excitatory thalamocortical projections.10 The ascending arousal system also incorporates the aminergic arousal system that originates from brainstem neuronal groups principally containing serotonin (dorsal raphé nuclei), noradrenaline (locus coeruleus), histamine (tuberomammillary nucleus), and dopamine (ventral periaqueductal grey). Orexin neurons from the perifornical region of the hypothalamus and cholinergic neurons from the basal forebrain also contribute to this ascending arousal system.10 Overall, multiple neuronal systems contribute to cortical arousal and wakefulness. These neuronal systems are also positioned to influence respiratory neurons and motoneurons via their anatomical projections to the pons, medulla, and spinal cord (see Fig. 21-2).
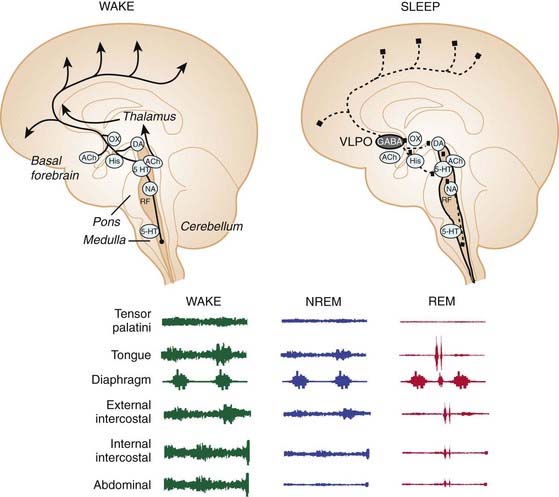
Figure 21-2 Saggital section of the brain showing the main wake and sleep-generating neural systems.
In wakefulness, acetylcholine (ACh); orexin (OX); histamine (His); dopamine (DA); 5-hydroxytryptamine (5-HT); and noradrenaline (NA) containing neurons contribute to brain arousal (arrowheads). This ascending arousal system is inhibited in sleep by gamma-aminobutyric acid (GABA)-containing neurons from ventrolateral preoptic (VLPO) neurons (inhibitory projections shown by dashed lines). By their anatomical projections to the pons, medulla and spinal cord, these wake- and sleep-promoting neuronal systems are also positioned to influence respiratory neurons and motoneurons (see Fig. 21-1). However, whether the influences of the different arousal-related neurons is excitatory or inhibitory will depend on the receptor subtypes activated (this uncertainty is shown by solid squares). Overall, these changes in neuronal activities across sleep–wake states, and their impact on respiratory neurons and motoneurons, mediate the stereotypical changes in the tonic and respiratory components of activity for different respiratory muscles, and their different susceptibilities to motor suppression in sleep. RF depicts the reticular formation.
(Adapted from Saper CB, Scammell TE, Lu J. Hypothalamic regulation of sleep and circadian rhythms. Nature 2005;437:1257-1263.)
NREM Sleep
Sleep is actively generated by neurons in the ventrolateral preoptic area, anterior hypothalamus, and basal forebrain (see Fig. 21-2).10 These neurons become active in NREM sleep, an effect influenced by the thermal stimulus that accompanies the circadian-mediated decline in body temperature at normal bedtime.11 This circadian-mediated decline in body temperature is mediated by a change in the set-point of hypothalamic temperature-regulating neurons, which initially leads to a relative “warm stimulus” as body temperature is at first higher than the new set-point, that is, before heat loss occurs. This warm stimulus activates NREM sleep-active hypothalamic neurons and so promotes sleep onset. This effect of internal body temperature on sleep is distinct from the influences of ambient environmental temperature on sleep regulation. Activation of ventrolateral preoptic neurons leads to a direct suppression of cortical arousal, this via ascending inhibitory cortical projections. Ventrolateral preoptic neurons also promote sleep by descending inhibition of the aforementioned brainstem arousal neurons via release of gamma-aminobutyric acid (GABA) and galanin.11,12 This effect of GABA explains the sedative-hypnotic effects of barbiturates, benzodiazepines, and imidazopyridine compounds that enhance GABA-mediated neuronal inhibition via interactions with binding sites on the GABAA receptor.13 GABAA receptors are also strongly implicated in respiratory control and are present throughout the respiratory network,14 excessive stimulation of which can promote respiratory depression.15
In summary, sleep onset is triggered by increased GABA-ergic neuronal activity, and this is accompanied by a massed and coordinated withdrawal of activity of brainstem arousal neurons comprising serotonergic, noradrenergic, histaminergic and cholinergic neurons. Given the widespread projections of these sleep state–dependent neuronal groups, these changes in neuronal activity in sleep are also positioned to influence respiratory neurons and motoneurons (see Fig. 21-2).16
REM Sleep
Decreased serotonergic and noradrenergic activity preceding and during REM sleep withdraws inhibition of the laterodorsal and pedunculopontine tegmental nuclei.10,12 This effect leads to increased acetylcholine release into the pontine reticular formation to trigger REM sleep.17,18 Exogenous application of cholinergic agonists or acetylcholinesterase inhibitors (to increase endogenous acetylcholine) into the pontine reticular formation is used to mimic this process experimentally in animal studies, that is, the “carbachol model of REM sleep.”17,18 A significant component of the motor suppression of REM sleep is mediated by descending pathways involving activation of medullary reticular formation relay neurons19 that are inhibitory to spinal motoneurons via release of glycine.20
Despite the strong experimental support for the interaction of pontine monoaminergic and cholinergic neurons as being primarily responsible for the initiation and maintenance of REM sleep, recent evidence has implicated a glutamatergic-GABAergic mechanism.21,22 One of the key differences between the aminergic-cholinergic and the glutamatergic-GABAergic hypotheses of REM sleep generation is that the motor atonia is produced by different pathways, that is, the latter framework does not require a relay in the medullary reticular formation.22 Rather, in the glutamatergic-GABAergic mechanism of REM sleep induction, the REM sleep-active pontine neurons are thought to lead to suppression of spinal motoneuron activity via long glutamatergic projections to the ventral horn of the spinal cord, which then activate local glycinergic interneurons to inhibit motor activity.22 Such a mechanism is likely involved in the strong inhibition of spinal intercostal motoneurons in REM sleep, but whether collaterals from these specific long descending glutamatergic projections also synapse onto glycinergic inhibitory interneurons in the hypoglossal motor pool is not established.16
Control of Respiratory Motoneurons
A characteristic and defining feature of mammalian motor activity is that postural muscle tone is highest in wakefulness, decreased in NREM sleep, and minimal in REM sleep, with the hypotonia of REM sleep punctuated by occasional muscle twitches that are associated with vigorous eye movements and phasic REM sleep events.20 Whether respiratory muscle activity is affected in the same way as postural muscle activity across sleep–wake states is somewhat complicated by the interaction of the primary influence of sleep state (e.g., producing suppression of muscle tone) and any subsequent respiratory response (e.g., to compensate for any hypoventilation). On balance, however, the overall stereotyped pattern of suppression of postural muscle activity across sleep–wake states also typically occurs in respiratory muscles, with the degree of sleep state–dependent modulation being most readily apparent in those muscles that combine respiratory and nonrespiratory (e.g., postural and behavioral) functions such as the intercostal and pharyngeal muscles.23 In these respiratory muscles, decreases in activity typically occur immediately at sleep onset23 indicating a primary suppressant effect of sleep neural mechanisms on the activity of respiratory motoneurons, that is, before any compensatory increase in activity takes place in response to altered blood gases, mechanical loads, or sleep-disordered breathing. In contrast to those respiratory muscles with both respiratory and nonrespiratory functions, the diaphragm has an almost sole respiratory function and undergoes lesser suppression of activity in NREM sleep and is largely spared the motor inhibition of REM sleep (see Fig. 21-2).24 Chapters 22 and 23 provide more detail regarding clinical aspects of the control of breathing and upper airway function during sleep, whereas in this chapter the fundamental mechanisms underlying these effects of sleep on the respiratory system are presented.
Determinants of Respiratory Motoneuron Activity
Tonic and Respiratory-Related Inputs to Respiratory Motoneurons
The changes in muscle tone across sleep–wake states ultimately result from the impact of sleep neural mechanisms on the electrical properties and membrane potential of individual motoneurons located in the respective motor pools in the central nervous system. In turn, the excitability of individual motoneurons changes across sleep–wake states because of varying degrees of excitatory and inhibitory inputs to those motoneurons from sleep–wake related regions in the brain, and from neurons activated during specific behaviors such as purposeful motor acts in wakefulness.16 At each individual motoneuron, therefore, the relative strengths and balance between time-varying excitatory and inhibitory inputs ultimately determines net motor output, with neural activity being generated when the membrane potential rises above threshold for the production of action potentials (Fig. 21-3). In addition to the excitatory and inhibitory nonrespiratory inputs to a motoneuron that alter membrane potential across sleep–wake states, a respiratory motoneuron also receives additional inputs (excitatory and inhibitory) that alter membrane excitability and neural activity in phase with the inspiratory or expiratory phases of the respiratory cycle. Simply put, a respiratory motoneuron resembles a postural motoneuron in its control except that it receives an additional rhythmic drive related to respiration, that is, the central respiratory drive potential. Figure 21-3 highlights that the electromyographic activity recorded in a given respiratory muscle is critically dependent on the overall sum of the respiratory and nonrespiratory (i.e., tonic) inputs to the motoneurons innervating that muscle.
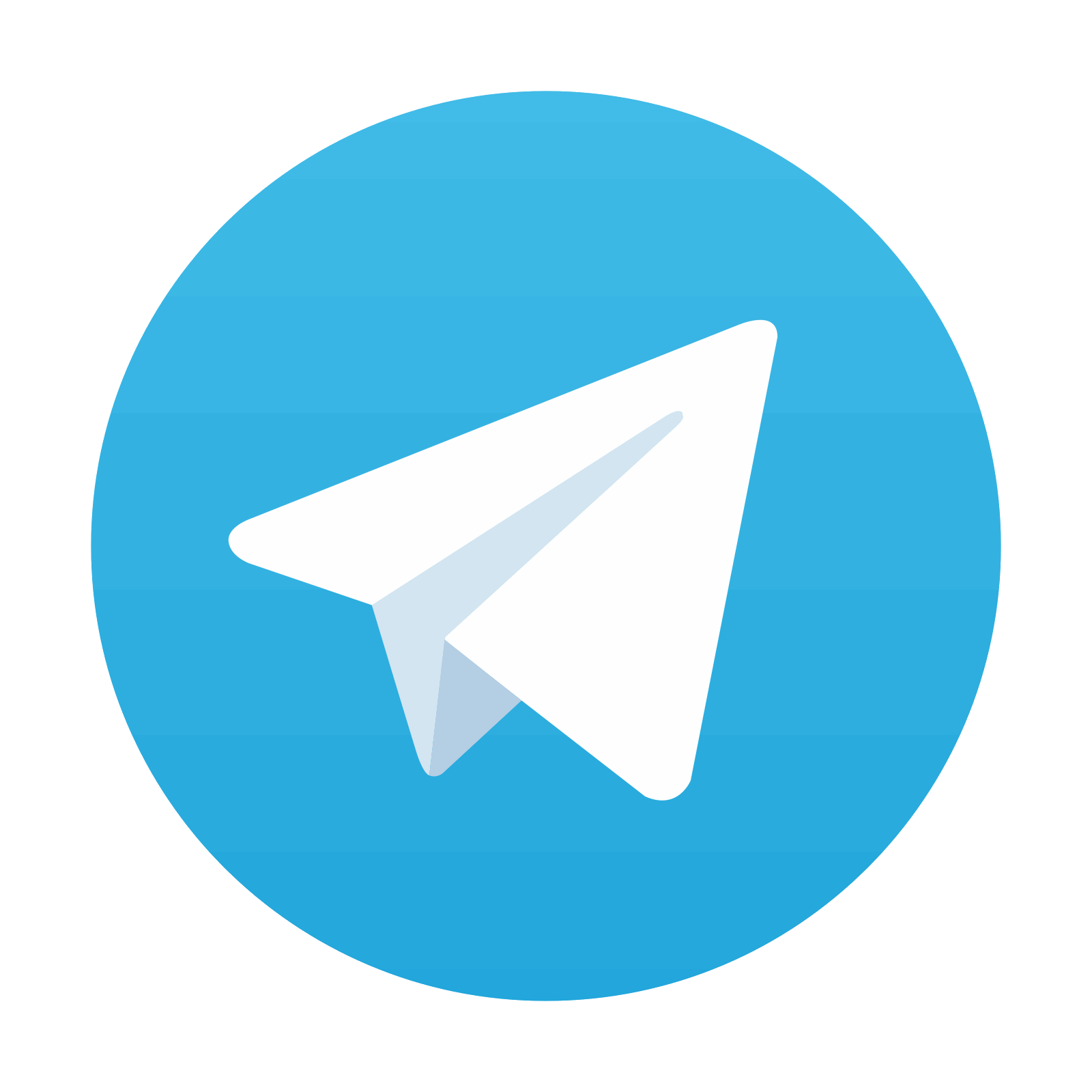
Stay updated, free articles. Join our Telegram channel

Full access? Get Clinical Tree
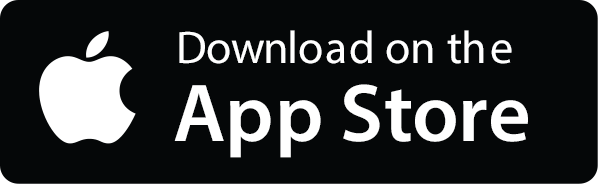
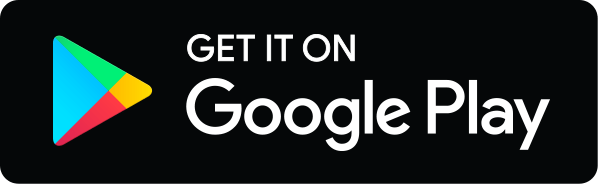