Chapter 24 Respiratory Physiology
Sleep at High Altitudes
Abstract
Sleep disturbance is a common cause of discomfort among the constellation of symptoms after ascent to high altitude. Subjectively, the sensation is that of a restless or sleepless night. Individuals commonly experience awakening from sleep with a feeling of suffocation, taking a deep breath, feeling much improved, and falling back to sleep. Objective observations show associated periodic breathing and frequent awakenings but relatively little change in total sleep time. Sleep stages are generally shifted from deeper toward lighter sleep stages.
Periodic breathing at altitude seems to reflect the respiratory dilemma of acute altitude ascent in which the stimulatory effects of hypoxia are opposed by the inhibitory action of hypocapnic alkalosis. The outcome is respiratory oscillation. Hypocapnic alkalosis induces apnea, which in turn lessens alkalotic inhibition and augments hypoxic stimulation. This triggers hyperpnea, which lessens respiratory stimulation by decreasing hypoxia and increasing alkalosis, leading to recurrent apnea. The occurrence of altitude related apnea with lessening of ventilatory stimuli is enhanced during sleep.
On balance, the poor subjective quality of sleep seems to reflect the fragmentation of sleep by frequent arousals linked to the marked changes in respiratory pattern of periodic breathing. Arousals commonly occur at the transition from the end of apnea to the onset of hyperpnea. Subsequent acclimatization to altitude is associated with lessening of periodic breathing and better-quality sleep.
The most common treatment is prophylactic administration of acetazolamide, an inhibitor of carbonic anhydrase, which likely works by reducing alkalotic ventilatory inhibition. The results of recent studies suggest that benzodiazepines and other sleep-promoting agents may improve sleep quality without apparent adverse effects.
In this chapter, relevant features of acute physiologic adjustment to high altitude are briefly summarized; then the characteristics of the sleep disturbance at high altitude, its pathogenesis, and therapeutic interventions are reviewed. Although much of the focus is on sleep after acute ascent to high altitude, alterations in sleep during long-term altitude exposure are also briefly mentioned. Information in this chapter may also be relevant to the pathophysiology of central sleep apnea at low altitude (see Section 13).
Physiologic Adjustment to High Altitude
Primary among the changes in physical environment that attend the ascent to altitude is a decrease in barometric pressure such that although the fractional concentration of O2 is similar to that at sea level, O2 tension—the product of fractional concentration and barometric pressure—is reduced (Fig. 24-1). This decreased O2 tension of ambient air presents a threat to arterial and tissue oxygenation and elicits a series of responses that may act to minimize tissue hypoxia. These consist of early increases in ventilation and cardiac output, and, during more prolonged exposure, rises in circulating red cell concentration and adaptive changes in peripheral tissue, including increased spatial density of capillaries and mitochondria. Reading Chapters 21 and 22 may help one to further understand the physiological changes associated with sleep and breathing in high altitude.
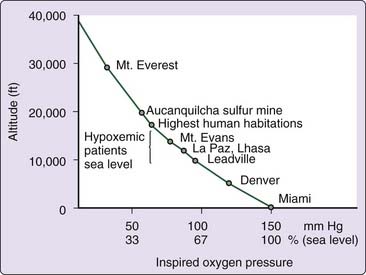
Figure 24-1 Relationship between altitude and inspired O2 pressure.
(From Kryger MH. Pathophysiology of respiration. New York: John Wiley & Sons; 1981.)
Increased Ventilation
Probably the earliest, best studied, and one of the most important of these responses is increased ventilation, which acts to minimize the extent of alveolar hypoxia and arterial hypoxemia in the face of a decrease in ambient O2 tension. The magnitude of the ventilatory response increases with increasing altitude, but it also varies considerably among individuals at a fixed altitude. This variability in part reflects intrinsic, interindividual differences in the strength of the basal (preascent) ventilatory response to hypoxia.1 In addition, ventilation progressively increases over several days after ascent to high altitude. This gradual increase occurs despite the fact that the increasing ventilation is lessening hypoxia, which is the presumed stimulus to breathing, and increasing hypocapnic alkalosis, which is a ventilatory inhibitor. This is the phenomenon of ventilatory acclimatization to high altitude, which is manifested as a progressive decrease in arterial PCO2 (PaCO2) with increasing ventilation over several days. Although the mechanism of acclimatization is debated, studies in humans and animals suggest that increased hypoxic sensitivity of the carotid body may be a major contributor.2 In any case, it is during the early phase of altitude adjustment, shortly after ascent, that sleep disturbances appear to be most marked; they tend to improve during the period of acclimatization.
Sleep Disturbances
The earliest systematic study of sleep at altitude was a report in 1970 of studies of subjects working in Antarctica, where a combination of geographic elevation and terrestrial spin produces decreased barometric pressure ranging from 485 to 525 mm Hg, equivalent to an altitude of 4500 to 5000 meters. The men stationed there experienced a sleep disturbance termed polar red-eye, and electroencephalographic studies showed major disruption of sleep with a marked decrease in slow-wave sleep (stages 3 and 4).3 Although these changes were ascribed to hypobaric hypoxia, the effects of isolation and disturbances of light–dark cycle that are typical in persons living at the South Pole clouded their interpretation.
As research at low altitude began to elucidate the close links between breathing and sleep and showed that sleep disruption was often closely linked to changes in respiratory rhythm, such as occurs in sleep apnea, studies were performed at high altitude with simultaneous monitoring of sleep state and respiratory pattern. Breathing and sleep interactions were studied in normal subjects at sea level and during a stay of several days on the summit of Pike’s Peak (4300 meters).4 On the initial night after ascent, most subjects exhibited periodic breathing that was present during roughly half the time asleep but varied among subjects from 0% to 93%. Sleep was characterized by significant decreases in stages 3 and 4, with an increased number of arousals (Fig. 24-2). In most but not all subjects who initially showed periodic breathing, this tended to decrease over subsequent nights, with a decreased number of arousals. Although there was a trend toward more wakefulness, the duration of sleep (total sleep time) was not significantly reduced compared with that at sea level.
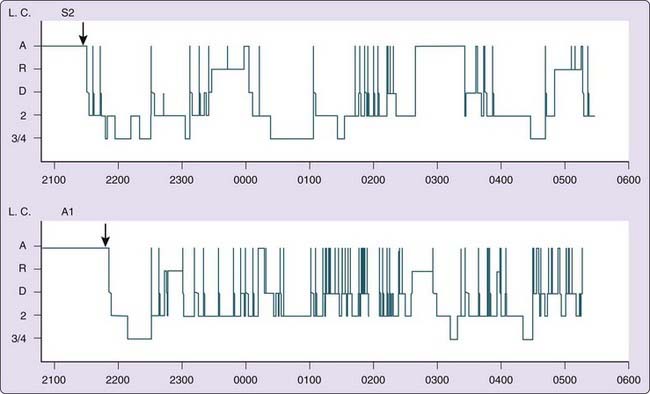
Figure 24-2 All-night sleep plots for a single subject during a night at sea level (upper plot) and the first night at altitude (lower plot). Time in hours is plotted on the horizontal axis. Lights out occurred at the small vertical arrow in each plot. Sleep stage is shown on the vertical axis: A, awake; R, REM sleep; D, stage 1 sleep; 2, stage 2 sleep; 3/4, stages 3 and 4 sleep combined. Sleep at high altitude was associated with increased fragmentation by frequent awakenings and a reduction in stage 3/4 sleep.
(Reprinted from Reite M, Jackson D, Cahoon RL, et al. Sleep physiology at high altitude. Electroencephalogr Clin Neurophysiol 1975;38:463-471.)
Although most of these subjects complained of poor-quality sleep, this could not be related to abbreviated sleep, which, as mentioned, was of normal duration. Rather, it seemed associated with an increased number of awakenings. Many of these were synchronous to the transition from termination of apnea to onset of hyperpnea and thus were similar in some respects to the arousal seen in sleep apnea syndromes at low altitude. Although this suggests that periodic breathing, frequent awakenings, and poor-quality sleep are mechanistically interrelated, there must be some reservation about this because increased awakenings in one subject occurred in the absence of periodic breathing and because oxygen administration abolishes periodic breathing but not the increased frequency of awakenings.4–7 It appears, however, that most of the sleep fragmentation at altitude is related to the increased frequency of arousals that are temporally linked to apneas.8
Poor subjective quality of sleep might also reflect altitude-associated changes in sleep stage distribution. Most studies find that sleep at high altitude is characterized by an increase in light sleep (stage 1) with a decrease in stage 2 sleep, and a relative paucity of deeper stages (3 and 4) of non–rapid eye movement (NREM) sleep. Total sleep time is usually unchanged, but there is a significant increase in time spent awake, and there is shortening of sleep epochs, which are fragmented by frequent arousals.4,5,7,9 There seems to be no consistent change in the amount of rapid eye movement (REM) sleep, which is variably found to be either unchanged,4,7 increased, or decreased6,10 at altitude. The disparity between subjective evaluation of sleep quality and the objective findings of normal sleep duration most likely reflects the importance of sleep continuity in the apparent subjective quality of sleep and suggests that sleep fragmentation despite normal cumulative duration produces the impression of sleeplessness.4,8,11,12
As mentioned earlier, total sleep time is remarkably preserved or even increased4,8,11,12 in the face of the disruptive effect of periodic breathing. Perhaps this paradox is explained by the frequent but largely unstudied observation that ascent to altitude induces sleepiness, which is consistent, prompt, and often profound. Although this may be due to hypoxia, a study in normal subjects at low altitude showed marked sleepiness induced by brief voluntary hyperventilation, pointing to a potential role of hypocapnia.13 Regardless of its cause, the hypnotic effect of altitude could contribute to the maintenance of total sleep duration. Hypocapnia may also suppress REM sleep; a study in sleeping cats at simulated altitude and during mechanical hyperventilation showed decreases in REM sleep that were reversed by CO2 administration.14
Periodic Breathing
In the mid-19th century, Cheyne and Stokes described the crescendo–decrescendo breathing pattern in cardiac patients that now bears their names.15 That periodic breathing is frequent during sleep in normal individuals at high altitude was observed shortly thereafter by Tyndall in 1857, by Egli-Sinclair and Mosso in 1893 and 1894, and by Douglas and colleagues16 in 1913, and it continues to be a consistent finding in current studies of sleep after ascent to high altitude.4,9,17–20 The respiratory dysrhythmia of sleep at altitude is one of machinery-like periodicity (Fig. 24-3) similar to that seen at low altitude in patients with heart failure (see Chapter 122) or with central nervous system (CNS) disorders.
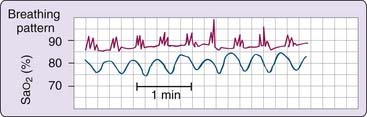
Figure 24-3 Breathing pattern and arterial oxygen saturation (SaO2) in a normal subject during sleep at high altitude (4300 meters). Such a pattern is seen throughout much of sleep in most individuals after ascent. There is characteristic, monotonously repetitive, machinery-like periodic alternation of apnea and repetitive clusters of hyperpneic breaths.
(From Weil JV, Kryger MH, Scoggin CH. Sleep and breathing at high altitude. In: Guilleminault C, Dement WC, editors. Sleep apnea syndromes. New York: Alan R Liss; 1978. p. 119-136.)
The temporal pattern of periodic breathing and its linkage to sleep stages show some night-to-night variation and considerable intersubject differences,4,6,10,19,21–25 yet on balance, periodicity is usually evident early in sleep and during light stages. Periodic breathing at altitude may also occur in wakefulness, especially during periods of drowsiness.26–28 The pattern, as mentioned, is similar to that of patients with central apnea at low altitude, but with shorter cycle length ranging from 12 to 34 seconds, which progressively shortens with increasing altitude29–31 and differs from the longer cycles of 40 to 90 seconds in patients with heart failure.32 The shorter cycle length at altitude is thought attributable to increased peripheral chemoreceptor sensitivity.33 The most obvious aspect of this periodicity is the oscillation of tidal volume, and with a less obvious change in breathing frequency. However, there are subtle, but parallel, variations in frequency that produce a reinforcing effect on ventilatory oscillation.29 Like periodic breathing at sea level, periodicity at altitude is often initiated by movement or a deeper breath with a transient increase in hypocapnia. Periodicity at altitude is also most prevalent in light, stage 1 or 2 sleep. Although deeper stages of NREM sleep are relatively rare after acute ascent, the propensity to periodic breathing seems much reduced at these times.
The most striking influence of sleep stage on periodic breathing is the observation in most,4,7,25 but not all,5,6 studies that REM sleep promptly and consistently terminates periodicity and restores regular breathing However, periodicity seems to persist in REM sleep at altitudes greater than 4300 meters.8
To our knowledge, no data are yet available on the long term risks of high-altitude sleep on health for either occasional or regular high-altitude sleepers. During sleep, ventilation and oxygenation fall below waking values, and the relative sleep induced decreases are similar at altitude and at sea level.34 However, the important difference is that at altitude, basal (awake) oxygenation and PCO2 are lower and thus closer to critical ventilatory thresholds (Fig. 24-4). Oxygen tensions fall closer to the descending limb of the dissociation curve, where values are associated with desaturation and are nearer the threshold for stimulation of ventilation. Similarly, CO2 tensions fall to values nearer the “dog-leg,” below which CO2 may lose its ventilatory stimulus potential, and they closely approach the PCO2 apneic threshold below which breathing ceases during sleep.35 As a result, small variations in gas tensions have much greater effects on ventilation at altitude with greater stimulation by hypoxia and increased inhibition by hypocapnia.
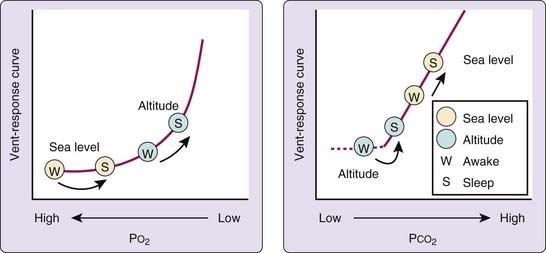
Figure 24-4 A schematic illustration of the relationship of blood gases to ventilatory responses during wakefulness and sleep at sea level and high altitude. At altitude, during wakefulness (W), arterial oxygen tensions (PO2) move lower toward the steeper portion of the hypoxic ventilatory response curve, an effect that is augmented during sleep (S). Hyperventilation causes arterial PCO2 tensions during wakefulness and sleep to decrease and move closer to the “dog-leg” of the CO2 response curve, where the ventilatory response to PCO2 is greatly reduced. The result is that during sleep at high altitude, small changes in the partial pressure of either oxygen (PO2) or carbon dioxide (PCO2) produce much greater changes in ventilation than at low altitude. This sets the stage for respiratory instability.
Respiratory pauses in sleep at altitude are mainly of central origin, unassociated with snoring or other noises suggestive of obstruction and accompanied by decreased rib cage and abdominal activity.5,7,17,19,36 There is no evident association with the usual risk factors for obstruction, such as obesity. However, at low altitude, many apneas are “mixed” with both central and obstructive features.37,38 This is most likely a reflection of the phasic “respiratory” nature of many upper airway muscles such that loss of drive reduces activation of both classic inspiratory muscles and those responsible for maintenance of upper airway patency. The hypoxia of altitude may act to decrease airway obstruction. A study of subjects with known obstructive sleep apnea at low altitude showed that at a simulated altitude of 2750 meters obstructive events were entirely replaced by central apneas.39 This likely reflects an increase in ventilatory drive in hypoxia which may augment the activity of muscles of the upper airway.40 On the other hand, sleep studies of subjects living at moderate altitude (above 2400 meters) that were conducted at a lower altitude (1370 meters) in comparison to the altitude of residence, show that at lower altitude, the respiratory disturbance index (RDI) was reduced. This is largely due to a decrease in central events compared to studies at the higher altitude of residence. There was no change in frequency of obstructive apneas, although the latter were more prolonged at a low altitude.41 This finding points to the importance of performing diagnostic sleep studies at the altitude of residence, that is, the places where the subjects are living on a regular basis.
As mentioned, sleep disturbances and periodic breathing seem to be most pronounced during the first few nights after ascent to moderate altitude, with a tendency toward more regular breathing on subsequent nights. However, most studies find substantial interindividual differences in the extent and persistence of periodic breathing and several studies describe the prolonged persistence of periodic breathing over 10 days to 5 weeks at altitudes greater than 4500 meters.19,36,42,43 This might reflect incomplete acclimatization at very high altitudes.
Mechanisms
In the early 1900s, ingenious experiments done at low altitude explored the physiologic mechanisms of periodic breathing and highlighted the importance of both hypoxia and hypocapnia.44 The authors were aware that during wakefulness, breathing was characterized by intrinsic momentum, a “flywheel” effect that caused breathing to continue despite brief hypocapnia. The study revealed also that respiratory periodicity could be readily induced in normal subjects by intense, sustained voluntary hyperventilation with room air, which produced apnea followed by Cheyne-Stokes respiration. When hyperventilation was produced with an O2-enriched gas, apnea was induced without periodic breathing. Such findings indicate the importance of hypoxia in the induction of breathing periodicity. Furthermore, they also support the specific role of phasic hypoxia through the application of dead space containing the CO2 absorber soda lime, which produced rebreathing-induced hypoxia without the usual attendant hypercapnia. When the resulting progressive hyperpnea produced an increase in tidal volume sufficient to bring in “fresh air” replete with O2, the resolution of hypoxia produced apnea followed by recurrent rebreathing-induced hypoxia and perpetuation of the cycle. These findings pointed to the combined roles of hypocapnia, which initiates apnea, and hypoxia, which stimulates hyperpnea, in the genesis of periodic breathing. Similar principles apply at high altitude, as suggested by findings that the administration of O2 or CO2 abolishes periodic breathing.4,17,22,36
Roles of Hypoxia and Hypocapnia
Interpretation of the specific roles of O2 and CO2 is complicated, firstly because O2 administration improves oxygenation but also reduces ventilation and increases PaCO2 with lessening of alkalosis. Secondly, the administration of CO2 corrects respiratory alkalosis but also increases ventilation and improves oxygenation. Despite this difficulty, considerable evidence suggests that although hypoxia may be contributory, hypocapnic alkalosis may have a particularly important role. Respiratory alkalosis is typical in patients with classic Cheyne-Stokes respiration at low altitude45 and occurs in normal subjects in whom apneas during sleep have been noted to occur after transient episodes of decreased end-tidal PCO2 and presumed increase in blood pH.26 The critical role of hypocapnia in periodic breathing in sleep at altitude became clearly evident when periodic breathing in NREM sleep at simulated altitude (barometric pressure of 455 mmHg equivalent to an altitude of 4300 meters) was abolished by a selective increase in PaCO2.17 This was achieved through the administration of low levels of CO2 with added nitrogen to prevent the increase in oxygen saturation (SaO2) that would be anticipated as a result of the stimulation of ventilation by a rising PaCO2. In one subject, during NREM sleep, the appearance of periodic breathing during the induction of hypoxia was temporally related closely to the fall in end-tidal CO2 rather to the decrease in PO2. Similarly, this study showed that breathing was regular in NREM sleep in hypoxia when CO2 was added to maintain isocapnia. However, when CO2 administration was terminated, periodic breathing occurred when the end-tidal CO2 fell, although the extent of hypoxia remained constant.
The Apneic Threshold
An experimental study showed that apneas could be consistently produced at low altitude in NREM sleep through the induction of hypocapnia by passive positive-pressure hyperventilation against a background of either hypoxia or hyperoxia.46 Apnea tended to occur when the end-tidal CO2 was lowered 1 to 3 mm Hg below levels observed during wakefulness (the apneic threshold). Thus, hypoxia alone seems insufficient to produce periodic breathing, for which a fall in PCO2, below an apneic threshold, seems important (Fig. 24-5). Just as apneas at low altitude frequently follow sighs or breaths of increased tidal volume with a drop in PCO2,26 breaths of increased tidal volume with decreased end-tidal PCO2 often trigger periodic breathing at altitude.17,36
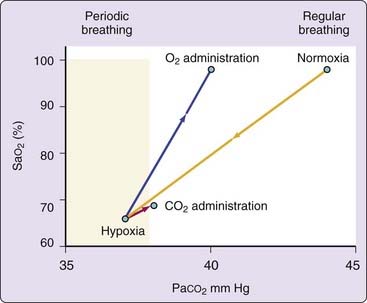
Figure 24-5 Relationship of respiratory pattern to arterial partial pressure of CO2 (PaCO2) and oxygen saturation (SaO2). This figure is a schematic representation of data of Berssenbrugge et al.17 and demonstrates that reduction in PCO2 is a critical determinant of periodic breathing in normal human subjects during hypoxia. Even a small increase in PCO2 after administration of either O2 or CO2 restores normal breathing. This effect seems largely independent of changes in SaO2.
(From Weil JV. Sleep at high altitude. Clin Chest Med 1985;6:615-621.)
It thus appears that the events leading to periodic breathing at altitude begin with hypoxia, which stimulates hyperventilation, and the resulting hypocapnic alkalosis induces apnea, as illustrated in Figure 24-6. Apnea, in turn leads to enhanced ventilatory stimulation by increasing hypoxemia and lessening hypocapnic alkalosis, which promotes subsequent hyperpnea and arousal. The ensuing increase in ventilation lessens hypoxia and restores hypocapnic alkalosis, promoting the recurrence of apnea. Indeed, another study suggested that a stable and slightly elevated PCO2 is necessary for stable rhythmic breathing during low-altitude sleep.26
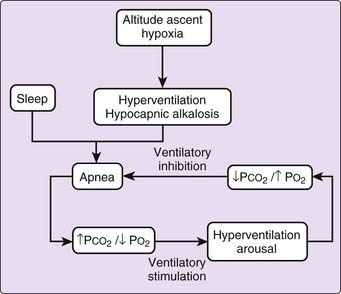
Figure 24-6 Schematic summary of mechanisms responsible for periodic breathing during sleep at altitude. Altitude-induced hypoxia stimulates increased ventilation. The resulting hypocapnic alkalosis together with the effects of sleep promotes apnea. During altitude-related apnea, increasing hypoxemia and lessening of hypocapnic alkalosis stimulate resumption of ventilation and arousal. This augments oxygenation and lessens hypocapnic alkalosis, permitting recurrence of apnea.
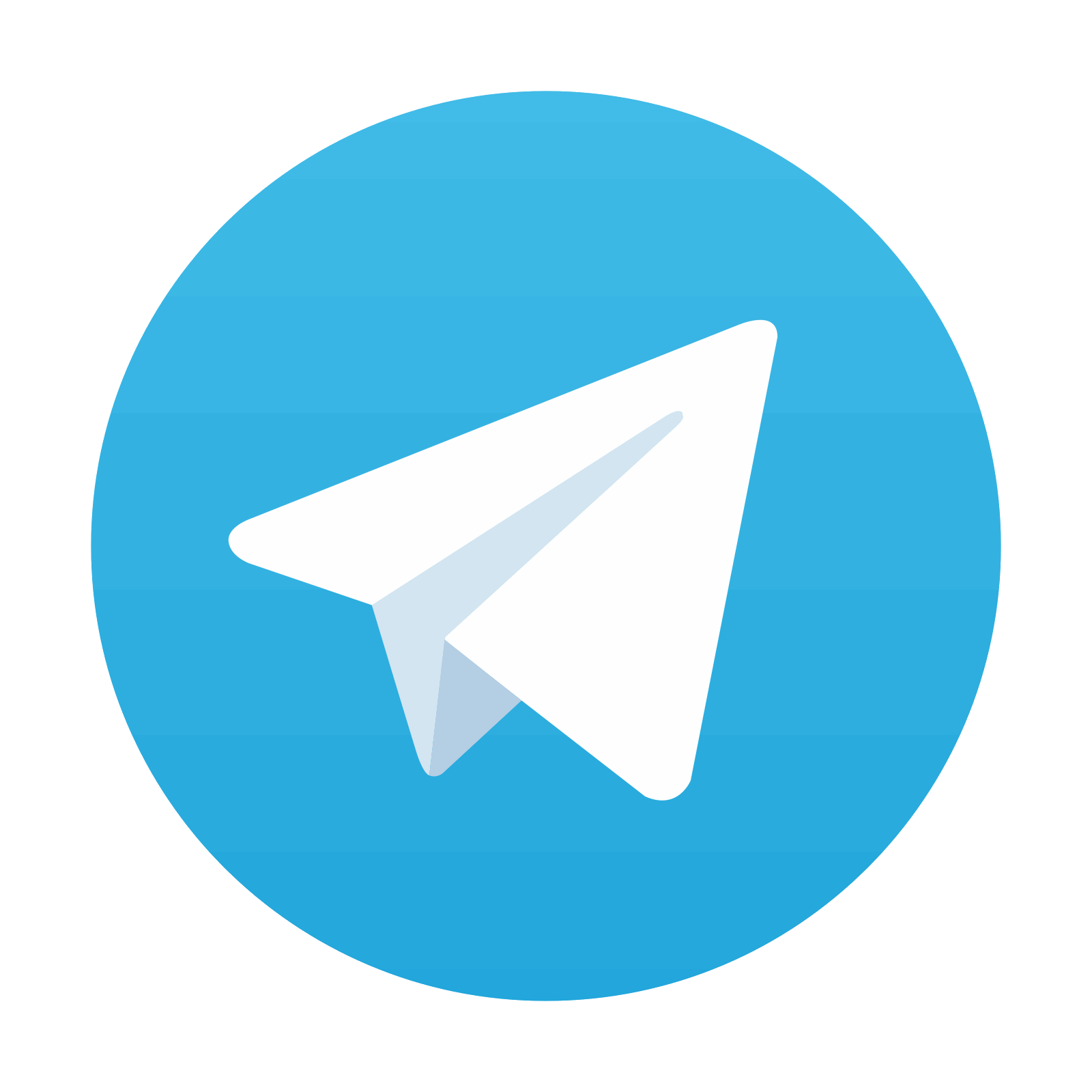
Stay updated, free articles. Join our Telegram channel

Full access? Get Clinical Tree
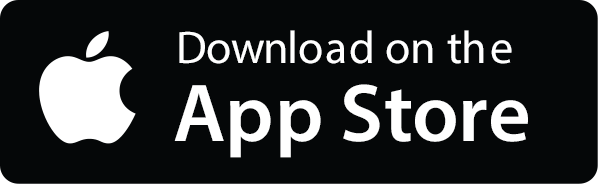
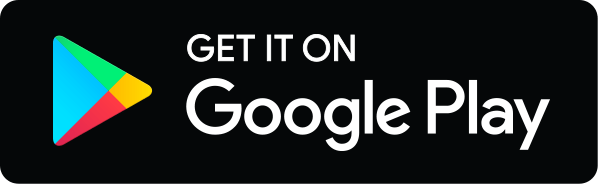