Fig. 11.1
Neural crest migration and schizophrenia. Schematic figure showing the dynamics in Nedd9 expression in trunk premigratory and migratory neural crest cells (NCCs) which, being induced by dermamyotome-derived retinoic acid (RA), is required for their efficient migratory behavior (a). Note that Nedd9 is transiently expressed in the neural tube roof (before NCC delamination), and later on is confined to multipotent premigratory and migratory NCCs (see cells with their cytoplasm depicted in red, outside of the neural tube). Nedd9 is sharply downregulated when migrating NCCs become restricted to the neuronal lineage (see a cell with nucleus depicted in green, expressing Brn3a and Ngn2) at E9-E9.5 in mouse embryos and equivalent stage in chicken. (b) Facial NCCs (FNCCs) are composed by early delaminated non-neural ectoderm cells which give rise to the frontonasal mesenchyme. This structure produces RA, which is required for neural ectoderm-derived FNCCs, cells which might also depend on Nedd9 expression for their motility. Deficiencies in FNCC migration would result in decreased Foxg1 levels (the master transcriptional regulator in forebrain development) and in the ventralization of the most rostral part of the brain, thus affecting the thalamic and pre-tectal nuclei. Such features could be involved in some schizophrenia cases. (c) At E10.5 and equivalent stages in chicken, Nedd9 is expressed in the boundary cap and in the multipotent Sox2+ NCCs located at the periphery of the dorsal root ganglia. It is downregulated as soon as NCCs become restricted to the neuronal or glial lineages. From E12.5 on, neural crest-derived cells no longer express Nedd9, although some glia progenitors named as Schwann cell precursors (SCPs) continue migrating through growing peripheral nerves. Some SCPs detach from nerves, and shortly afterward they turn on MITF expression (a master transcriptional factor regulator of melanocytes development): see cells depicted with red nuclei. Note that some late neural crest cells migrating through the dorsolateral route are also a source of melanocytes. Derivatives of SCPs as they are known today are listed
The Retinoid Acid and the Migratory Capacity of Neural Crest Cells
The motile capacity of NCCs has been shown to depend on the RA-dependent induction of Nedd9 expression [45]; (Fig. 11.1). Nedd9 (also known as HEF1 and Cas-L; a name which stands for neural precursor cell expressed, developmentally down-regulated 9) is a scaffolding protein, member of the Crk-associated substrate (CAS) family, involved in the beta1-integrin signaling pathway, and frequently localized in focal adhesions and found associated with FAK and the Abl kinase. In addition, it has been found expressed in the centrosome and in the mitotic spindle during mitosis. It has been suggested that this protein may play a role in cell adhesion and migration, apoptosis, and cell cycle.
Nedd9 was first reported by Kumar et al. [46] by performing subtraction cloning experiments with the aim of identifying genes highly expressed in the brain during development. Nedd9 was shown to induce upregulation of matrix metalloproteinases, ephrin ligands and receptors, and the NRG receptor ErbB2; nevertheless, both its pathway–target specificity and how those genes are activated remain unknown [47]. Cell adhesion was found to trigger the conversion of Nedd9 isoforms from p105 into p115, which reflects the serine/threonine phosphorylation state and is associated with integrin receptor activation and cytoskeleton organization [47].
In embryonic stem cells, Nedd9 promoter was found to be co-occupied by Sox2 and Nanog, which would suggest its involvement in stem-cell behavior [47]. By means of a screening based on a degenerate PCR approach looking at a homeobox-sequence containing proteins highly enriched in the neural tube and the DRG at intermediate stages of mouse development, Aquino et al. [48] were able to clone a 498 bp corresponding to a fragment in the C-terminal domain of Nedd9. Interestingly, by in-situ hybridization and immunohistochemistry analyses Nedd9 was found to be expressed in multipotent progenitors (able to give rise to all common tissue-specific derivatives) of diverse tissues, including the CNS and the PNS [48].
Moreover, during early CNS development, Nedd9 is expressed in the ventricular zone of the neural tube, from the diencephalon to the caudal spinal cord axial levels [48]. Nedd9 expression is further upregulated in dorso-ventral neural tube domains of multipotent cells, giving rise to Ngn2+ neuronal progenitors in the CNS. Interestingly, these regions are those which show further growth at early neural tube morphogenesis (E10-E12 in mouse; Fig. 11.1). Considering the described role of Nedd9 in the migratory and/or mitosis behavior, whether or not Nedd9 higher expression in such progenitor domains could be associated with tissue morphogenesis remains to be addressed. It is worth noting that Ngn2+ progenitors are among the first neuronal precursors to be born in the CNS. Finally, considering that RA-mediated Nedd9 upregulation makes NCCs responsive to integrin as well as to other pro-migratory signals, it might probably be involved in the onset of their frontward migration within the head.
With regard to the PNS, Nedd9 is induced in neural tube roof by the time of neural crest delamination as well as at the onset of their migration through the sclerotome. Consistent with recent reports confirming the multipotency of the majority of premigratory and migratory neural crest cells [49], most of them were found to co-express Sox10 and Nedd9 [45]. Thus, maintenance of multipotency in NCCs is probably required for their migratory behavior (Fig. 11.1).
It is worth noting that Nedd9 is downregulated as soon as cells become restricted to any of neural lineages in the CNS as well as in the PNS, and consistent with that it was not found to be expressed in the NCC lineage at E12.5 and thereafter. Gain of function of Nedd9 in chicken, by forced expression of its full length, enhances the migratory behavior of NCCs, whereas Nedd9 knockdown was found to inhibit their emigration from the neural tube and the migratory capacity of NCCs once delaminated. Similar results were obtained in boundary cap neural crest stem cells (bNCSCs) by performing in-vitro assays. The reduced Sox10/Nedd9 expression levels found in first Ngn2+/Brn3a+ migrating NCCs, which correspond to neuronal precursors biased to the sensory fate, might suggest a role for Nedd9 downregulation in the formation of the DRG through a reduction in their migratory properties.
Nedd9 function in the migratory behavior of multipotent NCCs was found to be dependent on integrin ligands, since NCCs were only able to efficiently spread in laminin, and Nedd9 siRNA caused a significant reduction in cell spreading area when compared to control. Consistently, while the majority of scrambled siRNA-targeted NCCs showed a migratory phenotype, cells became mostly stationary when Nedd9 was knocked-down [45]. In addition and as was expected, Nedd9 loss-of-function resulted in decreased frequency of focal complexes and actin filaments and the rare appearance of stress fibers in NCCs, which are all signs of their hypomorphic migratory phenotype.
In-vivo quantification analyses of Nedd9 expression levels during NCCs development suggested that it is induced by a signal derived from the dermamyotome, since a peak in Nedd9 immunoreactivity was found in migrating cells located close to the dermamyotome dorsal lip, and these levels decreased in cells which have migrated further down this landmark (Fig. 11.1). Indeed, when neural tube explants were incubated for 6 h in culture medium supplemented with different inducible factor candidates and/or their antagonists/inhibitors (all-trans retinoic acid at low and high levels, citral, BMP4, Noggin, FGF8, Shh, Wnt3a, Wnt3a plus BMP4, Wnt5a or none—control) RA was found to be the only factor likely able to induce Nedd9 expression. Several experimental outcomes support this statement: (1) the highest Nedd9 expression levels were obtained in our in-vitro model system when explants were cultured in presence of high retinoic acid, (2) upon citral treatment (an inhibitor of retinoic acid biosynthesis), Nedd9 was down-regulated in NCCs when compared to control condition, and (3) retinoic acid injection in pregnant mothers up-regulated Nedd9 expression in trunk neural crest of E9.5 mouse embryos after 6 h of treatment. A minor increase in Nedd9 expression was seen for FGF8, Wnt3a, and Shh treatments when compared to controls; however, this could eventually be explained by direct or indirect Nedd9 expression regulation and/or a role played by these factors in maintaining the multipotent status of NCCs in vitro. Results suggesting retinoic acid induction of Nedd9 in NCCs are also consistent with dynamic changes in in-vivo Nedd9 expression patterns [47]. It is known that the expression of Raldh2, the main enzyme synthesizing retinoic acid in the trunk, increases in a posterior-to-anterior fashion in somites and peaks at the level of somite dissociation into dermamyotome and sclerotome. And consistently, and since Nedd9 is involved in NCC motility, first NCCs migrating down through the rostral half of the somite do so at this very same trunk level.
In conclusion, a retinoic acid regulation of Nedd9 expression probably gives multipotent NCCs the competence to respond to extracellular signals and to initiate migration through the sclerotome in an integrin-dependent manner. Nedd9 might exert its effects in NCCs through regulation of focal complexes and actin filaments, required for cell adhesion and spreading and for the development of traction forces which altogether drive motility.
Schwann Cells in the Injured Central Nervous System
Schwann cells are one of the NCC–glia derivatives which can be found associated with one or several axons, depending on whether they correspond to a myelinating or to a non-myelinating phenotype, respectively. Apart from their supportive role to axons and myelin production, Schwann cells have also been shown to be able to: (1) present antigens and produce immunologically-relevant cytokines (in certain cases); (2) modulate neuromuscular synapse formation, actively respond to neurotransmitters, and repair the neuromuscular junction; (3) modulate pain, and (4) regulate the activity of haemopoietic stem cells [50]. Interestingly, Schwann-like cells were shown to contribute to remyelination in the CNS in demyelinating injury models and in multiple sclerosis. Moreover, some of the Schwann-like cells were found to originate from OPCs (the NG2-glia; [51]). In addition, neural progenitor cells from the subventricular zone might possibly give rise to some Sox10+/GFAP+ Schwann like-cells, an issue which requires further examination [52]. In fact, a week after injury some Sox2+/Sox10+ cells were found within the ependymal layer of the spinal cord central channel, which could consist of progenitors capable of originating Schwann-like cells (47; Fig. 11.2). Interestingly, 9 days after weight-drop injury many Sox10+/Sox2+ cells could be found rostrally to the injury core (47; Fig. 11.2). CNS-derived Schwann cells show properties which differ from those of NCC-derived ones, and resemble those of OPCs, such as: (1) they express higher levels of O4/A2B5 and lower levels of S100 expression; (2) they display prominent outward rectifier KD currents, and (3) their K+ currents are more efficiently inhibited by broad-spectrum potassium channel blockers (TEA, Ba2+; [53]). Some of them might also correspond to previously reported aldynoglial Schwann cells [54].


Fig. 11.2
Schwann/Schwann-like cells in spinal cord regeneration. These representative microphotographs show changes in cellular subpopulations as well as axonal regeneration through the injured area, in a rat weight-drop model. Unless stated, pictures were taken from saggital sections of the spinal cord. Most of them correspond to animals treated with mouse Rosa26 boundary cap neural crest stem cell-derived Schwann cells (bNCSCs). In most cases, grafted cells die shortly after transplantation. Nevertheless, only when injected alive were they able to induce changes resulting in significant improvements in BBB locomotor score studies. (a) A composite image showing rostral aspects of the injury zone (9 days after injury and 2 days after bNCSC grafting). Note the significant increase in the density of Sox2+/Sox10+ oligodendrocyte progenitor-like cells (empty arrowheads) within the spinal cord parenchyma at less affected and anatomically more conserved areas of the spinal cord. In addition, Sox2+/Sox10+ cells are found within the ependymal layer (arrows, a layer which is disorganized after injury; see higher magnifications images of insets at the upper-right corner). RIP is a marker of oligodendrocytes. Scale bar: 200 μm. (b) Changes in endogenous cellular populations in between two (upper panel) and 14 (lower panel) days after cellular transplantation in rostral (left), middle (central) and caudal (right) regions within the injury core. Note that the density of axons (arrows, immunolabeled using antibodies against neurofilament -NF- 160KDa and 200 KDa epitopes) and of associated Sox2−/Sox10+ glia (empty arrowheads) are increased at all regions analyzed with time. In addition, while few axons could be observed at the middle and only debris are seen at distal/caudal regions of the injury zone at early time points analyzed, they are increased in numbers and appear forming bundles at both regions at later time points, suggestive of spinal cord regeneration. Scale bars: 50 μm. (c) Representative figures showing endogenous Sox2−/ Sox10+/p75+ glial cells (arrows) at central aspects of the injury core at 2, 7, and 14 days after cellular transplantation. Note the increase in the density of these cells with time, and their organization in bundle-like structures (consistent with b). These cells are probably derived from a Schwannosis process, although some of them might originate from spinal cord progenitors (see a), a matter that requires further studies. Scale bars: 50 μm). (d) Many Sox10+ cells at the middle of the injury core (14 days after bNCSC grafting) were also P0+ (and thus peripheral Schwann cells which have invaded the spinal cord) and were able to remyelinate axons (arrows) or served as scaffolds for axonal regrowth (empty arrows; in green). Note also the presence of Sox10+/P0− cells (empty arrowheads) which could in part be derived from spinal cord progenitors. Scale bar: 20 μm). (e, f) Pictures from transversal sections of the spinal cord proximal (rostrally) to the injury zone immunostained for p75. Note the increase in the abundance of Schwann/Schwann-like cells in the dorsal funiculus of bNCSC-treated animals (arrows). Scale bars: 100 μm. (g, h) The increase in Sox10+/p75+ glia in the dorsal funiculus at similar axial levels was accompanied by a reduction in OX42+-activated microglia/macrophages. Scale bars: 100 μm. (i) Glia invading the center of the injury core after bNCSC grafting are S100+/low (empty or filled arrows, respectively). Scale bar: 20 μm. (j) Some of the Sox2−/Sox10+ glia express RIP at low levels (arrows) and few of them are associated with PGP9.5+ axons
Schwannosis is a very frequent feature after contusion, transection/hemisection or photochemical insult of the spinal cord, and it is characterized by the invasion and growth of peripheral nerve elements, including axons and Schwann cells [55, 56]. In such injuries, in the long-term, CNS axons are mainly remyelinated by Schwann cells, while oligodendrocytes remain largely unable to do it in the absence of astrocytes [57, 58]. It has been shown that Schwann cells can myelinate CNS axons only when they are demyelinated or growing [59]. Moreover, p75+ Schwann cells, which can interact and ensheath axons in a 1:1 relationship, have been observed filling the epicentre of the injury after grafting of olfactory ensheathing cells, exogenous Schwann cells, and bone marrow stromal cells [47, 56]. In such cases, central axons were found to be partially enwrapped by P0+ myelin (Fig. 11.2). Interestingly, in a weight-drop injury experimental model in rat and after grafting of exogenous bNCSC-derived Schwann cells, endogenous Schwann cells were found to invade the epicenter of the injury in its anterior border and then to grow and migrate toward the posterior stump of the injury core, concomitantly to regenerating axons (47, 56; Fig. 11.2). Moreover, recruitment of endogenous p75+/ Sox10+/ Sox2−/ S100+/low Schwann-like cells toward the dorsal funiculus rostrally to, and within the injury core was enhanced in animals treated with bNCSC-Schwann cells when compared to vehicle-controls, and this was found to be associated with a reduction in OX42+-activated macrophages and with a less atrophic dorsal funiculus (Fig. 11.2). These cells were found to increase in numbers over time, thus being able to reach the distal stump by 2 weeks after bNCSC-Schwann cells grafting. By then, few Sox2+/Sox10− astrocyte-like cells appear in association with p75+ cells at the middle of the injury core. Finally, few neurofilament (NF)+ / PGP9.5+ axons were found to be in contact with p75+ while most of them lack PGP9.5 expression, which is probably a sign that only few peripheral axons invade the spinal cord or that most of axons belong to cortical motorneurons which have regenerated though the injury core in association with Schwann-like cells.
Whether or not some of these endogenous Schwann cells could have originated from CNS progenitors at the ependymal layer or from OPCs in the context of traumatic injuries remains to be addressed. In this regard, Barnabé-Heider and collaborators performed lineage tracing studies using different transgenic mice, identifying contribution of cells to the injury site originated from the ependymal layer (Foxj1-CreER mice), astrocytes (Cx30-CreER mice) or OPCs (Olig2-CreER mice) [60]. However, since the authors removed part of the dorsal funiculus without affecting root entry/exit zones (thus avoiding formation of cystic cavities) and they used markers which could be also expressed in CNS-derived Schwann-like cells, such as NG2, new studies are required to address the extent of Schwannosis vs CNS origin of glial subtypes, including Schwann-like cells, repopulating injured spinal cord areas in a more common scenario.
Kaneko and collaborators have shown that long-term inhibition of semaphorin3A (Sema3A) was able to enhance spinal cord regeneration after complete spinal cord transection at the Th8 lamina level in rat [61]. The Sema3A inhibitor used, SM-216289, was found to enhance axonal regeneration (mainly of neuropilin-1+ axons, including serotoninergic raphe–spinal tract ones) and to reduce cavity volume in the injured spinal cord. Interestingly, most of the regenerated axons in the experimental group were remyelinated by cells with peripheral-type properties, i.e., presence of Schwann cells, perineuria, and basal lamina, a feature rarely seen in control animals. Thus, and with support from in-vitro assays, the authors concluded that this treatment was able to enhance Schwann cell migration all through the spinal cord injury zone. Thus, the invasive behavior of Schwann cells and/or Schwann cell precursors (SCPs; [62]), as a natural common phenomenon after spinal cord injury, might probably play a significant role for future therapeutic strategies with the aim of enhancing CNS regeneration. Nevertheless, undesired side-effects of promoting such processes, such as the formation of Schwannomas or the possible development of neuropathic pain, would require further analyses [56].
The Multipotency of Schwann Cell Precursors
One of the latest discoveries in the neural crest research field, the unexpected multipotency and high plasticity of the so-called SCPs, has greatly increased our knowledge on developmental biology and opened new ways for regenerative medicine. SCPs are the first stage in Schwann cell differentiation lineage of some of the postmigratory NCCs. They are found in association with growing axons, which correspond to recently born peripheral sensory neurons and spinal cord motorneurons, and at their growth cone tip [63]. They are characterized by novel expression of proteolipid protein (PLP), brain fatty acid-binding protein (BFABP), P0, and Cadherin-19, among other markers. Interestingly, such postmigratory committed neural crest cells coexpressing P0 and PMP22 obtained for instance from the dorsal root ganglia were found to be able to differentiate in vitro into neurons and glia [64]. SCPs and satellite glial cells (another peripheral glia subtype, found in association with peripheral neuronal soma in ganglia), from postmigratory neural crest cells or from boundary cap neural crest cells (localized at the neural tube-nerve root entry/exit zone), would probably differ from each other in the factors specifying them [65].
By performing mouse genetic lineage-tracing experiments as well as nerve ablation and in vivo chicken electroporation studies, Adameyko and collaborators showed that SCPs are also a source of a significant proportion of skin melanocytes [66]. Interestingly, shortly after SCP detachment from growing axon tips and if they do not contact neuronal projections shortly after again, they would start expressing MITF and undergo melanocytic differentiation (Fig. 11.1). Thus, in the absence of axonal NRG1 signals (required for subsequent steps of Schwann cell differentiation, [67] SCPs become melanoblasts, provided that they find sufficient levels of survival growth factors [such as insulin growth factor-like 1 (IGF-1), platelet-derived growth factor, PDGF, or hepatocyte growth factor, HGF]. In the same article, the authors wonder themselves whether after injury myelinating Schwann cells might regain the capacity to undergo dedifferentiation and subsequently generate pigment cells in the absence of NRG1. With this aim, they performed right sciatic nerve axotomy on Krox20-Cre-YFP mice, with ligation of the proximal stump nerve end in order to avoid regeneration. They also dissected out a 0.5 cm fragment distally to the axotomy and sutured it to the underlying muscle. Two months later, animals were sacrificed, and many YFP+ were seen in the nerve fragment and in the dermis of the ipsilateral experimental animals flank, thus confirming their hypothesis.
A few years later, two independent groups reported that parasympathetic neurons (which constitute one of the two divisions of the autonomic nervous system) originate from FOXD3+/ p75NTR+/ ErbB3+/ Cadherin-19+/ PLP+/ Phox2B+ SCPs [68, 69]. And even more recently, SCPs recruited to the gut through pelvic nerve innervation were shown to be the source of postnatally born neurons of the enteric nervous system [70]. Most of these SCP-derived neurons are calretinin+ and are present in both the myenteric and submucosal ganglia of the large intestine. Moreover, SCP-specific Ret ablation causes oligoganglionosis of the terminal region of the colon, which might require higher levels of Ret expression for their survival and development. Such results likely suggest a role for SCPs in Hirschsprung disease-related disorders.
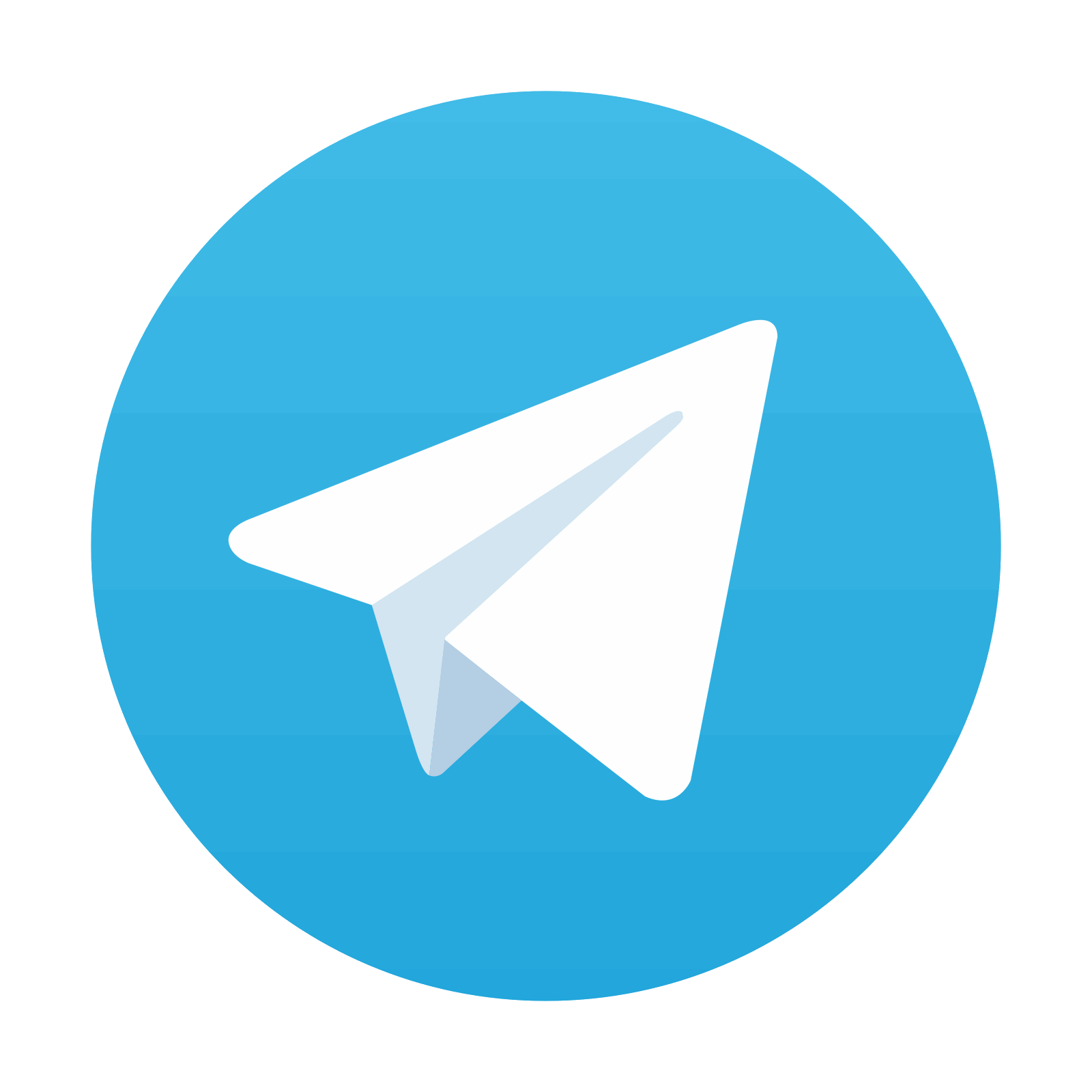
Stay updated, free articles. Join our Telegram channel

Full access? Get Clinical Tree
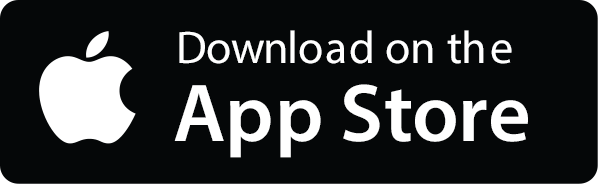
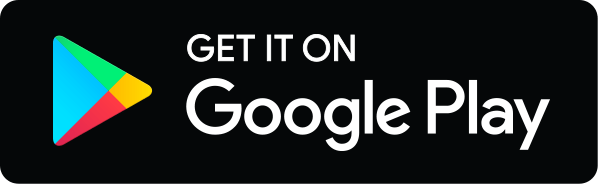