Poul Anderson (1926-2001)
28.1 Introduction
Intracranial hypertension (ICH) is still the leading cause of death in patients with acute brain damage and especially in those with severe traumatic brain injury (TBI) (Glasgow Coma Scale [GCS] score ≤8).
The normal range of intracranial pressure (ICP) measured in any compartment (intraventricular, in the brain parenchyma, subdural space, etc.) does not exceed 10-12 mmHg in the supine position. However, high ICP or intracranial hypertension (ICH) is defined when the absolute values of ICP exceed 20 mmHg during a defined period of time (5-10 minutes) and all those causes that can increase it have been ruled out (poor patient adaptation to the ventilator, inadequate sedation or analgesia, postural changes, nadequate head and neck position, high blood pressure, hyponatremia, fever, etc.). However, this threshold should always be considered relative, and it can vary depending on the etiological factors responsible for ICH, the location of the injury, and other circumstances such as when the skull is partially open in patients with decompressive craniectomy or semiclosed as in infants and children. There is now widespread agreement, endorsed by the Clinical Practice Guidelines of the Brain Trauma Foundation (BTF), that the threshold of 20 mmHg is acceptable, with a level II recommendation to initiate therapeutic measures [1]. However, ICP values should be individualized for each patient and for each clinical situation in particular [2].
Traditionally, it was claimed that between 50-75% of patients with severe TBI developed ICH during the first week after injury. This incidence has been verified in nearly all studies since the publication of the results of the Traumatic Coma Data Bank (TCDB) published in 1991 [3,4]. In this study, 72% of patients had an episode of ICH (>20 mmHg) during their stay in intensive care [3]. Since then, we know that in the natural evolution of patients with severe TBI the risk of ICH is highly variable and will depend on the type of injury according to the classification proposed by Marshall et al. [5]. However, the analysis of recent multicentre studies questions this widely-accepted premise. The results of a phase III controlled study to demonstrate the neuroprotective efficacy of dexanabinol (synthetic cannabinoid), showed that in both the treated and the control group the percentage of time in which ICP remained above the 25 mmHg threshold was only 10% [6].
The results of this study are striking and may be explained by the following reasons: 1)the 861 patients included were treated in highly specialized neurotrauma centres with a standardized and homogeneous medical and surgical treatment; 2) half of the patients (48% in the placebo group and 49% in those treated with dexanabinol) had type II lesions on the Marshall classification while in the TCDB study only 24% of the patients were included in this category, and 3) in most participating centres the Brain Trauma Foundation recommendations were followed (to evacuate the space-occupying lesions with a volume >25 cc).
In our opinion, the significant reduction of ICH reported in this study was mainly due to an early management of systemic complications and a more proactive behaviour in the evacuation of focal lesions with a relevant volume in most of the participating centres.
28.2 Etiology of Intracranial Hypertension
To better understand the implementation of the second level measures, it is useful to recall the traumatic lesion classification introduced by Gennarelli et al. in the 1980s [7-9]. Pragmatically, these authors differentiated between two types of lesions: focal and diffuse.
The inclusion of a patient in one of these two groups is determined from the findings observed in the computed tomography (CT). This classification, although apparently simple, allows to differentiate groups of patients with different mechanisms of injury, clinical presentation and outcome. Focal lesions (cerebral contusions, lacerations and hematoma) cause neurological deficits due to tissue destruction and perilesional ischemia and cause coma when they reach a size big enough to cause brain herniation and secondary compression of the brainstem (Figure 28.1).
Figure 28.1. CT scan of a 19-year-old woman who underwent evacuation of an acute left subdural hematoma. In the immediate postoperative period she developed a contralateral anisocoria with a mydriatic right pupil. A new control CT scan showed the appearance of an extensive right epidural hematoma which caused severe brain herniations (subfalcial, uncal and tonsillar), with the complete absence of the basal cisterns. Although the latter hematoma was also evacuated, the patient remained in a vegetative state for several months after injury. She then regained a minimally conscious state; 3 years after trauma, she remained in a state of severe disability, with total dependence for activities of daily living and very limited communication.
Diffuse injuries are those that do not occupy a well-defined volume within the intracranial compartment (axonal injury, brain swelling, etc.). This latter group of patients is clinically and neuroradiologically heterogeneous, and in most cases coma is a consequence of the diffuse involvement of axons in the cerebral hemispheres and deafferentation of subcortical structures and the brainstem. In these cases, anatomopathological study fairly consistently demonstrates diffuse axonal injury of variable severity [10].
28.3 Intracranial Pressure Monitoring
The only reliable way to confirm whether or not a patient has ICH is with the continuous monitoring of ICP. Estimates from neuroradiological signs have a high margin of error. Continuous ICP monitoring has become routine in the management of severe TBI since the publication of the Brain Trauma Foundation’s Clinical Practice Guidelines for the management of severe TBI in adults in 1995 [11]. These Guidelines have been updated and endorsed by most international scientific societies. The latest version, published in 2007, reaffirms with level II evidence, that ICP monitoring should be conducted in all patients with severe TBI who have an abnormal CT scan on admission [1]. However, in recent years we have learned that the absolute value of ICP, although the mainstay in the management of severe TBI, as an isolated parameter is not sufficient for decision making in complex patients. Monitors for recording ICP, signal processing, its value as a diagnostic method and its importance in the clinical management of many patients with acute neurological diseases have been substantially modified over the last two decades. There are two main reasons for this change: first, a much better understanding of the pathophysiological factors that regulate the response of the craniospinal axis in situations of volume increases, and second, advances in the technology available for recording and analyzing the ICP signal and other physiological variables associated with it (cerebral perfusion pressure, cerebral blood flow, etc.).
The introduction in the last two decades of global and regional methods to monitor cerebral metabolism and oxygen supply (jugular bulb oxyhemoglobin saturation, brain tissue pressure of oxygen and microdialysis) has provided valuable information that allows us to better understand the complex pathophysiology of high ICP.
28.4 Clinical Significance of the Pressure-Volume Curve
Often, new technologies let people forget basic pathophysiological fundamentals. In the late 19th century, Monro and Kellie were the first to define the relationship between pressure and volume in the intracranial space. In the neurosurgical arena, Burrows and Cushing introduced their theories, which remain valid today with almost no significant changes.
According to the Monro-Kellie theory, in the adult – in whom the fontanelles and cranial sutures are completely closed – the volume of intracranial space must remain constant due to the physical characteristics of its components. These characteristics are: the incompressibility of the three intracranial components – brain parenchyma, blood and cerebrospinal fluid (CSF) – and the indeformability and inextensibility of the intracranial space in the adult. For these reasons, a volume increase at the expense of any of the three intracranial components or adding a new volume (tumour, hematoma, etc.) must necessarily be accompanied by a simultaneous decrease in one or all of the other components can be distingusihed. The mathematical equation which relates the variables pressure and volume in the intracranial space is not comparable to that of a perfect elastic body, but two distinct components. In a first phase, the volumetric increases do not change the absolute value of ICP, suggesting an intracranial compartment with high compliance (low elastance) [12]. However, when the physiological buffers of the intracranial space (circulating blood volume and CSF, mainly) are depleted, even a minimal increase in volume causes large increases in ICP (low compliance or high elastance phase). The pressure-volume curve is mathematically described by an exponential function and its mathematical description is based on both clinical and experimental studies. The pressure increase is always more pronounced as the patient moves to the right part of the curve. This right part of the curve, which draws an almost vertical segment, is called the space decompensation phase and is associated with a higher risk of neurological deterioration (Figure 28.2).
Figure 28.2. Pressure-volume curve. The horizontal segment of this curve (A) corresponds to the compensation phase. In this phase, the physiological compensatory mechanisms (venous drainage, displacement and increased absorption of CSF, etc.) allow, despite the progressive increase in volume, the maintenance of intracranial pressure (ICP) within the normal limits. As compensatory mechanisms are exhausted, i.e., the more we move to the right of the pressure-volume curve, small increases in volume generate significant increases in ICP. From the point of inflection (B), we enter what is called the decompensation period of the curve or period of high elastance (low compliance) (C). This figure also shows how, as we move to the right the curve, there is a progressive increase in the amplitude of the cardiac component of the ICP. This increase can be used as an indirect information on the intracranial compliance.
The mathematical relationships between pressure and volume allow to assess by means of injecting a known volume in the intracranial space, the patient’s tolerance to the addition of new volumes into the cranial cavity or the elastance of the craniospinal axis. Czosnyka proposed, as a noninvasive method for continuous monitoring of the elastance, the use of a computerized system to assess continuously the Pearson’s correlation coefficient (R) between the amplitude of the arterial wave and the intracranial pressure wave. The periodic increase in the blood volume entering the intracranial space with each cardiac cycle induces a synchronous change in the amplitude of ICP, which allows to estimate the intracranial volume reserve [12].
An R value close to zero between the amplitude of the arterial wave and the amplitude of the cardiac component reflects the lack of synchronization between the pulse wave and ICP and therefore indicates an adequate reserve volume. A high index close to 1 indicates an almost linear transmission of the pulse wave to the intracranial space and therefore a low volume reserve (low compliance or high elastance status) [12].
The volumetric compensatory mechanisms in the intracranial space require a variable period of time to be effective. Therefore, slow increases in volume (brain tumours) are always compensated for much more effectively than the abrupt increases that occur in acute situations, such as in spontaneous intracerebral hematoma or acute hydrocephalus.
28.5 Where to Monitor ICP? The Problem of Intracranial Pressure Gradients
The traditional concept assumes that the intracranial space behaves as a single chamber where the pressure is distributed evenly and is, therefore, identical at all points. The immediate consequence that follows from this premise is that ICP monitoring in any compartment (epidural, subdural, etc.) should reflect similar results both in absolute values and in its evolution. The existence of gradients between the supra- and infratentorial space and between the subarachnoid space of the spinal cord and the posterior fossa has been confirmed in different animal species. However, the existence of gradients between the two cerebral hemispheres has always been questioned. Experimental studies have objectified the presence of significant interhemispheric gradients in space-occupying lesions. However, the few clinical studies on interhemispheric gradients in head-injured patients ended with conflicting conclusions.
Our group studied this subject in 50 patients with moderate or severe TBI in whom ICP was monitored in both cerebral hemispheres [13]. The monitoring results were stratified according to the predominant type of lesion into two groups: focal or diffuse lesions. Within the diffuse injury category, patients were included in whom the focal lesion volume was <25 ml and the midline shif was ≤3 mm. In the focal lesion cathegory we included patients in whom the sum of the lesion volumes of a hemisphere was >25 ml and/or the midline shif was greater than 3 mm.
Our study showed that in 25% of patients with a focal injury there were clinically significant differences in ICP (>5 mmHg) and hence in cerebral perfusion pressure (CPP) calculated in the two hemispheres. However, in none of the patients with a diffuse injury the differences observed were clinically relevant. It is therefore possible to assume that in patients with a diffuse injury the intracranial space behaves as a single unicameral chamber without a compartmentalized space and, therefore, the pressure transducer can be implanted interchangeably in either of the cerebral hemispheres. However, since volumetric increases may occur in initially small focal lesions, it is always advisable to monitor ICP on the side where lesion volume is greater. In focal lesions with or without midline shift, monitoring must always be performed on the side with the greater lesional volume. If ICP is monitored on the side contralateral to the lesion, treatment will be inappropriate and calculation of CPP inaccurate and overestimated.
The clinical management of patients with increased ICP is complex and often differentiated into several stages. The Brain Trauma Foundation Guidelines (1995) proposed a staged protocol wherein a distinction was made between first and second level therapeutic measures. Many clinical protocols have been based on this scheme. In the first Guidelines version, the classes of evidence (Class I, II and III) defined recommendations called standards, guidelines and options. In the latest Guidelines version published in 2007, the same classification of the evidence is followed, but the initial terminology is replaced with the most commonly used in other clinical guidelines: Level I, Level II and Level III [14]. Class I evidence is a result of controlled studies of high methodological quality. Controlled but methodologically questionable studies (systematic biases, significant losses, etc.) may give support to a lower level of evidence (II or III).
Class II evidence usually derives from well-designed prospective or retrospective studies where two or more treatment options are compared. The lowest level of evidence (Class III) corresponds to retrospective studies, case series or expert opinion, among others [14]. In the new 2007 Guidelines, although the standard terms and options are abandoned, its meaning remains.
28.6 Stepped Versus Individualized Treatment
In the 1990s many units, including ours, attempted to use individualized treatment of high ICP based on CT sacn criteria and parameters obtained from the monitoring of hemodynamics and cerebral oxygenation. At present, we believe that this kind of management is not successful, since it is difficult to systematize and is subject to great variability in both its application and its effectiveness. Moreover, the success of these personalized treatments often depends on uncontrollable factors such as the physician’s experience, protocols which are difficult to implement and the availability of complex and not widely-available neuromonitoring methods. Therefore, since the early 2000s, the use of structured and uniform protocols applied systematically to all patients with severe TBI and ICH has become general. These protocols tend to be minor modifications from the recommendations published by the different versions of the BTF Guidelines. There is no need to stress that these protocols should not be rigid, but flexible enough to allow their adaptation to specific clinical situations [15].
28.7 Types of the Therapeutic Measures
The original version of the Guidelines for the clinical management of patients with severe TBI defined three main levels to manage ICP: 1) general measures which should be applied to all patients, with and without ICH, 2) first-level measures for the treatment of high ICP and 3) second-level measures for the management of patients who do not respond to the first-level measures.
General and first-level measures have already been discussed in previous chapters of this book. This chapter will discuss the second-level measures. Note that the 2007 Guidelines present no treatment algorithm, so in this section we have followed the systematics of the initial BTF guidelines release updated in 2000. It is essential to emphasize that evacuation of volumetrically significant (>25 ml) space-occupying lesions or those with a lower volume but located in high-risk anatomic locations, as in the temporal lobe, should be included within the general or first-level measures. When there is a focal lesion, extending medical measures to extremes without evacuating the lesion is the wong way to go. This premise is especially important in the treatment of brain contusions, a field traditionally abandoned by the neurosurgeon and where early surgery allows to obtain excellent results in patients with high GCS scores. Recently Servadei et al. have completed a comprehensive review on the role of surgery in the management of TBI [16].
In all patients with severe TBI, a set of general measures should be initiated early in order to achieve hemodynamic stability, adequate nutritional support, and to avoid any factors that can increase ICP [15]. Among the last are the mismatch between the patient and the ventilator, improper patient position, hypoxia, hypercapnia, fever, seizures, arterial hypo-or hypertension and hyponatremia. Maintaining normovolemia and the proper choice of replacement solutions play a vital role in the management of the neurotrauma patient.
28.7.1 First-level Measures
These are initiated when the ICP values are >20 mmHg. The first version of the BTF Guidelines recommended treating thresholds above 20-25 mmHg, but it should be underlined that this recommendation was based on expert consensus rather than in any strong evidence. In a previous work, we emphasize that, in our opinion, the lower threshold suggested by the Guidelines (20 mmHg) is preferable because in this way treatment is started earlier improving the chances of achieving a correct control of ICP [2]. The last version recommends a threshold of 20 mmHg (level II evidence) although the text mentions the threshold of 20-25 mmHg as proposed in the 1995 version [17] makin this issue misleading. In patients with a bone decompression greatet than 5 cm and with an open duramater, it is recommended to lower the treatment threshold to 15 mmHg [4]. Also, this threshold should be also reduced in patients with focal lesions in one or both temporal lobes.
28.7.2 Second-level Measures
In the first version of the BTF guidelines these measures were defined as treatments which should be initiated when the first level measures (muscle paralysis, hyperosmolar solutions, moderate hyperventilation, etc.) were unable to control ICP below the established thresholds. In the 2007 update, this term and the whole algorithsm of treatment has disappeared. As a result of the collaboration between the Brain Trauma Foundation and a group of methodology experts, the methodology on the latest release has changed significantly compared to the first two editions. However, the lack of a clear proposal as to when and how to apply the various measures has generated some confusion. The latest version lacks a common thread and loses clarity. At the time of writing this chapter, the Brain Trauma Foundation has appointed an expert committee to update the algorithm proposed in the first version of the guidelines that should be upgraded in 2013.
In the earlier editions, the “second-level” measures formed a broad category which included many therapeutic manoeuvres considered strictly first-level or even general manoeuvres such as the use of the Lund therapy or a CPP increase above accepted thresholds (Rosner therapy).
To avoid misunderstandings, we have selected for discussion three therapeutic options:
1) barbiturates, 2) moderate hypothermia and 3) decompressive craniectomy.
These second-level measures should be introduced early after the threshold of 20 mmHg has been passed and under a stepwise treatment protocol. Some authors have suggested modifications in the indication and timing to introduce these measures, using other monitoring parameters such as the CPP, tissue oximetry and/or the jugular bulb oxyhemoglobin saturation. It should be emphasized that there is no evidence that any of these alternatives is superior to the classical thresholds of ICP. In addition, it must be emphasized that high ICP itself is harmful and that brain herniations may occur at relatively low ICP thresholds. Our recommendation is to apply these measures following a set of pre-established rules agreed between neurosurgeons and intensivists. The degree of collaboration between different specialists in the multidisciplinary management of these patients is probably the “non-measurable” and hidden factor which more strongly modifies the outcome of the patient with severe TBI.
Our recommendatios is second-level measures implementation shloud be based on the evolution of the ICP and serial neuroimaging tests. A new CT must always be obtained before instituting any of these measures and to rule out evacuable space-occupying lesions. Once the decission has taken, these measures should be implemented quickly applied in a complementary and not exclusive way. The association of barbiturates and hypothermia has been reported, as has the association of hypothermia and decompressive craniectomy. It is important to empasize that the use of these rescue measures makes sense only if the patient is considered neurologically viable.
28.8 Barbiturates
Barbituric acid was first synthesized in 1864 by von Bayer and is obtained from the condensation of malonic acid with urea [18]. From the chemical substitution of this primordial molecule a series of barbiturates are obtained which have a depressant action on multiple cellular functions in all organs, including the central nervous system (CNS).
The first barbiturates introduced into clinical practice were barbital in 1903 and phenobarbital in 1912 [18]. Since then, numerous derivatives has been introduced in the therapeutic armamentarium as sedatives, hypnotics, anticonvulsants or anesthetics. The most important ones from a neurological point of view are phenobarbital, pentobarbital and thiopental. At high doses, these drugs diffuse across all cell membranes and concentrate preferentially in certain tissues depending on their blood supply and the lipid solubility of the molecule, its affinity for plasma proteins and its degree of ionization [19]. Barbiturates are highly lipid soluble substances with a relatively uniform distribution in the CNS, are metabolized in the liver by oxidation, and are mostly eliminated with the urine [20]. However, there are pharmacokinetic differences depending on the type of barbiturate and its lipid solubility [20]. Moreover, individual factors such as body weight, fat compartment volume, muscle mass and other variables modify their pharmacokinetic characteristics. The main mechanism of action of barbiturates on the CNS is through the GABAergic system, the major inhibitory system in the mammalian CNS, mediated by GABA and its receptors (GABAA and GABAB). Barbiturates block GABAA receptors, a receptor involved in the mechanisms of action of benzodiazepines and substances with convulsive capacity such as picrotoxin [21]. In addition, barbiturates also inhibit AMPA glutamate receptors. This dual mechanism explains the deep depression that these drugs cause on the CNS metabolism.
28.8.1 Effects of Barbiturates on ICP: Recommendations
The effect on ICP of barbiturates was first reported by Horsley in 1937 [22]. However, it was Shapiro et al. in 1973 who first introduced barbiturate coma as treatment for patients with severe TBI and increased ICP [23,24]. In the beginning, this therapy created, as often happens with new treatments, excessive optimism owing to its potential neuroprotective effect. Over time, the use of these drugs has raised significant controversy and has also shown that their use requires considerable pathophysiological knowledge for their precise and individualized application [25]. In the 1995 Guidelines version, barbiturates were covered in a single paragraph [11]. In the last update of 2007, barbiturates are included in a larger section called “Anesthetics, analgesics and sedatives” [26]. In this section, two drugs are considered for the treatment of ICH, barbiturates and propofol. In our view, the inclusion of barbiturates in this broad section blurs the issue, because both analgesia and sedation are part of the general management of severe TBI, while high-dose barbiturates are used only as a second-level measure in the treatment of refractory ICP.
The 2007 Guidelines state that “High-dose barbiturates are recommended (level II evidence) to treat ICH refractory to medical or surgical measures. Hemodynamic stability is essential before and during the administration of barbiturates”.
Propofol is recommended with a level II evidence for ICP control but its efficacy in improving mortality or functional outcomes has not been proved. In addition,
high doses of propofol (>5 mg/kg/h) can cause serious side effects and the so-called “propofol infusion syndrome” [26].
In reviewing the evidence on the use of barbiturates there are only three controlled studies. The first multicentre study on the use of barbiturates in the treatment of TBI was published by Schwartz et al. in 1984 [27]. However, its design left unanswered the most important questions because it compared barbiturates versus mannitol in patients with severe TBI [27].
In a study by Ward et al. pentobarbital was administered prophylactically to a group of patients with intradural hematoma or diffuse lesions and a GCS score ≤5 [28]. In the analysis of the results there were no significant differences in the group assigned to pentobarbital compared with the control group or in the incidence of increased ICP, in the duration of these elevations or in its response to treatment [28]. Only the study published by Eisenberg et al. in 1988 studied the effect of pentobarbital in patients with refractory ICP [29]. In this prospective, multicentre controlled trial, barbiturates were used as a last resort to control refractory ICP. It included 73 patients aged between 15 and 50 years and with GCS score ≤7 [29]. The treatment protocol started early when ICP was above 15 mmHg, using hyperventilation, mannitol, muscle relaxation and other first-level options. When ICP was not controlled according to preset criteria – 25 mmHg in patients without bone defect and 15 mmHg in those with a bone defect >25 cm2 –, patients were randomized to treatment with pentobarbital or continuation with conventional measures. The success or failure of treatment was evaluated by assessing the control of ICP but not the patient’s functional outcome. The findings showed that the probability of controlling ICP in the group treated with pentobarbital was twice that in the control group. This probability remained after controlling for prognostic variables (GCS score, accident-inclusion period, etc.). In those cases without cardiovascular complications before inclusion into the study, the effectiveness of barbiturates was four times higher in controlling ICP than in the control group.
This study is the one which supports the Brain Trauma Foundation recommendation to assign a level II evidence to these drugs. However, this study presents some problems that prevent its analysis. Among others, 11 patients who did not respond to conventional therapy were allowed to cross over. When the “intention to treat” methodology was applied to the analysis of the results, the effect of barbiturates on mortality was lost [26]. In the latest Guidelines version, the results match those of the systematic review conducted by the Cochrane Collaboration that concluded that even though barbiturates may be useful to reduce ICP, their effect is moderate. In Eisenberg’s study the relative risk (RR) for the control of ICP was 0.81 (95% CI, 0.6-1.06)[30]. In addition, there was no evidence that barbiturates improve either mortality or functional outcome and in one out of four patients treated with pentobarbital hypotension was a clinically relevant adverse effect.
28.8.2 Thiopental or Pentobarbital?
The effects of barbiturates on ICP are attributed to their ability to reduce the brain functional metabolism and the consequent coupled reduction of cerebral blood volume (CBV). This CBV reduction causes decreased ICP. An important issue for clinical practice is which barbiturate is preferred to treat refractory high ICP. The most commonly used barbiturates have been pentobarbital in the United States and thiopental in Europe. In a pilot study Pérez-Barcena et al. have suggested that thiopental may be better than pentobarbital [31]. However, because of its design, this study does not allow draw any definitive conclusions. As a general recommedation, barbiturates should be selected based on pharmacokinetic considerations and the lower frequency of their side effects. Although thiopental is more widely used in Europe, pentobarbital theoretically offers some advantages that facilitate its use.
The metabolism and pharmacokinetics of thiopental are more complex than those of pentobarbital [20,32]. Part of thiopental administered parenterally becomes pentobarbital [20,32], making it difficult to control plasma levels and their correlation with therapeutic effects. Thiopental is more lipid soluble than pentobarbital and its action faster, although its half-life is shorter due to rapid redistribution into fat, muscle and other tissues [20,32]. The extracerebral distribution of pentobarbital is lower and its excretion slower, as it is eliminated mainly by metabolism. In obese patients or those with a large volume of adipose tissue, thiopental accumulates more than pentobarbital and after prolonged treatment, thiopental is eliminated more slowly than pentobarbital [20,33]. Moreover, thiopental has a significant depressant effect on the immune system by inhibiting the phagocytic activity of lymphocytes. This effect has not been observed with pentobarbital [20,34].
For these reasons, and in the absence of controlled studies on the subject, our recommendation is to use pentobarbital when treating patients with increased ICP.
28.8.3 Dosage and Controls in Barbiturate Coma
The main problem with the use of barbiturates is that they make the patient completely inaccessible to neurological examination. Continuous ICP monitoring and sequential CTs are the primary methods for controlling the clinical of the patients in induced coma. The last version of the BTF guidelines recommend starting with a loading dose of 10 mg/kg pentobarbital administered over half an hour followed by three consecutive doses of 5 mg/kg/h [26]. The load should be continued with a continuous infusion of 1 mg/kg/hr [26]. The frequently observed hemodynamic instability should be treated with colloids and/or vasoactive drugs (dobutamine, dopamine or phenylephrine). It has been shown, however, that when intravascular volume is properly preserved and drug administration is slow, the hemodynamic effects are significantly reduced [19]. Daily barbituremias should be performed, trying to maintain plasma pentobarbital levels between 35 and 45 µg/ml. It has been shown, however, that there is often a dissociation between the degree of suppression observed on the electroencephalogram (EEG) and plasma barbiturate levels [20]. EEG is the best way to monitor barbiturate coma and the “burst suppression” EEG pattern is the best indicator of the maximum metabolic reduction achievable with barbiturates. The usefulness of the BIS or entropy in monitoring the depth of induced coma is still poorly defined.
28.9 Moderate Hypothermia
Moderate hypothermia, defined as a core temperature of 32-33.9°C [35], has been used sporadically since the 1940s in both experimental models of TBI and in non-controlled clinical studies for its potential neuroprotective efficacy [36,37]. The neuroprotective effects of hypothermia are manifold and some still poorly understood. Traditionally, it is assumed that its effect is closely linked to its ability to reduce cerebral metabolism, especially the basal metabolism. It is clear that brain metabolism, and cerebral oxygen and glucose consumption (CMRO2, CMRgl), are temperature-dependent and that hypothermia reduces both. However, experimental studies show that this effect is only one of the many mechanisms by which hypothermia modulates the abnormal neurochemical cascades triggered by TBI [38,39,40].
In healthy subjects, moderate hypothermia induces a linear reduction of CBF in a temperature range between 18 and 37°C. An interesting phenomenon is that physiological metabolic coupling is preserved intact across this temperature range [41].
28.9.1 Basal Metabolism and Functional Metabolism
To understand the beneficial effects of hypothermia on the CNS, it is important to remember some basic physiological concepts. Basal metabolism of an organism is the basic metabolic requirements below which the body is unable to survive [42]. Howerver, certain animals are able to reduce their metabolism for prolonged periods of time and enter in a self-induced state of prolonged reduction of their metabolism to protect themselves against adverse environmental conditions such as lack of heat, water or oxygen [42].
In the cerberal metabolic rate of oxygen (CMRO2), we must distinguish two compartments: functional metabolism and basal metabolism. Functional metabolism is the fraction of CMRO2 dedicated to maintaining electrical brain function, an the electrical activity reflected in the electroencephalogram (EEG) [43]. Basal metabolism is dedicated almost exclusively to sustain the functions necessary to maintain cell structure [43]. The basal metabolism in mammals is organ-dependent, so that each organ contributes differently to the total basal oxygen consumption. Skeletal muscle, abdominal organs and the brain are the three organs which, in this order, consume most of the basal oxygen. However, when CMRO2 is considered in relation to weight, the brain has the highest basal metabolism of any organ in the body.
Vise, using the comparison with a computer defined functional metabolism simply as the metabolic requirements needed to give support to the “brain software”, while basal metabolism is responsible for maintaining the “hardware” [44]. Michenfelder reported that 50-60% of CMRO2 is dedicated to maintaining the brain’s electrical activity [45,46]. This functional metabolism is the one that decreases when barbiturates are administered at doses sufficient to induce the so-called “burst suppression”. An important fact to consider is that hypothermia is only able to cause an isoelectric EEG when reaching 17 to 18°C core temperature.
28.9.2 Therapeutic Use of Hypothermia
In the early 1990s several researchers reported encouraging results in phase II and III clinical trials in which moderate hypothermia was used—prophylactically or to treat patients with increased ICP— in patients with severe TBI [47-51]. The turning point that rekindled interest in hypothermia was the study of Marion et al. published in 1997 where moderate hypothermia was used prophylactically [50]. The excitement caused by this study was largely due to the substantial media coverage that it received outside the medical field. However, the hopes that moderate hypothermia was able to improve the outcome of patients with severe TBI were aborted prematurely and probably hastily, after reports of the results of two large multicentre trials conducted in the United States and Japan [52-55]. In these two studies, early induced moderate hypothermia was not effective in improving mortality and functional outcome of patients with severe TBI. The two studies were questioned for various reasons but we will not discuss them in detail in this chapter. However, it must be emphasized that these results put a moratorium on further research into this powerful but complex treatment. A few years later, a controlled single-centre study published by Zhi et al. involving 396 patients showed that hypothermia was able to significantly reduce mortality and increase the percentage of patients with a favourable neurological outcome [56]. Due to the uncertainty of its effectiveness, the complexity of its use and its side effects, hypothermia has been virtually abandoned in recent years in the management of patients with TBI. However, in the opinion of many, hypothermia deserves a second chance in the management of neurotrauma patients. At present, a European multicentre study is being designed, aimed at demonstrating the effectiveness of moderate hypothermia in patients with severe TBI.
In a recent systematic review, Sydenham et al. [57] found 22 randomized clinical trials on hypothermia in severe TBI. These studies included 1409 patients. The study showed that mortality was significantly lower in the group treated with hypothermia than in the control group (odds ratio [OR] = 0.76, 95%CI, 0.60-0.97).However, the eight studies considered methodologically the most robust showed no significant differences in mortality (OR 0.96, 95%CI, 0.68-1.35) or in the probability of a favourable functional outcome [57]. The review also reported a greater tendency to pneumonia, but with a narrow odds ratio and a wide confidence interval (OR 1.06, 95%CI, 0.38-2.97).
Recently, Sahuquillo et al. in a pilot study have demonstrated the feasibility, speed and effectiveness of this measure, induced by intravascular cooling in reducing increased ICP refractory to first-level therapeutic measures [58]. These results are consistent with those of other authors who demonstrated in open and non-controlled studies that hypothermia is effective in reducing ICP.
In addition, the results of the six systematic reviews carried out to date do not allow to definitively exclude the neuroprotective efficacy of prophylactic hypothermia. For this reason, moderate hypothermia can be considered a reasonable alternative to barbiturates in a selected group of patients when first-level measures have failed to control ICP. However, we recommend that moderate hypothermia be used only in centres with experience in its management and a sufficient number of patients to maintain a minimum level of expertise, without which the complications of moderate hypothermia can mask its clinical utility [58].
Traditionally, methods to induce moderate hypothermia in patients with severe TBI were surface methods (by convection or conduction) associated or not with adjuvant measures such as gastric lavage with cold saline, infusion of cold intravenous fluids, ice applied to the skin surface, and so on. The main disadvantage of these methods is that they require skilled nursing staff to reach the target temperature within a reasonable time, which is almost always more than 6-8 hours. On the other hand, after reaching the target temperature with these methods, the stability of the core temperature is highly variable and can range from 0.5 to 1.5°C in either direction. These temperature variations result in a suboptimal temperature management. Another additional disadvantage of the surface methods is the significant workload it posses to nurses.
Moderate hypothermia may have some complications which can be grouped into four broad categories [59]:
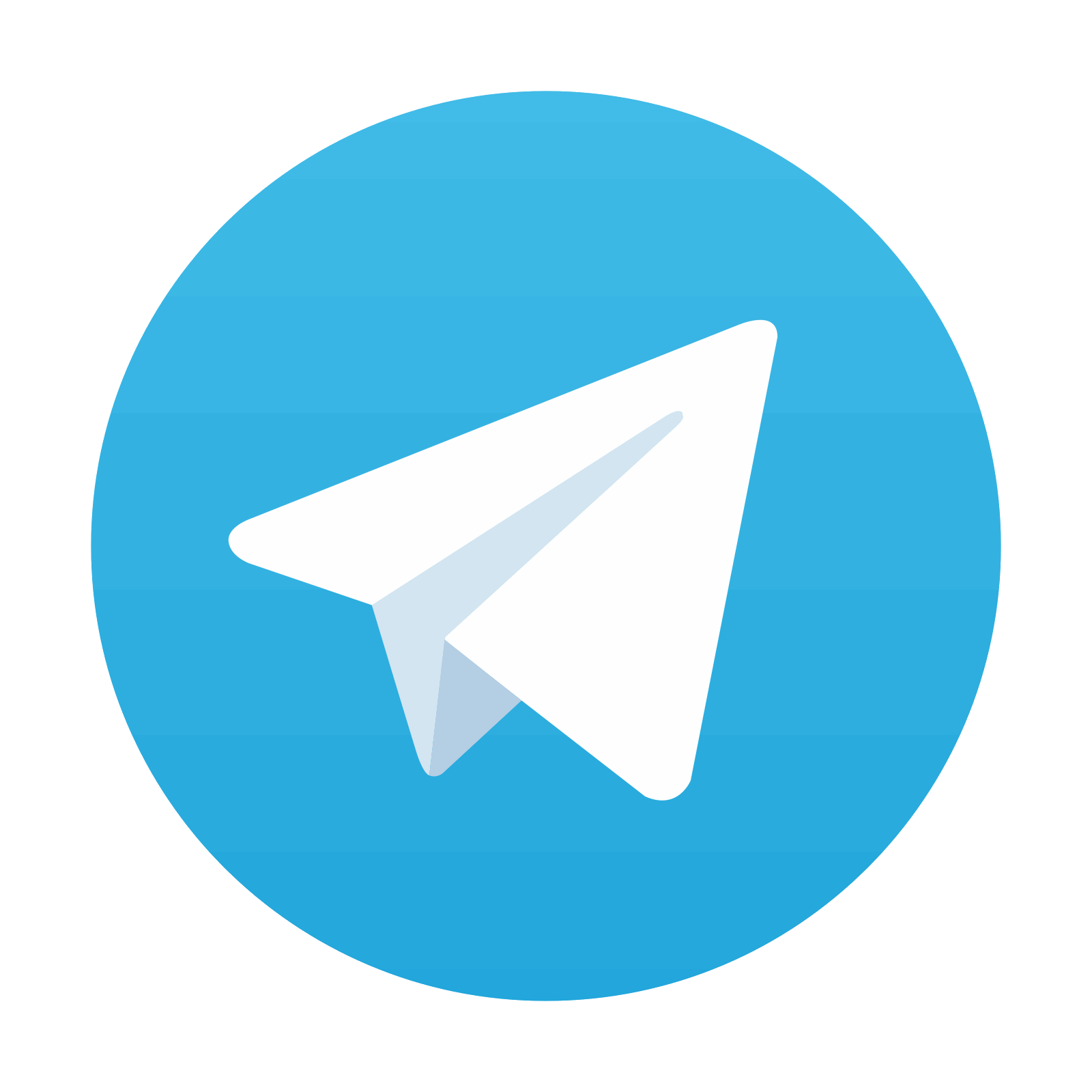
Stay updated, free articles. Join our Telegram channel

Full access? Get Clinical Tree
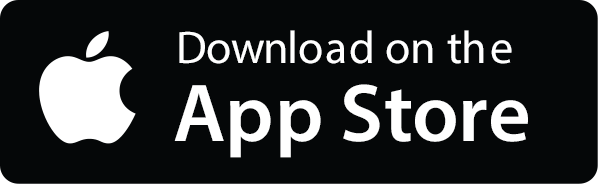
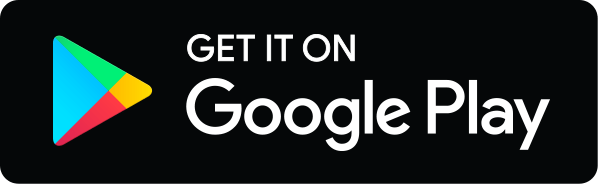