Chapter 30 Sensory and Motor Processing during Sleep and Wakefulness
Abstract
This chapter is divided in three parts: pathways and mechanisms of sensory processes, especially those related to pain; the integration of sensory and motor processes; and finally, respiratory reflexes. Each part considers these functions during sleep and wakefulness because our main objective is to provide an overview of how changes in sensory and motor processes and their integration during sleep contribute to both normal and abnormal physiology. We also discuss the mechanisms by which sleep influences the central processing of nociceptive information and how pain itself may affect sleep. Although all sensory and motor systems are affected by sleep, we primarily focus on how sleep impacts the sensorimotor processes of spinal reflexes and the respiratory responses to chemical (e.g., CO2) and mechanical stimuli (e.g., airway pressure). We focus on these physiological systems because it is important for the clinician to realize that sleep-related changes in sensory-motor processing and their integration have a direct impact on human health and disease and can underlie common and serious sleep disorders. (see Chapter 124 for fibromyalgia; Chapter 126 for pain; Section 13 for sleep-disordered breathing; and, for movement disorders during sleep [e.g., PLMD and bruxism] see Chapters 90 and 99).
Modulation of Sensory Processes During Sleep and Wakefulness
Sensory Pathways and Mechanisms
The body’s peripheral tissues (e.g., skin, mucosa, muscles, and joints) are supplied by small-, medium-, and large-diameter primary afferent nerve fibers. The medium- and large-diameter afferents are myelinated and comprise Aβ and Aδ afferents, most of which terminate in the tissues as sense organs (receptors) detecting tactile or proprioceptive stimuli applied to the tissues. However, some of the Aδ afferents, along with unmyelinated C-fiber afferents, terminate in the tissues as free nerve endings, many of which act as nociceptors—that is, they are the sense organs that respond to noxious stimulation of the peripheral tissues; other Aδ or C-fiber afferent terminals function as thermoreceptors responding to warming or cooling stimuli. The activation of nociceptors may result in the elicitation of action potentials in the Aδ or C-fiber afferents, and these action potentials are conducted along the afferents into the CNS and thereby provide the brain with sensory-discriminative information about the location, quality, intensity, and duration of the noxious stimulus.1–4
In some cases, a prolonged increase in excitability of the nociceptors may occur after tissue injury or inflammation, to the stage where they become more responsive to noxious stimulation or even start responding to stimuli that normally are innocuous. Thus, this process of “peripheral sensitization” may contribute to the hyperalgesia (increased sensitivity to a stimulus that is normally painful) and allodynia (pain resulting from a stimulus that does not normally provoke pain) that occur in certain pain conditions. Numerous chemical mediators are involved in peripheral sensitization of nociceptive endings as well as in their activation by noxious stimuli, including several that also have actions in the CNS (e.g., glutamate, serotonin [5-HT], noradrenaline).5–7
The primary afferents in spinal nerves supplying skin, viscera, and musculoskeletal tissues of the limbs, trunk, and neck project to the spinal cord and synapse on second-order neurons that are predominantly located in the spinal dorsal horn and the dorsal column nuclei.3,4 The afferents innervating orofacial tissues project mainly to the trigeminal brainstem sensory nuclear complex (V-BSNC). This structure is subdivided into the main or principal sensory nucleus and the spinal tract nucleus, which from rostral to caudal comprises three subnuclei known as oralis, interpolaris, and caudalis.2,8,9 The subnucleus caudalis has many anatomical and physiological features that are analogous to those of the spinal dorsal horn.8 Another brainstem region receiving primary afferent inputs is the solitary tract nucleus (NTS); it contains second-order neurons on which respiratory and alimentary tract afferents, taste afferents, and cardiovascular and chemoreceptor and baroreceptor afferents synapse, and plays an important role in autonomic (e.g., respiratory, cardiovascular) functions, taste, and reflexes evoked from the respiratory and alimentary tracts (e.g., cough, swallow).
The spinal Aδ and Aβ primary afferents conducting actions potentials evoked by tactile or proprioceptive stimuli terminate principally in the spinal dorsal horn and dorsal column nuclei, where they may activate second-order nonnociceptive neurons (e.g., low-threshold mechanoreceptive [LTM] neurons). Analogous trigeminal afferents terminate at all levels of the V-BSNC. The Aδ- and C-fiber primary afferents carrying thermoreceptive or nociceptive information predominantly terminate in the spinal dorsal horn (Fig. 30-1) and subnucleus caudalis (Fig. 30-2). The main excitatory neurotransmitters or neuromodulators released from the central terminals of the nociceptive afferents are glutamate and substance P.4,8 In addition to LTM and thermoreceptive neurons, there are nociceptive neurons that are of two main types: nociceptive-specific (NS) neurons that are excited only by noxious stimuli (e.g., pinch, heat) applied to a localized receptive field in peripheral tissues (e.g., skin), and wide dynamic range (WDR) neurons that are excited by nonnoxious (e.g., tactile) stimuli as well as by noxious stimuli.
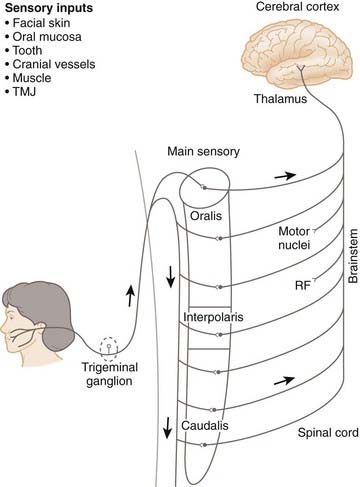
Figure 30-2 Major somatosensory pathway from the face and mouth.
(From Sessle BJ. Acute and chronic craniofacial pain: brainstem mechanisms of nociceptive transmission and neuroplasticity, and their clinical correlates. Crit Rev Oral Biol Med 2000;11:57-91.)
Neuroplastic changes can be manifested in caudalis and spinal dorsal horn (and thalamocortical) nociceptive neurons as a result of nociceptive afferent inputs evoked by injury or inflammation.4,8 This neuroplasticity results from the release from the nociceptive afferent terminals of neurochemicals, such as glutamate, that act via N-methyl-D-aspartate receptor mechanisms to induce a cascade of intracellular events in the nociceptive neurons. These events can result in an increase in neuronal excitability, reflecting what has been termed a “central sensitization” of the nociceptive neurons. The neuroplastic alterations in the nociceptive neuronal properties, and in the reflex neuromuscular changes and other behavioral activities that may be induced by injury or inflammation, represent mechanisms that along with peripheral sensitization (discussed previously) can explain the allodynia and hyperalgesia as well as pain spread and referral that characterize several pain conditions.
Some neurons in the spinal dorsal horn, dorsal column nuclei, solitary tract nucleus, and the V-BSNC give rise to axons that ramify within that structure and serve to modulate the activity of other neurons. Nevertheless, many neurons in these various structures project to other spinal cord or brainstem regions including the reticular formation, raphe nuclei, and spinal ventral horn or cranial nerve motor nuclei. Such connections provide the central circuitry underlying autonomic and muscle reflex responses to peripheral stimuli or provide sensory inputs to neurons in some of these structures that contribute to descending modulatory systems (discussed hereafter). In view of the role that the raphe and reticular formation areas play in wakefulness and sleep, projections to these areas also may provide neural substrates by which noxious stimulation may influence sleep and consciousness.10
Many neurons in the spinal dorsal horn, dorsal column nuclei, NTS, and V-BSNC also (or instead) project to the contralateral thalamus (see Figs. 30-1 and 30-2), either directly or via multisynaptic paths. The main regions of the thalamus that receive and relay this sensory information are the ventrobasal complex (or ventroposterior nucleus in the primate) as well as the medial thalamus and the posterior nuclear group.3,11,12 These thalamic regions contain LTM and thermoreceptive neurons that project to the overlying somatosensory cerebral cortex, where their relayed signals are processed by similar neurons to provide for the detection and localization of tactile and nonnoxious thermal stimuli. In addition, NS and WDR neurons occur in these thalamic regions and most have spatiotemporal coding properties and connections to NS and WDR neurons in the overlying somatosensory cortex that indicate a role for them in defining the spatiotemporal features of peripheral stimuli, and thus in the sensory-discriminative dimension of pain. In contrast, the spatiotemporal coding properties of most nociceptive neurons in the medial thalamic nuclei and posterior nuclear group and their connections to areas such as the anterior cingulate cortex are more suggestive of a role in the motivational or affective dimensions of pain. These features are generally consistent with brain imaging findings in humans that noxious stimuli activate several cortical regions, including the somatosensory cortex and the anterior cingulate cortex.13,14
Modulation of Sensory Processes Including Those Related to Pain
In addition to its role in transferring to the cortex neural signals used for sensory, affective, and motivational functions, the thalamus is also the site of modulatory influences involved in the state of consciousness. The thalamocortical transfer of sensory information is subject to modulation or “gating” as a result of facilitatory and inhibitory processes exerted by local neural circuits or inputs to the thalamus and cortex from other CNS regions such as the reticular formation.15–17 These gating process is especially apparent during changes in behavioral state and consciousness such as during NREM sleep, a period characterized by a marked attenuation during NREM sleep so that sleep continuity is not disrupted.
In the case of nociceptive transmission, the variety of inputs and interconnections in spinal dorsal horn and subnucleus caudalis noted earlier provide the basis for considerable interaction between the various afferent inputs derived from peripheral tissues (e.g., so-called segmental or afferent inhibition) or from intrinsic brain regions (e.g., descending inhibition). Examples include the interneuronal system within the substantia gelatinosa of the spinal dorsal horn and subnucleus caudalis, and the descending inputs to these structures from the periaqueductal gray/raphe nuclei, reticular formation, cerebral cortex and several other brain centers (see Fig. 30-1). Several neurochemicals, including GABA, 5-HT, noradrenaline, dopamine, hypocretin, cholecystokinin, prolactin, melatonin, and opioids (e.g., enkephalins), provide a neurochemical substrate by which many of the afferent and descending inputs can exert their modulatory actions on nociceptive transmission; it is notable that many of these neurochemicals and descending influences also are involved in sleep mechanisms.15,16 Inhibitory influences exerted by many of these inputs on nociceptive neurons have been implicated as intrinsic mechanisms contributing to the analgesic effects of several procedures used to control pain, including deep brain stimulation, acupuncture, and opiate-related (e.g., morphine) and 5-HT agonist (e.g., amitriptyline) drugs. Some, instead, have a role in facilitating nociceptive transmission, for example, in the central sensitization process noted earlier.4,8
Processing Related to Pain during Sleep and Wakefulness
As noted previously, sensory transmission through the spinal cord and brainstem, as well as thalamus and cerebral cortex, may be modulated during sleep and thereby may contribute, for example, to the decreased responsiveness to external stimuli during NREM sleep to favor sleep continuity. The modulatory influences on nociceptive neurons of behavioral factors, including state of alertness, attention, and distraction, are just some examples where the higher brain centers involved in these states give rise to descending influences operating at these levels and thereby contribute to the influence of these behavioral factors on pain. Unfortunately, although much has been learned independently about pain and sleep, there has been only limited investigation of how sleep affects pain and vice versa (see Chapter 126).
There is evidence that sensory processing, including that related to pain, is reduced in sleep states compared to wakefulness, but the underlying processes are still unclear. Nonetheless, with the advent of studies in chronic (awake and sleeping) animal preparations as well as more conventional anesthetized preparations, as well as some correlated studies in humans, it has been shown that somatosensory transmission through several ascending pathways originating in the spinal dorsal horn is tonically diminished during REM sleep; nonetheless, the gating process is quite complex during REM sleep because modulation of somatosensory transmission may be different between phasic and tonic REM sleep.18 There is also evidence of complex gating during NREM sleep, including findings that sensory transmission through some ascending tracts may be attenuated in some stages of NREM sleep and that this is reflected in reduced thalamic and cortical activity in these stages.16–20 Recent studies also suggest that the modulation of spinal sensory transmission during REM sleep appears to involve presynaptic inhibition as well as postsynaptic inhibitory mechanisms, thus supporting earlier findings that presynaptic regulatory processes are important in REM sleep and contribute to the reduction in motor activity during this sleep phase (discussed hereafter). There is evidence that the presynaptic inhibitory processes in the spinal cord utilize GABA, and the postsynaptic inhibition utilizes glycine. Several other neurochemicals released from neurons in higher brain centers have also been implicated in the sleep-dependent modulation of spinal cord neurons or their thalamic targets; these include 5-HT, noradrenaline, acetylcholine, and hypocretin.16,18–20
A complex gating mechanism has also been documented in the rostral components of the V-BSNC that is state dependent, for example, the activity of many rostral V-BSNC neurons and their responses to sensory inputs, including those evoked by noxious (tooth pulp) stimuli, may be presynaptically and postsynaptically inhibited during REM sleep through respectively GABA and glycine-based modulatory processes.16,18–20 However, comparable investigations have not been made in more caudal V-BSNC nociceptive (e.g., caudalis) neurons, which as noted previously have many features analogous to those of spinal dorsal horn nociceptive neurons and play crucial roles in trigeminal nociceptive transmission; thus, if and how sleep stages affect their properties is an important field requiring future study. It should also be noted that the state-dependent processes on spinal and V-BSNC nociceptive transmission that have been revealed have only been studied in acute sleep models, and it is unclear what neural modulatory processes are involved in the more clinically challenging sleep–pain interactions that may occur in chronic pain conditions (discussed later). Furthermore, if nociceptive afferents inputs do reach cortical levels and result in the conscious feeling of pain, then sleep may be disrupted to alert the individual of the noxious event.
Clinical Correlates
When healthy subjects are sleeping in conditions favoring good sleep quality (e.g., a quiet, comfortable environment), low-intensity stimuli may have little or no effect on sleep quality, whereas a loud noise or a sudden pain attack during sleep can produce awakening that might trigger anxiety or concern that impedes subsequent sleep21 (see Chapter 126). Nonetheless, there are several other factors in addition to pain that can influence sleep quality (e.g., past experiences, psychological variables, anxiety, mood, life style, health status, and any concomitant pain–sleep interactions).
In the case of pain patients, they may experience long delays in falling asleep, as well as sleep-stage shifts, frequent sleep arousals, and undesirable body movements; furthermore, some analgesic drugs (e.g., opioids) may affect sleep patterns (see Chapter 126). Although the majority of patients with chronic pain report that pain occurred before or at the onset of poor sleep, suggesting that pain may have a direct effect on sleep quality, studies using experimental noxious stimuli have revealed only minor sleep disturbance in healthy subjects.22,23 However, these studies have elicited acute pain, and it remains unclear the mechanisms by which chronic pain may negatively influence sleep quality. Nonetheless, as noted earlier, chronic pain can be associated with neuroplastic changes in spinal dorsal horn, V-BSNC, and thalamocortical relays, so there is potential for these or other pain-related changes to have effects on sleep; however, how these changes influence the brainstem and thalamocortical circuits involved in sleep is largely unknown.16
The reverse situation, that poor sleep might be a significant cause of pain, is also unclear. There are reports that experimental sleep deprivation or fragmentation can lead to pain, and that restoration of sleep quality (continuity and duration) can reduce this pain in humans. Likewise in animals, sleep limitation leads to a reduction in nociceptive threshold during wakefulness that is reversed once sleep is restored. However, some studies suggest that other factors (e.g., fatigue, mood changes, cognitive impairment) may be involved24 and thereby raise doubts whether poor sleep is indeed a dominant or a pathognomonic cause of pain. Thus, at present, there is a need to further investigate the relationship between chronic pain and poor sleep quality.
Modulation of Sensorimotor Processes During Sleep and Wakefulness
Sleep mechanisms markedly affect sensorimotor functions. Sleep not only suppresses basal muscle tone, but it also attenuates, and in some cases even abolishes motor reflexes (see Chapter 31). Sensory and motor processes and their integration are also differentially affected by prevailing behavioral states. For example, during wakefulness, chemical and mechanical stimulation of laryngeal tissues evokes coughing; however, during sleep the same stimulus only elicits a brief expiration. Such observations not only suggest that sensorimotor processes are suppressed by sleep, but that sleep mechanisms themselves control the integration and expression of sensorimotor processes.
Sensorimotor Pathways and Mechanisms
The afferent inputs that access the spinal cord, brainstem, thalamus, and cerebral cortex are involved not only in perceptual processes but also are involved in sensorimotor integration and control.1,9,25,26 The neuromuscular system is reflexively influenced by receptors that signal pain, touch, joint position, muscle stretch, or tension. In the case of muscles of the trunk, neck, and limbs, afferents from spinally innervated tissues enter the dorsal root and project into the spinal cord where they can excite or inhibit α-motoneurons that innervate skeletal muscles. The α-motoneurons that innervate craniofacial muscles (e.g., jaw, soft palate, laryngeal and tongue) reside in motoneuron pools in the brainstem, most notably the trigeminal, facial, ambiguus, and hypoglossal nuclei.
One source of afferent input that reflexively influences α-motoneurons is derived from the muscle spindle, a stretch-sensitive receptor signaling muscle length. The group Ia primary afferents innervating muscle spindles monosynaptically excite α-motoneurons to produce contraction of the stretched muscle. This monosynaptic circuitry is the neural substrate for the H-reflex and jaw-closing reflex. Muscle spindle afferents are a fundamental motor control mechanism in limb, neck, and trunk muscles; however, because there are insignificant numbers of muscle afferents in most craniofacial muscles (e.g., anterior digastric, facial, pharyngolaryngeal muscles), other receptor systems, for example, receptors in tooth-supporting tissues and in the pharyngeal and laryngeal mucosa, play a significant role in the control of these muscles.1,9,25
Some afferent inputs trigger either excitatory or inhibitory reflexes via affecting the activity of interneurons, which are located in the spinal dorsal horn, V-BSNC, NTS, or within motor pools themselves. Both interneurons and motoneurons are also modulated by the descending neural systems that regulate somatosensory transmission such as the reticular formation, limbic system, lateral hypothalamus, basal ganglia, LC, red nucleus, cerebellum, and sensorimotor cerebral cortex.25,26 Some of these systems are also involved in the initiation of movements (e.g., the primary motor cortex), others in the control and guidance of movements and their integration with other sensorimotor functions (e.g., basal ganglia; spinal or brainstem pattern generators for locomotion, chewing, swallowing) and yet others in the regulation of sleep–wake states themselves (e.g., periaqueductal gray, lateral pontine tegmentum and lateral hypothalamus).27
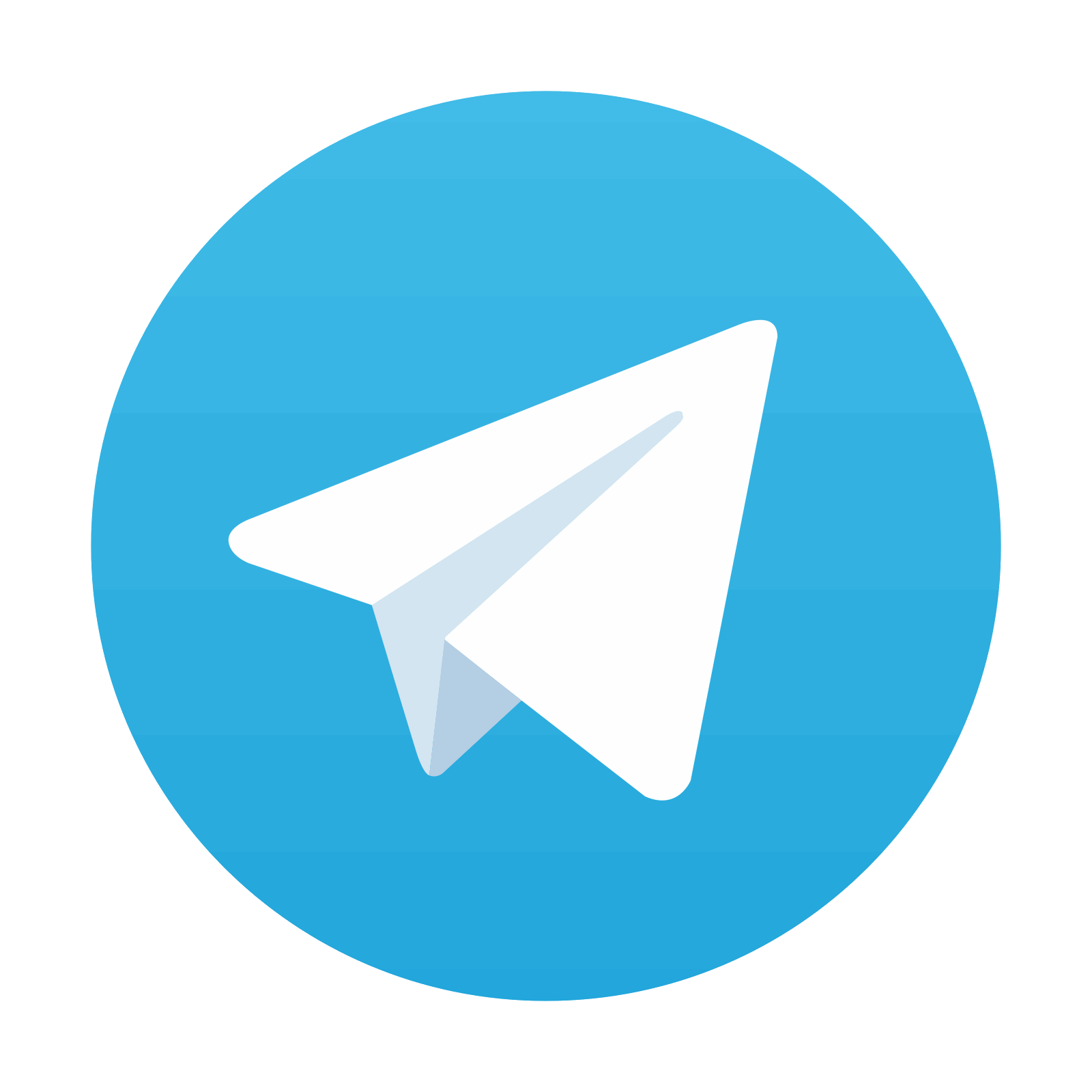
Stay updated, free articles. Join our Telegram channel

Full access? Get Clinical Tree
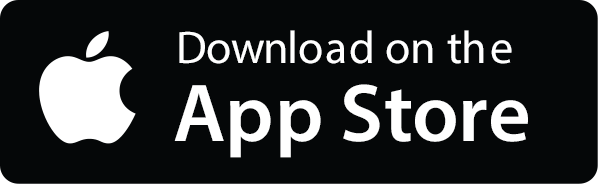
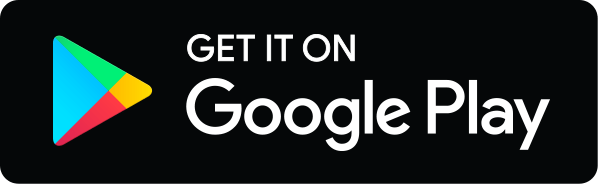