Skin Sense
In this chapter we look at the information that comes from cutaneous receptors, sensory receptors in the skin, and from the very similar ones that can be found in the gut and other visceral organs. The whole system is often loosely termed the somatosensory system, but this strictly also includes receptors from muscles and joints, which as proprioceptors are considered in Chapter 5.
Preamble: sensory processing in general
The conventional thing at this point would be to launch immediately into a description of the cutaneous sensory receptors, their afferent fibres, the spinal cord, the central pathways, and so on and so on. But in physiology in general, and above all in neurophysiology, if there are difficulties to be faced they lie not so much in assimilating these undeniably essential but nevertheless not-always-very-enlightening details, but in understanding the system as a whole, and above all what it is for. We make no apology then, since this is the first sensory system you have encountered, for beginning at a much deeper level, inviting you to think a little about the purpose not just of the cutaneous system, but sensory systems in general. What use is sensory information? How does its use determine how it is processed?
It is not easy to think clearly about our own sensory systems, as we all have this overpowering feeling of sitting inside our head as in a cinema, with all this sensory stuff being projected in front of us and providing us with ‘conscious sensation’. Being an egocentric species, we naturally therefore imagine that the purpose of sensory systems must be to deliver as accurate a picture of the outside world as possible to this little man in the head. Leaving aside the fact that consciousness is itself a difficult subject to think about scientifically – it is left to the very end of the very last chapter – intellectually, this is scarcely a very satisfying concept. For a start, it raises the natural question whether the little man in the head has a little man in his head. Nor is it a very biological way of regarding sensory processing. Systems do not evolve unless they are useful to their owners, ultimately in enhancing their ability to reproduce. The question we should be asking therefore is what we do with our sensory information, and what sensory systems need to do to make this information as biologically useful as possible. An analogy may make this clearer.
![]() |
Here we see two representations of the same area of Dorset countryside. Which is better? In one sense, the aerial photograph: it tells us exactly what we would see if we were actually there in the sky. But for an afternoon’s walking, we take the map, for it tells all the things we need to know if we want to interact with the landscape. More specifically, it provides information about what, where and how. To plan our walk we need to know what there is out there, the goals that motivate the walk itself – perhaps the village pub, perhaps a tourist attraction of some kind. To get to the goal, we need to know where it is in relation to our own location so that we can orientate ourselves and set off in the right direction; and finally we need hints about how to achieve our goal with the least effort and discomfort – avoiding the steepest inclines, crossing rivers without getting wet. What the helpful people at Ordnance Survey have done is to separate off these different kinds of information with different colour codes, so that we can convert them directly into actual directed movements: the map is the first stage in a process of translation, from sensory stimuli to motor responses.
Again and again we shall find when looking at
sensory systems that the information from sensory receptors is almost immediately segregated into different pathways reflecting different kinds of use that is going to be made of the information, in terms of generating movement. In vision, for instance, we shall see that one stream of information is devoted to localization of objects – and ourselves – in relation to the visual world so that we can go towards them, or reach out and grasp them. Another stream is devoted to recognizing visual objects so that we can make decisions about our reactions to them. In the somatosensory system, we shall see an even greater degree of specialization, which starts in the receptors themselves. One whole class of receptors in the skin is concerned exclusively with recognizing stimuli that demand immediate responses of one kind or another – withdrawing from pain, brushing off an insect – while others provide information that improves the precise control of manipulation. A particularly clear example is temperature sense. One might think the logical thing would be to have a single receptor whose firing frequency was simply proportional to temperature over some useful range. But in fact what we have are two kinds of receptor, conveying two very different types of information: one signals warmth, the other cold. They have evolved this way because each corresponds to a very specific kind of homeostatic response: it is the need to make particular responses that has driven the evolution of different receptors and sensory systems, rather than the other way round.
sensory systems that the information from sensory receptors is almost immediately segregated into different pathways reflecting different kinds of use that is going to be made of the information, in terms of generating movement. In vision, for instance, we shall see that one stream of information is devoted to localization of objects – and ourselves – in relation to the visual world so that we can go towards them, or reach out and grasp them. Another stream is devoted to recognizing visual objects so that we can make decisions about our reactions to them. In the somatosensory system, we shall see an even greater degree of specialization, which starts in the receptors themselves. One whole class of receptors in the skin is concerned exclusively with recognizing stimuli that demand immediate responses of one kind or another – withdrawing from pain, brushing off an insect – while others provide information that improves the precise control of manipulation. A particularly clear example is temperature sense. One might think the logical thing would be to have a single receptor whose firing frequency was simply proportional to temperature over some useful range. But in fact what we have are two kinds of receptor, conveying two very different types of information: one signals warmth, the other cold. They have evolved this way because each corresponds to a very specific kind of homeostatic response: it is the need to make particular responses that has driven the evolution of different receptors and sensory systems, rather than the other way round.
If the only purpose of sensory systems was to relay as exact an image as possible of the outside world to the little man in the head, there would be no need for ‘sensory processing’ at all. As soon as you realize that sensory systems are simply mapping from stimuli to responses, much that is otherwise incomprehensible in the brain suddenly begins to make sense. So we urge you, as you read the following chapters, to ask yourself all the time: What is this for? Only function makes sense of structure.
Structures and pathways
Types of cutaneous receptor
The afferent fibres from cutaneous receptors are bipolar cells: their bodies lie in the dorsal root ganglia near the spinal cord, and axons run all the way from the sensory endings in the skin to their terminals within the central nervous system. The dorsal roots are connected in an orderly way to different areas of the skin, and one may draw maps of the body surface showing the dermatomes or regions projecting to each dorsal root.
The demarcation of the different zones is not actually as sharp as such idealized representations imply, and because of overlap between adjacent dermatomes, each point on the body surface is connected to at least two dorsal roots; overlap is more marked for touch than it is for pain or temperature. Sensory fibres from the viscera are found in both the sympathetic and parasympathetic divisions of the autonomic nervous system.
The former pass in peripheral sympathetic nerves to the sympathetic chain, and thence via the dorsal root ganglia (where their cell bodies are) to the dorsal root; parasympathetic afferents of the sacral region travel with the corresponding efferents and again have their cell bodies in the dorsal root ganglion, while the cell bodies of the cranial afferents (in the vagus) are in the inferior (nodose) ganglion. ‘Vagus’ means ‘wandering’, and despite its localized connection to the central nervous system, it has an extremely widespread distribution in the thorax and abdomen.


![]() |
![]() |
![]() |
At the peripheral end, the fibres branch and terminate either as naked endings or in terminal encapsulations, of which many varieties have been described.
One such is the Pacinian corpuscle, whose responses to deformation were discussed in Chapter 3.
Others include the Meissner corpuscle, the Merkel disc, and the Ruffini ending (the latter is very similar to the Golgi tendon organ found in muscle). Encapsulated endings are found mainly in hairless or glabrous skin: the palms of the hands and soles of the feet, the lips, eyelids, mucosal surfaces and parts of the external genitalia. Some, notably the Pacinian corpuscles, are distributed in visceral structures and in joints and ligaments and deep connective tissue. Free or naked endings are abundant in hairy skin, some innervating the hair follicles themselves and sensing hair movement, and are also to be found in both glabrous skin (in dermis, but often penetrating the epidermis, as below) and in deep fascia and visceral organs. Their afferent fibres are small and sometimes unmyelinated, falling into group C and group Aδ (or III and IV, with a few in II): the fibres from encapsulated endings are mainly of group Aβ (or II). (See Table 4.1; cf. also Box 2.1.) In addition, there are specialized sensory structures associated with sinus hairs (the eyelashes, for example, or the whiskers of a cat). The hair is surrounded at its base by pressure-sensitive Pacinian corpuscles; in the middle it is encircled by a ring of Merkel discs, and along part of its length the hair is linked by thin filaments to a palisade of fastadapting lanceolate endings. The result of this battery of mechanoreceptors is to provide the brain with exquisitely sensitive and highly directional information about displacements of the hair brought about by contact with external objects.


![]() |
![]() |
![]() |
Table 4.1 Types of skin receptor | ||||||||||||||||||||||||||||||||||||||||
---|---|---|---|---|---|---|---|---|---|---|---|---|---|---|---|---|---|---|---|---|---|---|---|---|---|---|---|---|---|---|---|---|---|---|---|---|---|---|---|---|
|
It is only recently that we have begun to form a clear picture of what this great variety of receptor types in the skin is actually for. Broadly speaking, there is a clear division between the large afferents (Aβ) coming entirely from encapsulated mechanoreceptors, and the small
afferents (Aδ and C) from free endings and other fibres that respond to a wide range of stimuli, thermal and chemical as well as mechanical. Obviously these receptors give rise to what we feel when the skin is stimulated. But the question of the correspondence between the categories of feelings from the skin (sensory modalities, classically warmth, cold, pain, various mechanical categories, ‘deep pressure’, ‘light touch’ and so on) and categories of nerve fibre is a complex and controversial one, and is discussed more fully later in this chapter (p. 91).
afferents (Aδ and C) from free endings and other fibres that respond to a wide range of stimuli, thermal and chemical as well as mechanical. Obviously these receptors give rise to what we feel when the skin is stimulated. But the question of the correspondence between the categories of feelings from the skin (sensory modalities, classically warmth, cold, pain, various mechanical categories, ‘deep pressure’, ‘light touch’ and so on) and categories of nerve fibre is a complex and controversial one, and is discussed more fully later in this chapter (p. 91).
One might perhaps have expected each type of ending to correspond to one of the classical modalities, but this turns out not to be the case. The naked or free endings seem to serve the modalities both of warmth and pain, and are probably sensitive to mechanical stimuli as well. Specific encapsulated endings for cold have recently been described, but do not yet have a name: unusually for encapsulated endings, their axons fall into the Aδ category. All the other endings that are known are mechanoreceptors of one kind or another, and it is clear that the classical descriptions of ‘light touch’ or ‘deep pressure’ are completely inadequate for classifying the various kinds of mechanical stimuli to which they respond. A stimulus may generate uniform pressure over an area of skin, or it may cause shear if it exerts a sideways force, or tension if it is sticky, or stretch as a result of folding; there may be localized indentation, or more complex spatial textures; and all these stimuli may vary in time.
![]() |
In many cases the structure of the endings makes it fairly clear what they do. The concentric layers of the Pacinian corpuscle imply a rather non-directional sensitivity to local deformation. As we saw in Chapter 3, it is often used as an example of complete adaptation; but it is important to realize that this is only true of applied displacements or deformations, and not of pressure. We saw that a suddenly applied, maintained increase in pressure will not show adaptation, because collapse of the lamellae will not alter the force that is felt by the central receptive structure. Ruffini organs consist of branched naked nerve endings twisted in between collagen fibres anchored to nearby muscle cells and other structures. Tension in these fibres appears to distort the nerve endings and thus stimulates them; they adapt incompletely. (Very similar endings, responding to tension, are found in the tendons of muscles: as proprioceptors, these Golgi tendon organs are considered in Chapter 5.) Merkel discs lie much closer to the outside world, at the bottom of the epidermis, to which they are attached by desmosomes: they are extremely sensitive to deformation of the skin, and show incomplete adaptation. In classical terms, they might be regarded as light touch receptors; more functionally, they may serve to indicate local skin deformation and contact. Finally, Meissner corpuscles, like Ruffini endings, have nerve endings that are associated with collagen fibres, and are completely adapting. The corpuscles are found in the dermal folds beneath the epidermal ridges, and the collagen fibres are connected sideways with the epidermal cells on each side. Thus they are ideally placed to register sideways shearing of the skin, of the kind that is experienced, for example, when holding an object in the fingers and then lifting it (they are in fact most commonly found in the fingertips). A curious feature is that their density declines dramatically with age, from some 50/mm2 at 10 years to about 10/mm2 at 50.
The fact that many of these receptors show adaptation means that it is only when the pattern of stimulation to the skin is changing that we perceive very much. Shut your eyes, and run your hand over some nearby object, perhaps the table-top: your hand gives you a vivid impression of its texture, of its cracks and dents and all its other surface properties. Now keep your hand still: at once this perception vanishes, and it is hard to be sure that one is sensing anything at all except its temperature. It is evidently the temporal patterns of firing of mechanoreceptors from the skin, combined with knowledge of the movements we make, that determines what we feel when we touch something.
Afferent pathways
The broad division of afferent fibres into two groups (small, mostly from free endings; large, from encapsulated endings) is reflected in their mode of termination within the central nervous system. In the spinal cord, they both terminate in the dorsal horn, but in different parts of it. The dorsal horn is conventionally divided into a set of six roughly parallel layers, Rexed’s laminae. Smaller fibres enter directly from the dorsal root and terminate in layers I and II; the largest fibres sweep round dorsally on their
way up to their main destinations higher up, but branches terminate in layers III-V, where among other cells they make contact with short interneurons conveying mechanical information back to layer II. This is a fact of some significance in the processing of pain signals. The neurons of the dorsal horn are also under firm control from the brain; if the descending pathways that enter layer II are experimentally blocked the receptive fields of dorsal horn cells undergo radical alteration. We shall see that this descending control is also of huge importance in the case of pain.
way up to their main destinations higher up, but branches terminate in layers III-V, where among other cells they make contact with short interneurons conveying mechanical information back to layer II. This is a fact of some significance in the processing of pain signals. The neurons of the dorsal horn are also under firm control from the brain; if the descending pathways that enter layer II are experimentally blocked the receptive fields of dorsal horn cells undergo radical alteration. We shall see that this descending control is also of huge importance in the case of pain.
![]() |
The mode of projection to higher levels of these two divisions is also distinctive. Branches of the larger fibres, from encapsulated mechanoreceptors, essentially turn their back on their spinal cord, turning upwards soon after entering the dorsal horn, to form a pair of large ascending tracts called the posterior or dorsal columns.
These continue ipsilaterally up to the level of the medulla and terminate in the dorsal column nuclei (gracile and cuneate); the gracile receives afferents from sacral, lumbar, and lower thoracic segments, and the cuneate from higher regions.
The smaller fibres, in contrast, project only to the cord itself, and ascending fibres are derived from second-order neurons. As will become apparent, this difference between large and small fibres reflects a profound difference in their function. The large fibres are not much concerned with the initiation of responses: their main job is to provide feedback that is used by the brain, particularly by the primary motor cortex, to improve the way in which it manipulates objects. This phylogenetically new system, sometimes described as epicritic (implying the provision of precise, objective information) is the opposite of the ancient system of small fibres, sometimes called protopathic (‘primitive feeling’) whose function is to cause appropriate responses to quite specific types of stimuli (Table 4.2), and incidentally to give rise to corresponding feelings that can be extremely marked. Such responses as withdrawal from a noxious stimulus, brushing off an insect, paroxysms induced by tickling, thermoregulatory responses to warm and cold, and sexual reactions to caresses, all fall in this category, and are characterized by instinctive and often powerful behavioural responses that are strongly resistant to conscious control. Of these, pain is of such fundamental clinical importance that it is discussed separately later in this chapter.


![]() |
Table 4.2 Protopathic versus epicritic | ||||||||||||||||
---|---|---|---|---|---|---|---|---|---|---|---|---|---|---|---|---|
|
The final sensory destination of these ascending pathways is in the thalamus and then to cerebral cortex, and this is a convenient point to have a preliminary look at these areas, structures that we will be coming across again and again in subsequent chapters, since they dominate all the neural systems of the brain.
Cerebral cortex
Cortex is the rind of the brain. We perhaps tend to overestimate it, partly because it is on the outside and therefore relatively easy to investigate, and partly because for us humans it is the bit we’re most proud of, in which – because of its huge expansion – we differ most from other species. But we need to remind ourselves not to be totally infatuated with humans. When we look at sheep or gerbils or sparrows, we see that despite their cortical deficiency they lead happy and useful lives, and do all the biologically important things in life no worse than us, and in some ways better. At the risk of stating the obvious, cortex is for refinement, a bolt-on extra whose function is to add flexibility and programmability to what otherwise would be rather robotic. Cortex is the icing on the cerebral cake.
Apart from expansion, another evolutionary trend has been the superseding of a simpler three-layered sheet of neurons called archicortex by the more elaborate six-layered form, neocortex. Neocortex first appears in reptiles, but in marsupials and mammals it starts to expand dramatically in area. In most mammals it forms by far the largest part of the cortex, elaborately wrinkled into the complex pattern of sulci and gyri that enables a large surface area to be stuffed into a relatively small volume. By the time we get to Man, about 90 per cent of the cortex is neocortex – its total area is about the same as an unopened broadsheet newspaper – with some 1010 neurons. Under each square millimetre of cortical surface lie nearly a quarter of a million neurons.
![]() |
The structure of neocortex is essentially quite uniform, with only two basic kinds of neurons. The main output comes from the large pyramidal cells of layer V, forming what are known as the projection efferents, which go to subcortical destinations. However, in a sense the whole point of the cortex is to bring many diverse types of input and output into functional association, and to a large extent this is brought about by smaller pyramidal cells having cell bodies in layer III, that send off axons (association fibres) terminating in vertical columns in some other area. Pyramidal cells have a long apical dendrite, and a group of basal dendrites at their base, where the axon exits downwards, so that the cells are capable of being influenced by all layers of the cortex, within a radius of half a millimetre or so. They are excitatory, releasing glutamate or possibly aspartate. The other main type of neuron, the stellate cell, is entirely confined to the cortex itself. It comes in two main kinds: smooth stellates are inhibitory and release γ-aminobutyric acid (GABA); spiny stellates are excitatory and are covered in a profusion of dendritic spines. These neurons are organized horizontally into layers and vertically into columns some 0.5 mm in diameter. Corresponding to the projection efferents, on the input side there are the specific projection afferents of subcortical origin (mostly in fact from the thalamus) that ramify into large terminal trees around layers III and IV and tend to end on stellates rather than on pyramidal cells. There is also a diffuse input mostly from reticular formation, influencing the activity of cortex in a rather
global way: this is discussed in Chapter 14 (p. 287). In addition to these primary types of neuron, other varieties of interneuron communicate from layer to layer as well as horizontally; many of them are inhibitory in nature and probably carry out functions analogous to lateral inhibition (see below, p. 88).
global way: this is discussed in Chapter 14 (p. 287). In addition to these primary types of neuron, other varieties of interneuron communicate from layer to layer as well as horizontally; many of them are inhibitory in nature and probably carry out functions analogous to lateral inhibition (see below, p. 88).
![]() |
![]() |
![]() |
Of the six neocortical layers, III, V and VI contain most of the pyramidal cell bodies, while the stellates are mostly in layers II and IV, and there are horizontal interneurons in layer I. Because different regions have different proportions of input and output, the relative size and appearance of these layers varies from place to place. Because the larger projection pyramidals are mostly in layer V, it tends to be more prominent in motor areas; conversely, because layer IV is where the bushy afferents from the thalamus terminate, it tends to be more prominent in sensory cortex. A striking example is at the edge of a visual region of cortex, Area 17, whose massive inflow of fibres from the eye produces the dense stripe of Gennari. There is a broad division into granular cortex (sensory, input layers bigger) and agranular (motor, output), with other intermediate types. Finer differences in sizes of layers and density of fibres and so on form the basis of the division of cerebral cortex into the Brodmann areas, whose numbers are a convenient shorthand for referring to different cortical regions. Increasingly, however, it is found that the classic Brodmann areas need to be further subdivided because of obvious functional differences between one sub-region and another. 

![]() |
Thalamus
The thalamus is strategically placed, right in the centre of the cerebral hemispheres like jam in a doughnut. Its neurons project entirely to the cerebral cortex, and much of its input comes from the various sensory systems, so it is natural to think of it as a relay for afferent fibres to the cortex. But if that were all it was it would be perfectly pointless, and in fact it is clear from its other connections that it is far more than that. In the first place, fibres don’t just go from the thalamus to the cortex: they go the other way round as well; there is a massive, quite topographically precise, projection from layers V and VI of the cortex back down to the thalamic nuclei with which they are associated. Secondly, if it were just a relay, then you would only expect sensory areas to have thalamic connections. But in fact nearly all cortical areas have projections from the thalamus, including areas that are very definitely motor. It is important to appreciate that cortex and thalamus work together very much as a single unit. 

![]() |
![]() |
In cross-section the thalamus is divided by the internal medullary lamina (shown below in purple) into three major areas: anterior, medial and lateral. The subdivisions of these regions are illustrated diagrammatically below, and listed in Table 4.3. For the moment, in the context of the somatosensory pathways, we should note two nuclei, the ventroposterolateral (VPL), which receives somatosensory information from the entire body apart from the head and face, which are dealt with by the ventroposteromedial (VPM) nucleus. Second-order
afferents from the dorsal column nuclei first cross the midline, and then continue through the brainstem as the medial lemniscus to VPL, from which third-order fibres travel first through the thalamic reticular nucleus (TRN: blue, above) and then through the internal capsule to a region of cerebral cortex called the somatosensory cortical area or S1 (Brodmann areas 3, 2 and 1).
On the right, the various subdivisions of the thalamus are shown, and the corresponding areas of cerebral cortex. (MG, LG: medial and lateral geniculate; VPM, VPL, VL, VA: ventroposteromedial, ventroposterolateral, ventrolateral and ventro-anterior nuclei; LP, LD: lateral posterior and lateral dorsal; DM: dorsomedial; CM: centromedian; Ant: anterior; TRN: thalamic reticular nucleus. Afferent tracts include the trigeminal, medial lemniscus and spinothalamic.)
afferents from the dorsal column nuclei first cross the midline, and then continue through the brainstem as the medial lemniscus to VPL, from which third-order fibres travel first through the thalamic reticular nucleus (TRN: blue, above) and then through the internal capsule to a region of cerebral cortex called the somatosensory cortical area or S1 (Brodmann areas 3, 2 and 1).


![]() |
Throughout the projection system linking encapsulated mechanoreceptors to the cortex via the thalamus – often called the lemniscal system – the general topological relationship between the representations of different areas of the skin is preserved, so that the somato sensory cortex itself embodies a map of the opposite side of the skin surface. This map is topologically correct in the sense that neighbouring parts of the body surface are on the whole represented by neighbouring regions of cortex, but very much distorted in shape; those areas with the greatest cutaneous sensitivity and acuity such as the hands and lips have a much larger area devoted to them than regions such as
the trunk and back. These distortions can be represented by a correspondingly distorted sensory homunculus (above). A second somatosensory area, S2, is found in primates and differs from S1 in receiving somatosensory information from both sides, and to some extent in the modalities to which it responds.
the trunk and back. These distortions can be represented by a correspondingly distorted sensory homunculus (above). A second somatosensory area, S2, is found in primates and differs from S1 in receiving somatosensory information from both sides, and to some extent in the modalities to which it responds.
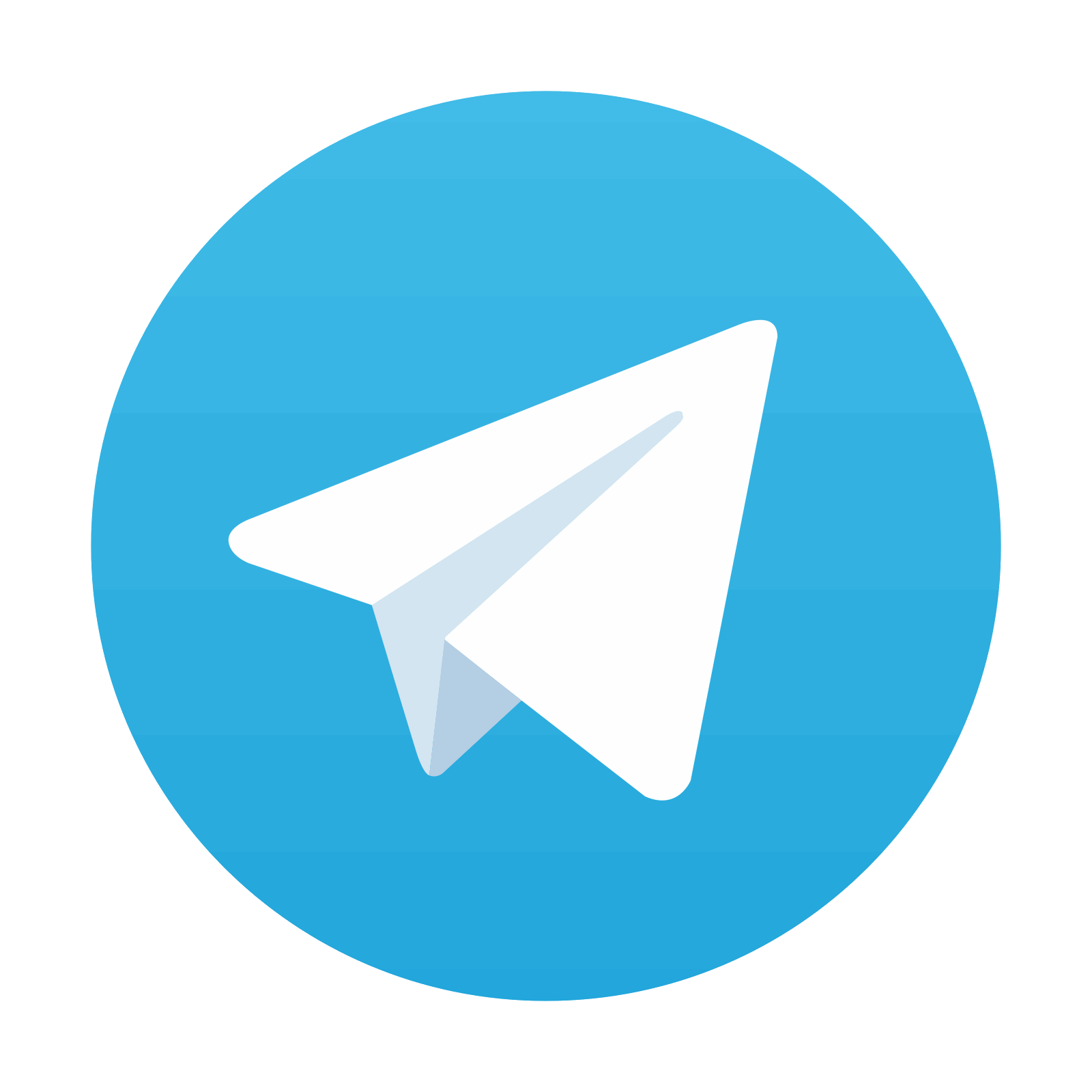
Stay updated, free articles. Join our Telegram channel

Full access? Get Clinical Tree
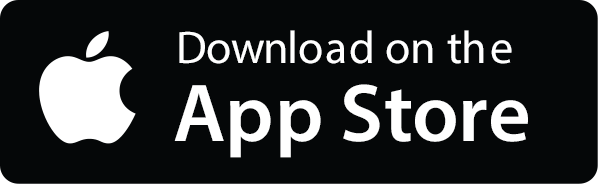
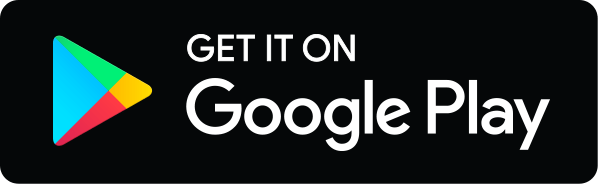