In a study examining the distribution of 600 focal seizures across the sleep–wake cycle recorded during video–electroencephalogram (EEG) evaluations, 43% were found to arise from sleep, the majority from non–rapid eye movement (NREM) stage 1 (23%) or 2 (68%), and none arose from REM sleep (6). Secondary generalization tends to occur more often during sleep (28%) than wakefulness (18%), and frontal lobe seizures tend not to secondarily generalize during sleep (6–8). Differences in peak seizure occurrence in relation to the rise in salivary melatonin between patients with temporal and frontal lobe epilepsy were observed. Temporal lobe seizures occurred most frequently in the 6 hours before and frontal seizures 6 to 12 hours after the dim light melatonin onset, suggesting that circadian influences may underlie some of these clinical observations (9).
Recent work has focused on clock time of seizures in different epilepsy syndromes. Using scalp EEG recordings, two studies found that temporal lobe seizures occurred more often during daytime hours with a peak incidence from 15:00 to 19:00, whereas extratemporal seizures peaked between 19:00 and 23:00 (10) or in the early morning hours associated with awakening (11). In an investigation involving 131 adult patients, peak timing of seizures varied by location of the seizure-onset zone identified by invasive EEG recordings (12). Specifically, mesial temporal lobe seizures had two peaks between 16:00 to 19:00 and 07:00 to 10:00, while neocortical temporal and occipital seizures peaked between 16:00 and 19:00, and frontal and parietal seizures between 04:00 and 07:00. In the second invasive EEG study, among 33 focal epilepsy cases, temporal lobe seizures occurred preferentially during the day (11:00 to 17:00), frontal seizures at night (23:00 to 05:00), and parietal seizures in the evening (17:00 to 23:00) (13).
The peak occurrence of generalized seizures was found to vary by seizure type with tonic–clonic seizures peaking between 06:00 and 09:00, tonic seizures between 21:00 and 24:00, absence seizures between 09:00 and 12:00, atonic seizures between 12:00 and 18:00, and myoclonic seizures between 06:00 and12:00 (14). Timing of epileptic spasms was found to be age dependent with peaks between 09:00 to 12:00 and 15:00 to 18:00 in children ≤3 years and between 06:00 to 09:00 in those older than 3 years (15).
Patients with sudden unexpected death in epilepsy (SUDEP) are more likely to have a history of nocturnal seizures than are people living with epilepsy (36% vs. 17%) (16). Most sleep-related cases of SUDEP occur between in the early morning hours (04:00 and 08:00). While the mechanisms underlying seizure timing are poorly elucidated, further investigation is warranted given the potential for therapeutic interventions.
EFFECTS OF SLEEP ON SEIZURES AND THE EEG IN EPILEPSY
The first systematic study of the diagnostic yield of sleep on the EEG was performed by Fred and Erma Gibbs in 1947 (17). Among 500 epilepsy patients, interictal epileptic discharges (IEDs) were observed in 36% of wake EEGs, increasing to 82% with sleep. Sleep activated additional epileptic foci in some patients who had only one focus during wake. The detection of IEDs by recording sleep was greatest for patients with focal seizures and least with absence seizures. Patients with psychomotor seizures often had normal wake EEGs, but 95% had IEDs during sleep.
Since these early observations, the activating effect of sleep on seizures and the EEG has been extensively investigated (18). The neuronal networks that generate wake, NREM sleep, and REM sleep give rise to different physiologic characteristics influencing the likelihood of seizure and IED occurrence (19,20). NREM sleep is a state of EEG synchronization and relative preservation of antigravity muscle tone. Synchronous oscillations of cortical neurons that generate sleep spindles, K complexes, and tonic background slow waves during NREM sleep promote seizure propagation and IEDs. In contrast, REM sleep is a desynchronized state characterized by the loss of skeletal muscle tone. Desynchronization of the EEG impedes seizure propagation during REM sleep and wake. Preservation of antigravity muscle tone during NREM sleep permits the expression of seizure-related movements, while its absence during REM sleep blocks the clinical expression of seizures.
Many studies demonstrate that NREM sleep activates IEDs in focal epilepsies (21,22). Interictal spikes increase at sleep onset, peaking in stage 3 (slow-wave sleep [SWS]), and then declining in REM sleep to levels lower than wakefulness. Similarly, the field of an IED typically increases in NREM sleep, becoming more diffuse—even generalized in SWS compared to light NREM sleep and more restricted in REM sleep. The extent and laterality of IEDs during REM sleep may be useful in confirming the primary epileptogenic zone in patients with pharmacoresistant temporal lobe epilepsy (TLE) (21,22).
Variations in IEDs with the sleep–wake cycle are also observed in the generalized epilepsies, although sleep is a less important activator because IEDs are often present in the wake EEG (23). Sleep further activates IEDs in patients with absence and/or GTC seizures (23,24). Typically, spikes increase with sleep onset progressively through SWS, diminish sharply in REM sleep, and increase again in the morning after awakening. Generalized spike–wave discharges become more disorganized, increased in amplitude, and slow in frequency in NREM sleep, whereas their morphology in REM sleep and wakefulness is similar (24). The EEG is most abnormal after the morning awakening or nighttime arousals in patients with awakening epilepsies such as juvenile myoclonic epilepsy. In contrast, the EEG tends to be normal during wakefulness or show an increase in IEDs during sleep in the generalized sleep epilepsies.
EFFECTS OF SLEEP DEPRIVATION ON EPILEPSY
The effect of sleep deprivation on epilepsy has been the subject of much investigation. In the first systematic study, sleep-deprived EEGs were performed after 26 to 28 hours of wakefulness, and IEDs were seen in 34% of 89 subjects with at least one seizure and a normal routine EEG, 56% of 34 patients with convulsive epilepsy and IEDs on routine EEGs, and 0% of 20 patients with neurologic disorders other than epilepsy (25). Subsequent work suggested that for most seizure types, spontaneous sleep and sleep-deprived EEGs produce similar activation rates, and seizures are more likely to be activated by sleep or sleep deprivation in patients with generalized rather than focal epilepsy (24). In a study comparing the yield of different EEG protocols, IEDs were recorded in 28% of subjects only following total sleep deprivation (TSD), defined as 24 hours of more of sustained wakefulness, compared to routine wake and drug-induced sleep EEGs, and TSD activated a new epileptic focus in 7% of cases (26). As the yield of recording IEDs due to repeated recordings was estimated to be in the range of 14% to 19%, the authors claimed their findings were unlikely to be due to sampling effects. In recent study involving patients with new-onset epilepsy, IEDs were observed in 92% of TSD EEGs versus 58% of drug-induced sleep and 44% of routine recordings (27). Similarly, a prospective study of 721 subjects who had a second EEG (routine, drug-induced sleep or TSD) after an inconclusive initial EEG found a significantly greater percentage containing IEDs after TSD as compared to a second routine record (23% vs. 10%) (28). Comparative studies largely confirm that TSD activates IEDs in 23% to 93% of patients with definite or suspected seizures (18). Whether the activation of IEDs on EEG produced by sleep deprivation is due to sleep itself (greater amounts of sleep recorded, sampling effects) or because TSD exerts an independent activating effect is uncertain.
The effects of less extreme degrees of sleep deprivation on the EEG are not fully elucidated. Among 71 patients with focal epilepsy, the odds of a seizure the next day decreased for each hour of sleep the prior night (29). In another study based on 2-year sleep and seizure diaries completed by 14 adults with TLE, the probability of a seizure was significantly greater after a night of sleep deprivation (at least 1.5 hours less than usual) compared with normal sleep (30). Indeed, sleep deprivation is reported as a seizure precipitant by up to one-third of people with epilepsy (3,29,31). Several studies using transmagnetic stimulation have reported a significant increase in cortical excitability following sleep deprivation in people with epilepsy compared to controls (32). These findings suggest that modest amounts of sleep loss can activate seizures and the EEG.
EFFECTS OF EPILEPSY THERAPIES ON SLEEP
Antiepileptic drugs (AEDs) have variable effects on nocturnal sleep and wakefulness (Table 37.2). The effects of AEDs may be due in part to a consolatory effect on sleep. Some AEDs tend to cause more sleepiness and drowsiness, while others can lead to insomnia.
Table 37.2 Effects of Chronic Antiepileptic Drug Therapy on Sleep Architecturea
0, denotes no change; —, denotes not reported.
aIncludes data acquired after achieving steady state.
Barbiturates and benzodiazepines suppress REM sleep, reduce sleep latency, and increase sleep efficiency, while phenytoin and valproic acid appear to increase light NREM sleep and increase arousals and awakenings (33). Carbamazepine (CBZ) is the most extensively investigated older AED. Studies suggest that the short- and long-term effects of CBZ on sleep differ. For example, a single 400-mg dose of controlled release CBZ in adults with newly diagnosed TLE and controls produced a significant reduction in REM sleep and increased stage shifts and REM sleep fragmentation, effects that reversed after 1 month of treatment (33).
Newer AEDs also affect sleep architecture but appear to have more favorable effects than do some of the older agents, perhaps contributing to their antiseizure properties. In particular, lamotrigine (LTG), topiramate, and zonisamide produce minimal alterations in sleep architecture in adult patients on relatively low-dose therapy, and LTG produced an increase in REM sleep in two studies (33–36). Gabapentin produced a deepening of sleep, increasing SWS percentage, and reducing sleep fragmentation in healthy subjects (37) and increased REM sleep in patients with epilepsy (33). Similarly, pregabalin was associated with a significant reduction in the number of awakenings and improvement in wake after sleep onset (38) and an increase in REM sleep (39) in patients with focal epilepsy, suggesting improvements in sleep continuity. Studies exploring the effects of levetiracetam are conflicting, but generally also show consolatory effects on sleep in both healthy subjects and adults with epilepsy (40,41). Finally, in several studies, tiagabine has been shown to increase SWS and reduce sleep fragmentation in older, otherwise healthy adults (42).
Like AEDs, vagal nerve stimulation (VNS) produces alterations in sleep and wakefulness. Although improved daytime alertness has been observed, VNS stimulus intensity >1.5 mA has been associated with a reduction in REM sleep and increased awakenings, wake after sleep onset, and stage 1 sleep, suggesting a shift to lighter sleep stages and more sleep fragmentation (43). VNS therapy can produce cyclical reductions in airflow during sleep that at time meets the polysomnographic (PSG) criteria for sleep apnea in adults and children with epilepsy (44,45). Lowering the stimulus frequency from 30 to 20 Hz was shown to ameliorate VNS-induced respiratory events (46). Therefore, PSG should be performed prior to VNS implantation in all patients due to the potential development of sleep apnea. Patients should be monitored after implantation for sleep apnea symptoms. Repeat PSG should be performed in those with new-onset snoring, witnessed apnea, or daytime sleepiness and in patients with previously diagnosed sleep apnea as treatment adjustments may be required.
Sleep Organization in Epilepsy
A number of sleep architectural abnormalities have been reported in people with epilepsy. These include disruption and lability of REM sleep with reduced percentage of sleep time spent in REM sleep; increased percentage of wake after sleep onset; prolonged sleep or REM sleep onset; and increased number of arousals, awakenings, and stage shifts (47). These findings hold true even in seizure-free patients and are observed in patients with genetic generalized epilepsies and TLE, although more so in the latter. Sleep organization is more disrupted in TLE than in FLE, with more time wake after sleep onset, more awakenings, increased duration of light NREM sleep, reduced sleep efficiency, and reduced SWS (7,22).
Nocturnal generalized motor seizures in adults with genetic generalized epilepsy or focal epilepsy are associated with a decrease in total sleep time and REM sleep, prolonged REM latency, and increased light NREM sleep, increased percentage of time wake after sleep onset, and increased arousals (22,48). In a video–EEG series of TLE, a significant decrease in REM sleep was found on nights following daytime seizures, an even greater decrease in REM sleep on nights of seizures, and the greatest amount of REM suppression on nights in which seizures occurred early in the sleep period before the first appearance of REM sleep (48). Both diurnal and nocturnal seizures prolonged REM latency. Nocturnal, but not diurnal, seizures increased stage 1 sleep and decreased SWS. In a large study involving adults with epilepsy, compared to seizure-free patients, those who had generalized motor seizure during the day had significantly prolonged REM latency, increased stage 2 sleep, and decreased SWS and REM sleep the following night (49). A recent study reported a significant reduction in time in bed, total sleep time, REM sleep, SWS, and sleep efficiency as well as a significant increase in wake time after sleep onset in children with pharmacoresistant epilepsy compared to children with benign focal epilepsy and normal controls (50). In turn, a significant increase in REM sleep was observed in patients with mesial TLE seizure free at 2 years following resective surgery (51). Taken together, these findings suggest that sleep is inherently unstable in people with epilepsy; this instability promotes seizures, and seizures in turn fragment sleep, thus facilitating the epileptic process.
SLEEP–WAKE COMPLAINTS AND SLEEP DISORDERS IN EPILEPSY
Many sleep disorder symptoms and some primary sleep disorders including excessive daytime sleepiness (EDS), insomnia, and obstructive sleep apnea (OSA) are two to three times more common in people with epilepsy than in the general population (52–57) and adversely affect quality of life (52,54–56). This subject has been the focus of a comprehensive review (58).
EDS and insomnia are two of the most common complaints of people with epilepsy, often attributed to the effects of AEDs and seizures. One-third to one-half of the epileptic population report EDS (54,59). In a recent study involving 152 adults with epilepsy, 55% suffered from insomnia and more than 70% were rated as “poor sleepers” (56). Insomnia and poor sleep quality were significantly correlated with number of AEDs and depressive symptoms. As in the general population, sleep problems are more common among adults with epilepsy taking psychotropics for the treatment of mood disorders (55). In children with epilepsy, sleep problems are also common and adversely affect behavior and academic performance (57). Disturbed sleep, snoring, limb movements in sleep, daytime hyperactivity, EDS, and bedtime refusal are more common in children with epilepsy than in age-matched controls (57).
The prevalence of OSA in adults with epilepsy exceeds general population estimates of 25% for men and 10% for women (60). In the first PSG study, 33% of 39 patients (50% of males; 19% of females) with pharmacoresistant focal epilepsy without sleep complaints had OSA defined by an apnea–hypopnea index (AHI) of ≥10 (61). Predictors of OSA included male gender, higher body mass index (BMI), and a history of snoring, witnessed apnea, or nocturnal seizures. In a recent study involving 130 adults with epilepsy unselected for sleep disorders symptoms, the prevalence of OSA (AHI ≥ 10) was 30%, 16% having moderate-to-severe disease (62). Predictors of OSA included older age, dental problems, and higher standardized AED dose, a measure of drug burden. Male gender, older age, higher BMI, hypertension, and dental problems were associated with having a higher AHI. In older patients with epilepsy, the presence of sleep apnea is associated with worsening seizure control or late-onset seizures (63). Potential contributing factors include the effects of AEDs on upper airway tone as well as AEDs and inactivity leading to weight gain and endocrinopathies such as polycystic ovarian syndrome and hypothyroidism.
Understanding the predictors of OSA and other sleep disorders in epilepsy is important since treatment of OSA reduces seizures in 40% to 86% of cases, children included (58,64,65). A recent retrospective study found that continuous positive airway pressure (CPAP) therapy reduced seizure frequency by more than 50% (from 1.8 to 1 seizure per month) in 41 adults in the absence of AED adjustments (64). In another study, use of CPAP in 6 patients with focal epilepsy produced a marked reduction in spike rate on EEG, suggesting that sleep consolidation and correction of desaturation and hypercapnia may reduce epileptogenicity (66). These findings underscore the importance of routine screening and treatment of sleep disorders in people with epilepsy.
COMPLEX NOCTURNAL MOVEMENTS AND BEHAVIORS: DIFFERENTIATING NOCTURNAL SEIZURES AND PARASOMNIAS
While the differential diagnosis of abnormal sleep-related movements and behaviors is extensive, the focus of evaluation is usually on the distinction between nocturnal seizures and parasomnias (67). Parasomnias are undesirable physical events or experiences that occur during entry into sleep, within sleep, or during arousals from sleep that involve complex, seemingly purposely, goal-directed behaviors without consciousness. The primary parasomnias pertinent to this discussion are the NREM arousal disorders (confusional arousals, sleep terrors, and sleepwalking). Seizures in nocturnal frontal lobe epilepsy (NFLE) have a wide range of complexity and severity, overlapping considerably with disorders of arousal. Complex motor features typically of the hypermotor type involving complex movements of the trunk and proximal extremities that are repetitive, high-amplitude, and high-velocity, and asymmetric tonic seizures having dystonic, dyskinetic, and repetitive proximal movements are characteristic of NFLE (68). The lifetime prevalence of arousal disorders is nearly fivefold greater in relatives of probands with NFLE than in controls, suggesting an intrinsic link between the two (69).
A dysfunctional arousal mechanism involving the cholinergic system is hypothesized to underlie the shared pathophysiology of NFLE and NREM parasomnias. Mutations in the neuronal acetylcholine receptor in the autosomal dominant form of NFLE suggest a molecular basis for the disorder. These ion channel receptors are widely distributed on neuronal and glial membranes in cortical and subcortical regions, regulating the release of acetylcholine, gamma-hydroxybutyric acid, and glutamate, and producing a modulatory effect on arousals at the cortical and thalamic levels. Receptor mutations confer a gain of function with increased sensitivity to acetylcholine that is postulated to produce changes in the excitability of cortical and subcortical networks preferentially affecting the mesial frontal area facilitating intrinsic epileptogenesis and altering arousal mechanisms by destabilizing sleep (70). Activation of common pattern generators is thought to underlie the similar semiological manifestations of nocturnal seizures and arousal disorders (71). These are genetically determined neural circuits located in the mesencephalon, pons, and spinal cord that code for self-sustained patterns of behavior subserving innate motor behaviors essential for survival, such as feeding, locomotion, defense, and copulation.
The diagnosis of nocturnal seizures and parasomnias is often achieved through a comprehensive clinical history including timing, frequency, semiology, and evolution of typical events (Table 37.3). The Frontal Lobe Epilepsy and Parasomnias (FLEP) scale has been proposed as an adjunctive tool to identify patients with NFLE (Table 37.4) (72). A score of 0 or less is unlikely to be seen in epilepsy, whereas patients scoring 3 or greater generally have epilepsy. Laboratory testing is recommended for those with an FLEP score of +1 to +3. If diagnostic uncertainty persists despite routine clinical, EEG, and imaging assessments, video PSG with EEG (VPSG-EEG) may be warranted. This procedure combines PSG with 21-channel EEG to evaluate unexplained nocturnal behaviors when epileptic seizures are suspected, particularly when primary sleep disorders are under consideration and episodes are frequent. Due to the limitations of capturing a typical event in one night of recording, long-term VEEG is preferred when episodes do not occur at least every other night, primary sleep disorders such as OSA are not suspected, a history of postictal agitation or wandering exists, and patient cooperation is uncertain. In a recent VPSG-EEG analysis of 120 events of 44 patients with NFLE or NREM arousal disorders, 94% were correctly classified using a diagnostic decision tree based on a cluster analysis (73).
Table 37.3 Differentiating Nocturnal Frontal Lobe Epilepsy and Parasomnias
From Foldvary-Schaefer N, Aksheikhtaha Z. Complex nocturnal behaviors: nocturnal seizures and parasomnias. Continuum (Minneap Minn). 2013;19(1):104–131.
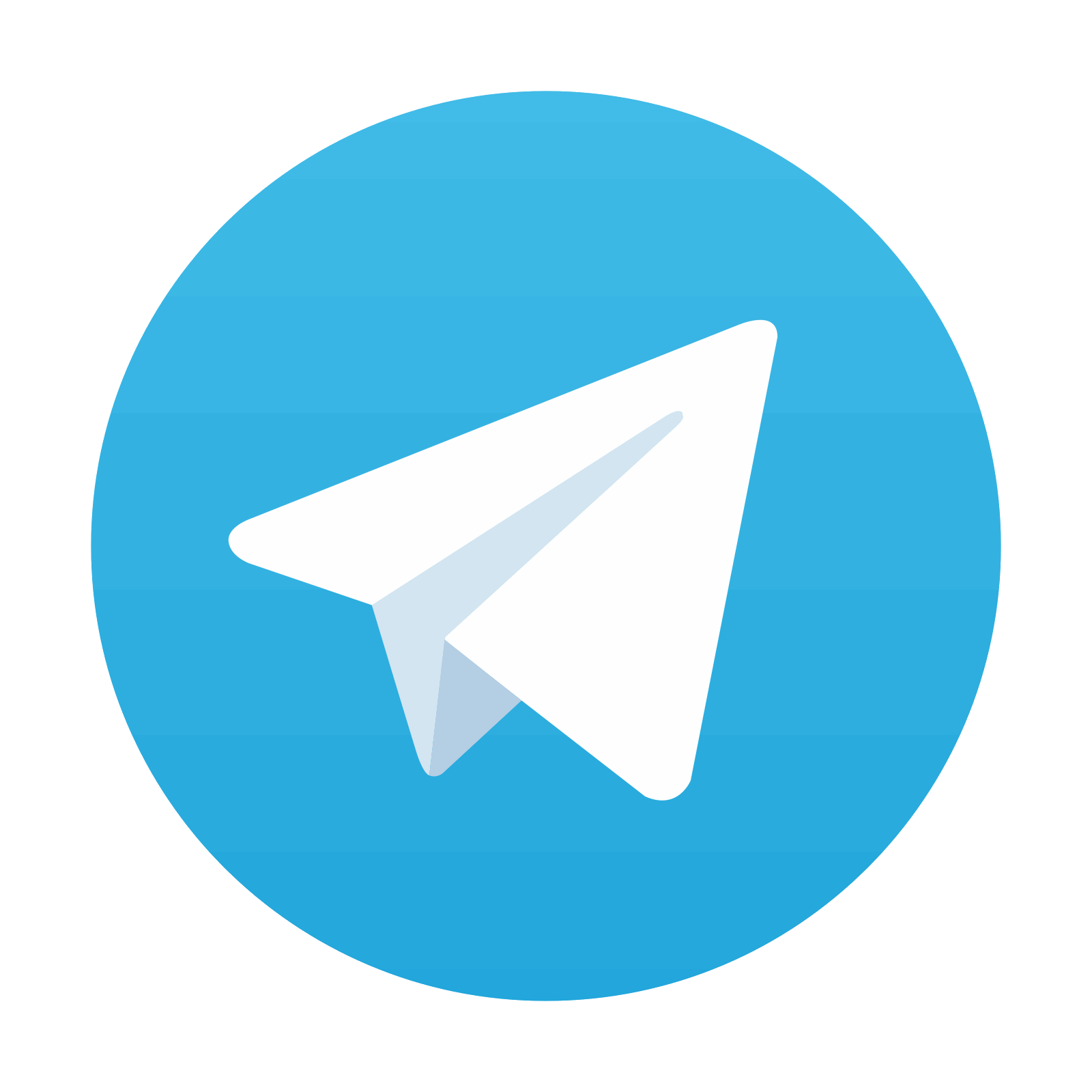
Stay updated, free articles. Join our Telegram channel

Full access? Get Clinical Tree
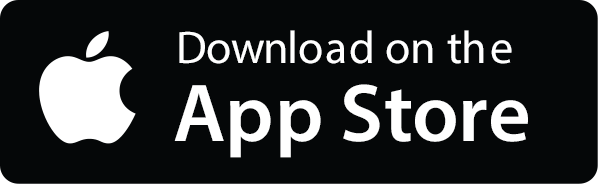
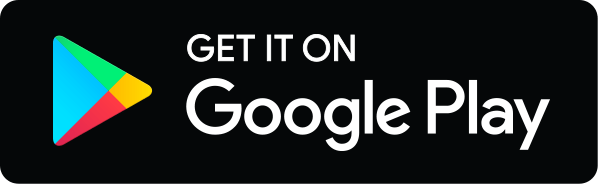