© Springer International Publishing AG 2017
Pascual Ángel Gargiulo and Humberto Luis Mesones-Arroyo (eds.)Psychiatry and Neuroscience Update – Vol. II10.1007/978-3-319-53126-7_1717. Stress and the Dynamic Fear Memory: Synaptic–Cellular Bases and Their Implication for Psychiatry Disorders
(1)
IFEC-CONICET, Departamento de Farmacología, Facultad de Ciencias Químicas, Universidad Nacional de Córdoba, Haya de la Torre y Medina Allende, Ciudad Universitaria, Córdoba, 5000, Argentina
(2)
Laboratory of Behavioral Neurobiology, Center of Biological Sciences, Federal University of Santa Catarina, Florianopolis, SC, Brazil
Abstract
There is consensus that the acquisition and storage of relevant aversive information allows organisms to cope with threat situations. Such a mnemonic process is supported by lasting modifications in the aversive neuronal circuitry, resulting in changes in the behavioral response. In this way, the capacity to form long-lasting emotional memories makes it possible to predict and anticipate a potential threat in future situations, thus favoring, from an evolutionary point of view, survival conditions.
In this context, one of the relevant questions is how the perturbations to the modulatory mechanism involved in the adaptive response result in an excessive and inappropriate state of fear and anxiety.
Associative learning related to the emergence of a long-lasting fear memory is critically implicated in the pathogenesis of anxiety disorders, including post-traumatic stress disorder, phobia, and panic. Consonant with such a view, most of the symptoms of these psychiatry entities are due to the persistence and the re-experience of traumatic memories.
Consequently, understanding the neurobiological changes associated with the formation of long-lasting fear memory under particular negative emotional states is relevant for the comprehension of the underlying mechanisms involved in the occurrence of traumatic and persistent memories, as well as for the rebuilding of potential therapeutic tools that could reestablish the adaptive dynamic of the fear memory trace.
In this chapter, we focus on the relevant outcomes observed in animal models of fear learning and memory and their interaction with stressful experiences, along with the observations performed in humans suffering the psychiatric illnesses previously mentioned.
Keywords
StressFear MemoryPsychiatry DisordersStructural PlasticityIntroduction
The formation of a long-term memory has a crucial role in the adaptive behavioral repertoire of different organisms to subsist in a complex environment and to anticipate future encounters with potential threat events. Following this line of reasoning, fear learning constitutes the mechanism that promotes, from an evolutionary point of view, survival conditions [1]. However, fear-promoting experience might lead to a negative emotional state [2]. This state is evident in individuals suffering from anxiety disorders such as phobia, panic disorder, or posttraumatic stress disorder (PTSD) in which the characteristic symptomatology is the re-experiencing of the aversive events, the avoidance of such events, and hyper-arousal.
Classically, one of the animal models widely used in the study of fear memory formation is Pavlovian contextual fear conditioning. In this, an animal learns to associate an unconditional stimulus (US; e.g., electric shock) with another stimulus that has no biological relevance by itself (conditional stimulus [CS]; e.g., light, sound, context). Once the memory is formed, simple re-exposure to the CS elicits a fear response [3]. It is important to highlight that the term “fear” used for animal models refers specifically to the measurable behavior induced by the threat [4]. The classic behavioral output that is evaluated as fear response is freezing, characterized by a crouching position with the absence of any movement except for those which are respiratory-related [5].
One of the main questions in the study of neuroscience is how the organism processes and stores any biologically relevant information for survival purposes.
In this respect, any situation or stimulus that represents a threat or any other potentially dangerous stimuli is processed by different and particular brain areas [6]. Thus, it is postulated that neurobiological changes induced by threat experiences, together with those associated with processes of acquisition/consolidation of the fear memory, underlie the psychopathologies mentioned above.
The Dynamic Fear Memory
As stated several times, “…we are mainly the consequence of our past experiences….” In other words, memories shape our identity and critically influence our emotional reactions to the changing environment. Thus, the different situations to which an organism is exposed along its vital cycle underlie its individual characteristics. Learning and memory processes are built on the basis of such a statement. During this cycle, the organism acquires diverse behavioral and biological responses to cope with future threat events.
The concept of learning and memory has received a dedicated analysis through the history of science. Both behavioral and neurobiological scientists have built the foundations of the actual concepts, nevertheless always under continuous debate and far from achieving a serene consensus.
From a cognitive “psychological” framework, learning refers to the acquisition of information about the environment, with the subsequent formation of a memory trace [7].
From a “neurobiological” point of view, this term refers to the neuronal change that encodes the memory trace.
In relation to memory, the most common definitions refer to the “lasting changes in the behavior of an individual as a result of their past experiences.” Currently, the most widely used and accepted concept of memory is of the “internal representation.” Basically, this concept refers to the structured information from previous experiences encoded on particular neuronal circuits [8]. Therefore, memory is the retention over time of experience-dependent internal representations that could take control of behavior [7].
From the point of view of memory as remembering, Tulving and Thompson argue that memory is similar to the perception, in the sense that it involves understanding of the current stimuli based on past experiences. Consequently, a new memory to be remembered in a meaningful manner should be consolidated within a related preexisting memory [9].
The Different Phases of Fear Memory
A large body of evidence has maintained that following an initial learning experience, the recently acquired information is in an unstable state, which is stabilized by a process termed “consolidation.” This process is commonly addressed at two levels of description and analysis: the cellular/synaptic level and the brain system level. Synaptic consolidation refers to the post-encoding transformation of the information acquired into a long-term form at local synaptic and cellular nodes in the brain circuitry that encodes the memory trace. System consolidation refers to the temporal post-encoding reorganization of long-term memory over distributed brain circuits. In this work, we focus on the synaptic consolidation since system consolidation is beyond the scope of this chapter. In contrast to the view that following consolidation memory is static or fixed, considerable behavioral and neurobiological evidence now indicates that memory is quite malleable. In fact, memory recall by filing a reminder stimulus (a key or signal associated with the original learning) can induce an additional unstable phase requiring an active process to stabilize such a memory trace again. This process has been defined as reconsolidation, and has been proposed as an important component of long-term memory processing.
Currently, it is well accepted that memory is primarily a dynamic encoding process. To understand the mechanisms involved in the formation of long-term memory, it is essential to elucidate the nature and the temporal evolution of the biological changes that accompany the process of acquisition, storage, retrieval, and reconsolidation [7].
Memory Consolidation
In 1900, Müller and Pilzecker, performing studies on human behavior, found that memory of recently learned information was interrupted by the learning of a different piece of information shortly after the original one [10]. This finding suggested that the process underlying new memories initially remains in a fragile state, and that consolidation occurs over time. Meanwhile, several years earlier, the French psychologist Théodule-Armand Ribot had already reported that the probability of amnesia by brain trauma was greater for recent than for more remote memories [11]. These studies, along with many others which came years later, constituted the main foundation of the phenomenon of retrograde amnesia, giving rise to two memory models, the synaptic and the systemic consolidation models.
Synaptic consolidation includes cellular and molecular phenomena accompanying changes in synaptic efficacy after a learning experience [8, 12]. In fact, learning experience leads to the activation of intracellular signaling cascades, resulting in post-translational modifications, the modulation of gene expression and the synthesis of gene products leading to changes in synaptic efficacy. According to this model, the higher the progress of these processes is, the lower is the probability that a memory change occurs by applying an amnesic treatment [13]. Different pharmacological, genetic, and lesion strategies have made possible to clarify, in part, the neuronal and the molecular processes underlying memory consolidation [14]. Diverse molecular events closely associated to synaptic consolidation have been used to describe this phenomenon. Accordingly, researchers have described the involvement of different glutamate receptors, transcription factors [cAMP-responsive element binding protein (CREB), transcription factor CCAAT enhancer binding protein (C/EBPβ), c-fos, nuclear factor-kappa B (NF-kB), Zif-268, and brain-derived neurotrophic factor (BDNF)], and kinases (protein kinase A, PKA). In addition, other effectors such as activity-regulated cytoskeletal protein (Arc) and tissue plasminogen activator (t-PA) were also used [15–17].
Memory Retrieval
Memory retrieval is a complex process by which the previously acquired information can be used. In other words, it is the only evidence that memory exists.
From a cognitive framework, Endel Tulving proposed that the retrieval can be subdivided into two separate processes: reactivation and conversion. Reactivation (Tulving called this “ecphory”) refers to the process whereby retrieval information, provided by a cue, is correlated with information stored in a memory trace. The product of this correlation enables the second stage of retrieval: conversion. This stage covers the subjective experience of reminder and the corresponding memory performance [9]. From a neurobiological point of view, reactivation is also considered as the initial stage of retrieval, and corresponds to the activation of the neural systems that encode the memory trace. Operationally, this phase results from the re-exposure to the information acquired during training. Thus, the reactivation involves the passage of a memory from an inactive to an active state, which in some instances can be manifested behaviorally [18]. In this context, the behavioral performance should not serve as a defining attribute for relevant memory formation.
As discussed below, reconsolidation researchers propose that retrieval of consolidated memories can destabilize the memory and thus induce an unstable state. In order to persist, a re-stabilization process termed reconsolidation is required, which is dependent on new protein synthesis [13, 19] and permits memory updating [20, 21]. Accordingly, memory retrieval has been used primarily as a tool to study the reconsolidation process. However, it is appropriate to reconsider retrieval as a process by itself, that is, not only as a preliminary stage of reconsolidation, but as a different stage of memory processing that plays a key role with respect to preexisting memories.
As previously noted [13, 22], retrieval is not a passive readout of prior experiences; rather, it can turn memory into a transient plasticity enabling dynamic modifications of the established memory trace [23–26]. However, it should be noted that retrieval does not always lead to a reconsolidation process [27]. Under certain circumstances, for instance, new information can be updated into the original trace and its strength can be significantly modified [21, 28, 29].
Memory Reconsolidation
It has long been argued that a newly acquired memory irreversibly passes over time from a labile to a stable state where it remains resistant to the effects of any manipulation, pointing to consolidation as a unitary process [20]. As noted above, this standard theory of consolidation was challenged when Donald Lewis revealed post-retrieval amnesia, a phenomenon referred to as “cue-dependent amnesia” [18]. Lewis and colleagues observed that memory was susceptible to the amnesic effects of electroconvulsive shock after the presentation of a signal associated with learning [30].
This additional labile retrieval-induced phase did not receive attention until 1997, when it was described that the retrieval of a well-established spatial memory was dependent on NMDA receptors (N-methyl-D-aspartate) to maintain stability [31]. Then, the “reconsolidation” concept was introduced to explain the process by which previously consolidated memories are stabilized again after retrieval [23]. In recent years, the reconsolidation theory has received greater theoretical attention and further experimental confirmation. This particular memory phase has been shown in a wide range of species (including humans), in different behavioral tasks and brain regions and by using diverse amnesic and memory-enhancing agents [32–37]. For instance, Bustos and colleagues among others demonstrated that systemic administration of the benzodiazepine midazolam after retrieval resulted in memory deficit in a subsequent test [38]. No memory disturbances were observed in the absence of retrieval. Moreover, no interfering effect was noticeable after the reconsolidation/stabilization window was closed. Reconsolidation does not seem to occur every time that a consolidated memory is reactivated. It is more likely to occur when new information becomes available in the retrieval situation. Even more, there are certain conditions where this process either does not appear to occur or is highly limited. These boundary conditions place constraints on the emergence of both retrieval-induced instability and the restabilization process. In other words, when retrieved, and under certain conditions, they can return to an unstable state and need to be reconsolidated to persist. For instance, older [39, 40] and stronger [41] memories, as well as those reactivated for a short period [39, 42] are less susceptible to engage the labilization/reconsolidation process.
Importantly, it has been proposed that memory reconsolidation may serve as an adaptive function. In fact, a malleable phase following retrieval could be critical for maintaining memory relevance in a changing environment, allowing the incorporation of new or additional information and thus enabling an immediate re-calibration of the existing memory [26]. However, as discussed above, it is suggested that retrieval could generate a transient plasticity state during which a memory would be modified and/or updated without requiring a reconsolidation process [27].
Despite the evidence suggesting that memories could be updated after retrieval, it is not entirely clear whether this update depends on the consolidation or reconsolidation process. It has been indicated that reconsolidation occurs only in conjunction with the incorporation of new information, i.e., accumulating findings support the notion that the availability of new information during retrieval is a requirement for the occurrence of reconsolidation [28].
A method for examining the reconsolidation role in memory updating is to identify differential mechanisms of reconsolidation and consolidation (see section “Consolidation vs Reconsolidation”). In this regard, Lee (2010) showed that reconsolidation supports the update of a hippocampal-dependent contextual memory [21].
Thus, understanding the molecular mechanisms of reconsolidation could provide crucial clues about the dynamic aspects of normal mnemonic function. In this context, psychiatric disorders can be characterized by exceptionally robust and persistent emotional memories.
Memory recall can induce a state in which synapses seem to require the synthesis of new proteins, as inhibition of this post-retrieval process results in a degree of amnesia for retrieved memory [32].
With regard to biological events, several transcription factors have been implicated in memory reconsolidation: CREB, NF-kB, EtsLiKe gene1 (ELK1), Zif268, and C/EBPβ [43–47]. Transcription factors are phosphorylated by kinases. The extracellular signal-regulated kinase (ERK) is required for the reconsolidation of conditioned fear [48], object-recognition [49], and conditioned place preference memories [45]. PKA is also necessary for the reconsolidation of conditioned fear memories [50].
As previously suggested, the reconsolidation process is composed of two distinctive and mechanistically different phases; namely, a reactivation-induced destabilization and a subsequent restabilization process. Currently, there is intensive research aimed at identifying the molecular mechanisms involved in the induction of a state from consolidated to labile, essential for the occurrence of memory reconsolidation. In this respect, NMDA receptors containing GluN2B subunits in baso-lateral amygdala (BLA) have been reported to be crucial for memory destabilization. Consistent with the fact that GluN2B subunits are required for memory destabilization, intra-BLA administration of a selective antagonist of this NMDA subtype prevents the instability induced by fear memory reactivation [51, 52]. Furthermore, this particular NMDA receptor subtype is critically involved in the protein degradation by the ubiquitin/proteasome required for memory destabilization after reactivation [53–55]. Consistently, inhibitors of proteasome activity block reactivation-induced destabilization.
Consolidation vs Reconsolidation
Despite its name, reconsolidation is not a faithful recapitulation of consolidation. Although there are common mechanisms at the cellular and molecular level, recent evidence has shown specific ones for consolidation and reconsolidation.
Clear differences between consolidation and reconsolidation are presented in the activation profile of transcription factors. In the dorsal hippocampus (DH), there is a double dissociation between brain-derived neurotrophic factor (BDNF), selectively required for consolidation, and Zif-268, selectively required for reconsolidation of contextual fear memory [46]. In this respect, it has been established that this separation at gene expression level is reflected in parallel and independent signaling pathways upstream of transcriptional activation: the NMDA receptor-ERK1-BDNF path is functional for consolidation, whereas NMDA receptor-inhibitor NFkB-kinase (IKK)-Zif-268 is functional for reconsolidation [57].
Extinction Process
As stated by Dr. Rescorla: “Extinction is one of the most basic findings in the study of Pavlovian conditioning. It is routinely observed that a CS which has previously paired with an US loses its power to evoke the learned response when the US is removed” [58]. Three theoretical models have been postulated for the development of the extinction process:
It is important to remark that the extinguished conditioned response can be observed again at different times after the extinction training has finished through different processes, those which are considered as definers of extinction properties:
- (a)
A mechanism called spontaneous recovery, in which the expression of the extinction decays with time;
- (b)
A mechanism called renewal, in which the original fear response reappears in a different context where the extinction process occurred;
- (c)
A mechanism called reinstatement, in which the return of an extinguished conditioned response is the consequence of the re-exposure to the unconditioned stimulus,
This evidence supports the notion that extinction is a new learning process that inhibits or reduces temporarily the original association [3]. Thus, as with other learning processes, the extinction takes place in three steps: acquisition, consolidation, extinction retrieval or recall [62].
The acquisition of the extinction is based on the initial learning in which the CS does not predict a threat, thus the conditional response decrease. Afterwards, different physiological and molecular events stabilize the extinction memory through the consolidation phase. Subsequently, the presentation of the conditional stimulus promotes the recall of the extinction, which would be expressed as the suppression or attenuation of the original conditioned response.
With regard to the signaling pathways and network mechanisms involved in the formation of the extinction memory, different results have emphasized a critical role of NMDA receptors and particularly the NR2B subunit in the lateral amygdala (LA) and ventro-medial pre-frontal cortex (vmPFC). In this way, the antagonism of NMDA receptors using MK-801 [63], AP-5 [64, 65] or the NR2B blocker ifenprodil [66] prevents the formation of the extinction memory. Interestingly, the administration before or after extinction of a partial agonist acting at the glycine-recognition site of the NMDA receptor, D-cycloserine, in a fear-potentiated startle paradigm, and fear conditioning facilitates extinction [67].
In relation to kinase pathways, many of them including PKA, MAPK, PI-3-K, and CAMK are also involved in extinction memory. In this respect, an upregulation of phosphorylated MAPK/ERK within the BLA was observed in the auditory fear-conditioning paradigm in a time-dependent manner at late extinction periods [68]. Similarly, the facilitating effects of D-cycloserine on fear extinction and MAPK activation was prevented by the blockade of MAPK in BLA (PD98059 and U0–126) or PI-3-Kinase inhibitor (wortmannin) preventing also the PI-3-K activation [69].
Stress
The word “stress” was introduced in physiological and biomedical research by the Hungarian-Canadian scientist Hans Selye in 1936 to describe a non-specific syndrome in laboratory animals in response to different harmful agents. Later, the stress definition was modified to describe a potential or actual threat to homeostasis, imposed by internal or external adverse forces, called “stressors.” Homeostasis is restored by a complex repertoire of adaptive behavioral and physiological responses of the body, named “stress response” mediated by the “stress system.”
With the increasing number of publications in the field of stress research, the use of a conventional definition of stress such as “the body’s response to any actual or threatened homeostasis disturbance” has become evident. This definition brings considerable problems, since it argues that any threat to homeostasis is a stressor [70]. In conclusion, it is inappropriate to use the term “stress” for conditions ranging from the lightest challenging stimulation to severely aversive conditions [71].
Stress, as a complex process, involves several components: the stimulus or stressor, cognitive appraisal of this stimulus, which assesses how far it can be considered as a threat, and the behavioral and physiological response or the stress response [72]. Clearly, at the present time, the main problem with the stress concept is that most existing definitions include only some of these components.
In recent years, the introduction of the terms “controllability” and “predictability” to stress concept has led to a more precise definition of this phenomenon. In this context, it is considered that the induction of a stress condition depends more on the extent to which the stimulus can be predicted and controlled than the physical nature of the stressor [73].
Koolhaas and co-workers (2011) proposed an interesting characterization of stress: “the term should be limited to conditions where an environmental demand exceeds the natural regulatory capacity of an organism, particularly in situations which include the lack of prediction and control” [71]. Thus, this more precise definition could avoid confusion with normal physiological reactions that are required to sustain the behavior.
There is a close association between stress and different psychiatric disorders including PTSD, depression, and schizophrenia. The hypothesis under consideration is that the symptomatology observed in those patients represents the behavioral manifestation of the stress-induced changes in brain structure and function. Consistent with this idea, the exposure to traumatic events results in acute or chronic neurobiological changes in specific brain areas involved in such responses, with the possibility of suffering long-term changes in brain circuits [74].
The Brain Circuit Involved in Fear Memory Process and Stress
The brain does not learn or remember as a unitary simple structure: different central circuits are specialized and code different types of information [75]. The critical information is controlled by a hierarchical neuronal system that determines the efficacy of the behavioral response and in consequence, the dynamic adaptation to the threatening situation [3]. The majority of the researchers propose a processing model for fear memories in which the amygdaloid nucleus, the hippocampus and the medial-PFC play differential but pivotal roles. In the same way, each brain area presents subdivisions with particular functional characteristics [76, 77]. It is important to remark that even though other brain areas participate in the processing of fear memories, advances in the knowledge of the brain circuits in fear memory were mostly achieved in these brain areas.
Under this scenario, a vast literature suggests that the amygdala is essential for the formation of emotional memories [78], and it is also critically involved in stress-induced anxiety and fear reaction [79–82]. This pivotal contribution to fear memory and stress implies that these processes can be influenced by each other and thus, be interrelated. Thus, the amygdala is a major contributor to the interaction between stress and fear memory [78, 83], which is consistent with studies reported in humans. In this respect, memory deficits in amygdala-lesioned patients [84], and functional imaging experiments, revealed a heightened amygdala activity during emotional encoding [85], supporting this notion.
The amygdaloid complex is located in the anterior medial portion of the temporal lobe, and is comprised of a very heterogeneous nuclear group divided on the basis of cytoarchitectonic, histochemical and immunocytochemical studies [86]. Considering the involvement of these nuclei in fear memory, the BLA, the central (CE), and the intercalated (ITC) cell masses are the principal sub-areas well defined in such a process.
The synaptic information from thalamic/somatosensory inputs and cortical and hippocampal afferents converges into the BLA, thus resuming in this sub-nuclei the information of the CS and the US from the conditioning paradigm, which promote synaptic plasticity events for the learning and memory processes [78]. In this way, the BLA integrates the information that makes it possible to distinguish innate and learned environmental threats, playing a fundamental role in prompting relevant actions for survival purposes. The CE nucleus is the principal efferent area from the BLA, particularly for emotional responses and associated physiological responses [87] which control the expression of the fear response, including behavioral, anatomical, and endocrine responses through efferent projections, mainly to lateral hypothalamic, paraventricular nucleus of the hypothalamus, the periaqueductal grey area, and motor areas of the brainstem [78].
The hippocampal formation has a fundamental role in different processes of contextual fear memory, stress, and anxiety. Behavioral evidence as well as neuroanatomical studies have demonstrated clear different conclusions about efferent and afferent connectivity throughout its septo-temporal axis [88, 89]. Thus, the dorsal pole of the hippocampus (DH) would play a more selective role in the spatial representation of an event, while the ventral region (VH) would contribute to the modulation of fear and anxiety [90, 91].
Related to the spatial and contextual processing of the information, the DH might be downstream from the BLA neuronal activation involved in contextual fear [92]. However, the BLA rarely presents direct projections to DH, its principal interconnection being through the entorhinal cortex (EntC), mainly the dorso-lateral portion (dl-EntC) [86], which constitutes the principal source of afferent excitatory neurotransmission to DH [93]. Strictly, layer 2 of the dl-EntC receives dense excitatory projections from BLA, and in turn sends dense excitatory efferent through the perforant path to the dentate gyrus of the hippocampus.
The PFC is a brain area involved in the consolidation as well as in the expression of conditioned fear, and the acquisition, consolidation, and expression of extinction memory. Considering the cellular types and the pattern of its organization, the PFC can be divided into four regions taking into account the dorsal to ventral distribution: medial prefrontal, cingulate anterior, prelimbic (PL) and infralimbic (IL) [94]. As with the majority of the neocortical areas, the cellular organization presents six layers (I to VI), where layer VI is the deepest one and layer I is the closest to the piamatter. Layers II/III and V/VI present pyramidal neurons with apical dendrites that project to layer I.
Different evidence from neuronal tracings and electrophysiological studies in PFC has revealed the existence of PL and IL interconnections, with functional physiological consequences when such connection is interrupted [95].
Stress, Fear Memory, and Psychopathology
It is important to make an observation with regard to the behavioral manifestations observed in psychological pathologies. In PTSD patients, it is commonly assumed that by definition a psychologically traumatic event caused the behavioral manifestation, and by consequence that any biological abnormality found to accompany the PTSD has to be traumatically induced. However, we should also consider that any exposure to a threat event which the individual fails to cope with would increase the risk of developing the psychological pathology [74].
It is known that patients suffering from PTSD, anxiety, or panic disorder present alterations in memory function [96] in which preclinical and clinical studies have shown changes in the brain circuitry critically involved in the memory process [74, 97]. Most of the psychophysiological studies, which include measures of heart rate, skin conductance, facial electromyogram (EMG), and cortical electroencephalographic event-related potentials (ERPs), showed alterations in those measures in PTSD patients when they were exposed to idiographic trauma cues [98]. Interestingly, these parameters decreased notably in an imagery test in patients that received post-trauma propranolol, a beta-adrenergic blocker that has been found to attenuate the consolidation of fear memories [99] compared to placebo.
In animal studies, it has been found that chronically stressed rats presented critical damage in CA3 pyramidal neurons (cornu ammonis 3) of the hippocampus, which was also associated with high levels of corticosterone, decrease of the neurotrophic factor BDNF, and inhibition of the neurogenesis [100–104]. Interestingly, in the same way as in this animal model, structural MRI studies in the hippocampus of PTSD patients revealed a reduced volume of this brain area. Whether smaller hippocampal size is a result of trauma exposure or rather represents a risk factor for PTSD remains to be determined. However, it has been suggested that the hippocampal volume serves as a pre-trauma risk factor for this pathology [74]. From a functional perspective, an interesting study performed in adult women revealed a functional deficit of this brain area that is associated to the failure to recall extinction memory [105].
Considering the cortex, particularly vmPFC and dorsal anterior cingulate cortext (dACC), a lower volume of such prefrontal regions has also been reported, but in contrast to the observations made in the hippocampus, such cortical reduction would represent an acquired feature of PTSD rather than a pre-existing vulnerability [106]. From a functional perspective, the activation of dACC is higher during fear conditioning in PTSD patients in comparison to control individuals [107]. Considering a critical role of these brain areas in fear learning and expression of the fear, such functional abnormalities are positively associated with severity of symptoms [107].
In relation to the amygdala, functional neuroimaging studies have revealed an exaggerated activation in response to trauma-related stimuli as well as generic ones in PTSD patients in comparison to control individuals [108]. This is in relation to the principal role that this brain area presents in the detection of threat, fear learning, fear expression, and heightening memory for emotional events [78].
The use of an animal model for these critical symptoms of PTSD, anxiety, panic disorder, or phobia has revealed that in addition to the hippocampus, the amygdala and the prefrontal cortex mentioned above were also implicated in the neuronal circuitry involved in stress and fear memory [109]. Lesion studies have demonstrated that the medial prefrontal cortex modulates emotional responsiveness through inhibition of amygdala function [110]. Studies show that neurons of the medial PFC play an active role in inhibition of fear responses that are mediated by the amygdala [111, 112]. Conditioned fear responses are extinguished following repeated exposure to the conditioned stimulus [in the absence of the unconditioned stimulus (aversive; e.g., electric shock)]. This inhibition appears to be mediated by medial prefrontal cortical inhibition of amygdala responsiveness. Animal studies have also shown that early stress is associated with a decrease in branching of neurons in the medial PFC [113, 114].
As mentioned, there is wide consensus that stress promotes the emergence of aversive motivated memories, including fear memory. Our laboratory has observed in rats that exposure to a single stressful event facilitates the encoding of contextual fear memories [83] and the induction of neural plasticity such as long-term potentiation (LTP) in BLA, a cellular model proposed to be implicated in fear-memory formation [83]. In the same way, the interaction between an aversive experience and an established fear-memory trace result in a robust and persistent fear memory [79, 80]. This stress-induced promoting influence was shown to be prevented by midazolam (MDZ, a positive modulator of GABAa receptors) intra-BLA infusion before stress exposure. Such influence is related to the modulation of the GABAergic transmission in this brain area, since intra-BLA bicuculline, a GABAa blocker, introduced just before the encoding of the fear memory mimicked stress-induced facilitating effect on fear-memory formation [79, 83].
Similarly, an identical environmental challenge promoted exaggerated behavioral responses to new encounters with discrete aversive stimulus not previously related [82, 115]. Additionally, a similar stressful experience prior to fear memory encoding resulted in a memory trace that was insensitive to the interfering effect of MDZ on fear-memory reconsolidation, suggesting that previous stress can restrict the destabilization and the engagement of reconsolidation in later retrieval sessions [116, 117]. In support of this view, stress was seen to impede the elevation of both the expression of Zif-268 and the GluN2B sites in BLA, two molecular markers of the labilization/reconsolidation process, following reactivation [116]. All together, this evidence indicates that stress exposure prevents the onset of destabilization/reconsolidation process following reactivation. Hence, highly arousing experiences are determinant for the subsequent emergence of reconsolidation of the memory trace following reactivation.
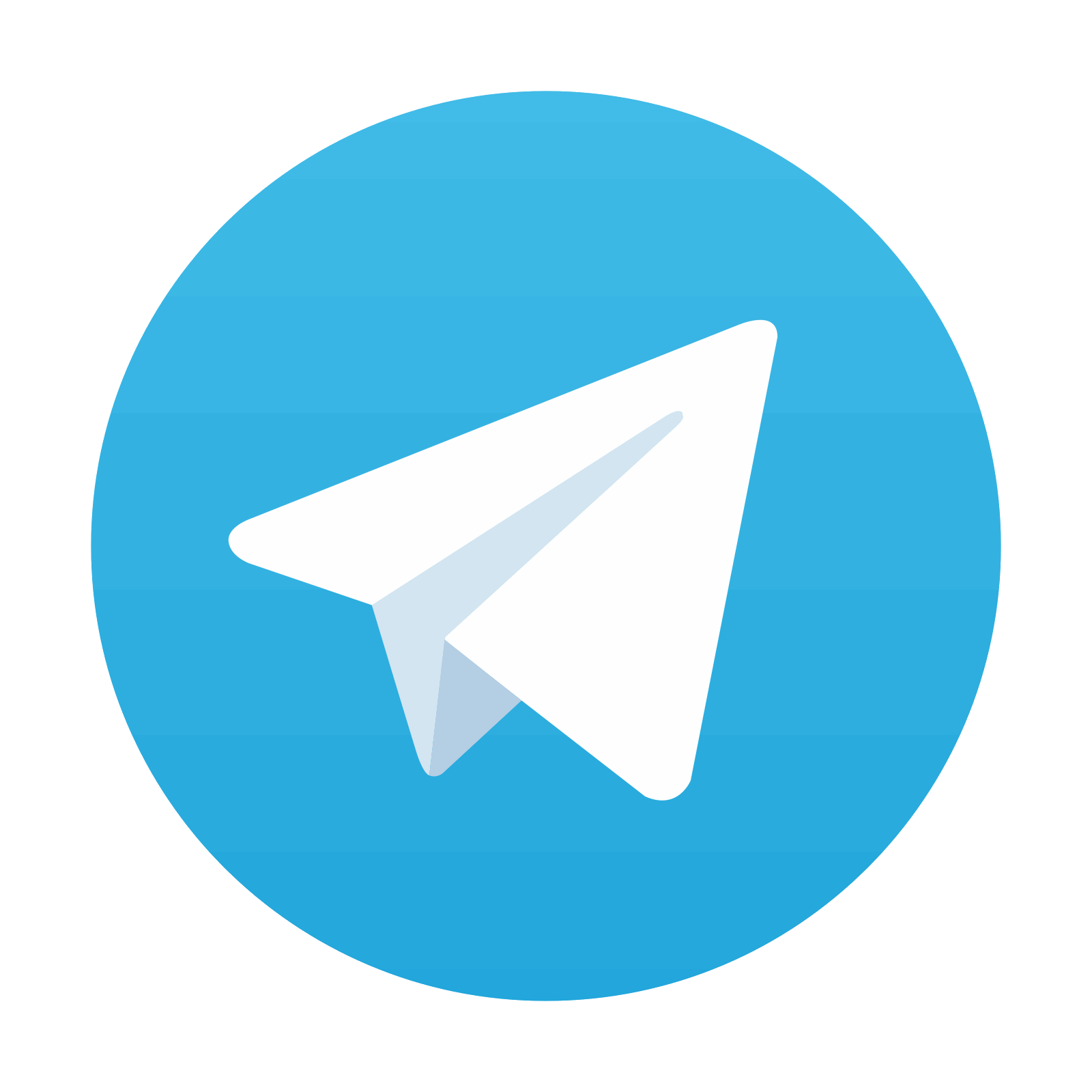
Stay updated, free articles. Join our Telegram channel

Full access? Get Clinical Tree
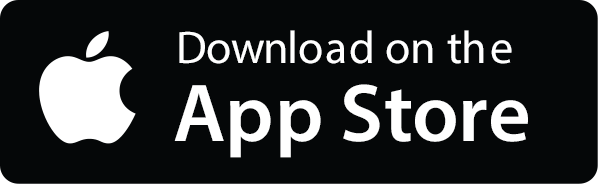
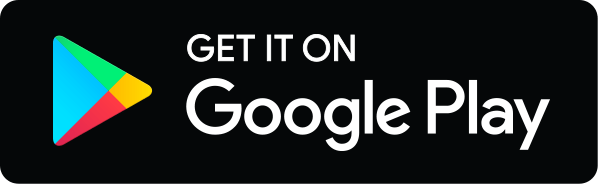