Figure 83.1. Proposed presurgical approach to pharmacoresistant epilepsy due to FCDs. (MRI, magnetic resonance imaging; VEEG, video EEG monitoring; PET, positron emission tomography; SPECT, (ictal) single-photon emission computed tomography; MEG, magnetoencephalography.)
IMAGING CHARACTERISTICS OF FOCAL CORTICAL DYSPLASIAS
MRI techniques have provided a noninvasive window for the identification, localization, and some definition of the extent of some forms of FCDs.
FCD type 1: Nonspecific MRI changes were described for type 1. These include white matter volume reduction and subtle increased signals on fluid attenuated inversion recovery (FLAIR) and T2-weighted images. However, MRI is normal in the majority of patients with type 1 FCD (12,13). Nevertheless, it is important to seek very carefully for asymmetries in the cortical–white matter interdigitations, or in the white matter volume, because subtle changes may prove relevant and aid decision making (Fig. 83.2).
Figure 83.2. Two-year-old girl with frequent partial seizures, secondary generalization, and severe developmental delay. Sagittal (A) and axial (B) MRI sections showing a grossly dysplastic lesion in the right frontocentral region. Ictal EEG (C) suggested right frontal seizure onset. Following extensive frontocentral resection, the patient was seizure free for 30 months, when attacks recurred in the form of frequent startle-induced seizures with drop attacks. There was dramatic psychosocial regression. In (B), note asymmetry of the white matter in the posterior quadrant, suggesting that the lesion was more considerably extensive than previously thought. Histopathology was FCD type 1 (D).
FCD type 2: The most commonly reported abnormalities in FCD type 2 consist of focal changes in the cortical thickness, signal increase (mainly on FLAIR and T2-weighted sequences), and gray–white matter blurring, and in type 2b, the additional presence of a “transmantle” sign (a linear track of increased signal, extending from the periventricular region to the cortical mantle) (12–16). Recent observations show that type 2b dysplasia may show two distinct imaging patterns: (i) Mild to moderate FLAIR signal increase associated with increased thickness of the gray matter. These lesions tend to occur in the depth of sulci, in particular the precentral sulcus, superior frontal sulcus, and the anterior extent of the sylvian fissure (frontal operculum) (17). (ii) A second group of balloon cell–containing FCD lesions is characterized by a “tuber-like” or “tumor-like” dense FLAIR signal increase in the center of the pathologic lesion surrounded by areas of variable FLAIR signal intensities (18), thickening of the cortical mantle, and gray–white matter blurring in association with transmantle signal increase pointing to the periventricular region (14,19,20). Less commonly described abnormalities such as cortical dimple, and hemispheric atrophy have been described in type 1 FCDs (21).
FCD type 3 lesions share the typical features of the associated lesions (HS, tumors, vascular malformations, scars) (22).
EPILEPTOGENICITY OF FCDS
The intrinsic in situ epileptogenicity of FCDs was previously reported by (4) using intraoperative electrocorticography (ECoG). Ictal or continuous epileptogenic discharges were mostly recorded from electrodes overlying dysplastic gyri. Direct cortical recordings demonstrated in situ epileptogenicity in FCD types 1, 2a, and some type 2b lesions (23,24) and showed various types of interictal epileptic activities (ranging from isolated spikes, polyspikes, repetitive spiking, to paroxysmal fast activities) and multiple ictal epileptic patterns (24). Some reports seem to suggest possible specific epileptic patterns in FCDs type 2, particularly repetitive, virtually continuous spikes (4,25,26). More recent data further suggested the in situ epileptogenicity of FCD through the recording of high-frequency oscillations (27).
The specificity of some interictal patterns as possible predictors of the area of ictal seizure onset has been reported, and focal continuous rhythmic discharges, repetitive spiking, and paroxysmal fast activities have been suggested as possible predictors of the ictal onset zone and early postoperative seizure outcome after resection of the area of focal electrical abnormalities (28).
We previously showed a differential distribution of the epileptic activities in balloon cell–containing lesions (type 2B) that display “tuber-like” or “tumor-like” high FLAIR signal intensities. The subregion within the balloon cell–rich FCD (mainly at the center of the MRI tuber-like abnormality) shows the least epileptogenicity, while the surrounding dysplastic cortical area containing few or no balloon cells (FCD type 1 or more often 2A) shows evidence of significant in situ epileptogenicity (24). On the other hand, deep sulcus balloon cell–containing lesions display intrinsic epileptogenicity (within the MRI-identified abnormality).
FUNCTIONAL STATUS OF FCDS
As a significant number of focal dysplastic lesions (FCDs) preferentially involve the frontal and temporal lobes, the issue of functional mapping and its potential overlap with epileptogenic cortex is the main challenge in the process of presurgical management of these patients (28). Dysplastic cortex has been shown to retain motor, sensory, or even language-related functions (29). Various electrical stimulation studies have shown that the dysplastic neocortex may conserve temporal and frontal language sites (30), but may display atypical motor homunculi (31,32). These studies demonstrate that function in dysplastic cortex could be preserved but may be reorganized and differences in functional expression may be dependent on the histopathologic subtype and spatial extent of the FCD. Some evidence is also provided by surgical intervention in high-functioning areas, in which a complete lesionectomy did not lead to motor deficit (33). What can be said, however, is that in FCD 2b, the areas with the brightest signal changes most likely do not contain functions (and are less epileptogenic!) (33). These data lead to this rather provocative (and to a large extent hypothetical) question: Could incomplete resections of balloon cell-containing regions be an option considering their lack of epileptic potential and their possible protective effect against the seizures?
PREOPERATIVE EVALUATION AND SURGICAL MANAGEMENT OF PATIENTS WITH FCD: DIFFERENT SCENARIOS, VARIABLE APPROACHES
The precise anatomical localization and mapping of eloquent cortex and its relationships with a well-defined epileptogenic area are the most important components of any presurgical evaluation. They will facilitate the surgical planning and optimize the chances for a safe resection of the epileptogenic region (thus maximizing the chances for seizure freedom and minimizing the risks of neurologic deficits). Based on our current knowledge regarding FCDs, we suggest the following processes of presurgical evaluation and surgical management in patients with pharmacoresistant epilepsy.
The Preoperative Identification and Localization of FCD Lesion
MRI remains the gold standard and the most sensitive noninvasive window to the brain anatomy in the process of investigating the cause of epilepsy. As discussed above and elsewhere in this book, the identification of a lesion significantly enhances surgical candidacy, simplifies presurgical workup, and often improves seizure outcome. Furthermore, high-resolution MRI may help in the formulation of a hypothesis on the pathologic subtype of FCD (34). Interestingly, despite all advances in MRI, there remains a significant number of patients with FCDs (in particular FCD type 1) whose MRIs remain normal. A recent study found that more than 40% of patients with MRI-negative epilepsy harbor pathologic-confirmed FCD, the majority FCD 1 (35). However, apparently normal MRIs of children with severe epilepsies and mental retardation, with focal or multifocal discharges associated with secondary bilateral synchrony, should be examined with utmost care, seeking for asymmetries in the cortical–white matter interdigitations or in the white matter volume. Subtle changes may be a clue to a specific type of FCD type 1 that deserves aggressive surgical management (34,36,37).
Additional postprocessing techniques such as voxel-based MRI postprocessing using a morphometric analysis program (MAP) may help in the identification of some of these lesions: MAP abnormalities were found in 48% of the MRI-negative epilepsy patients (38).
Localization of the Epileptogenicity, Definition of Its Extent and Functional Mapping
Video electroencephalography (VEEG) would help in the identification of the network structures that may be involved in seizure generation and progression (through analysis of the recorded seizure semiology), therefore leading to the formulation of a clear anatomoelectroclinical hypothesis. Further validation of the anatomic hypothesis is achieved through imaging (the identification of lesion on MRI), with or without metabolic imaging (including FDG-PET hypometabolism that may point to focal regions of cortical dysfunction). Other studies that may include ictal SPECT (SISCOM), MEG, and EEG-fMRI would further point to areas of dysfunction within a network or identify the three-dimensional localization of interictal epileptogenic regions, respectively.
Noninvasive studies achieve the goal of identification of the epileptogenic area and its possible anatomical cause in a sizable number of surgical patients (39–42). In many, however, formulation of a clear anatomoelectroclinical (AEC) hypothesis may not be possible, or an AEC hypothesis is generated but the exact location of the epileptogenic area within the network, its extent, and/or its overlap with functional (eloquent) cortex remain unclear (43–46).
The localization of functional areas in the brain, mapping of their extent, and their potential spatial overlap with the epileptogenic zone (EZ) are essential parts in the process of developing an adequate and individualized resective surgical strategy (39,47,48). As focal cortical dysplastic lesions (FCDs) are differentially located in the frontal and temporal lobes (therefore in potentially eloquent cortex), an understanding of the functional status of the involved region(s) and its anatomical and pathologic correlations are essential (39,49).
The pathologic differences and variable imaging characteristics in addition to the lack of known in situ biomarker(s) for the localization and mapping of the intrinsically epileptogenic dysplastic cortex constitute major challenges in the presurgical evaluation and surgical treatment of patients with FCDs (50,51). The main indications for an invasive evaluation in focal pharmacoresistant epilepsy due to suspected FCD (with the main purpose of direct cortical recording) are to address the main challenges and limitations of various noninvasive techniques. We recently recommended that an invasive evaluation should be considered in any one of the following cases (18):
1. The MRI does not show a cortical lesion in a location that is concordant with the electroclinical/functional hypothesis generated by the VEEG recordings (so-called MRI-negative cases).
2. The anatomical location of the MRI-identified lesion (and at times the location of a clearly hypometabolic focal area on PET) is not concordant with the electroclinical hypothesis. These include selected cases of deeply seated brain lesions such as deep sulcal lesions.
3. There are two of more anatomical lesions with the location of at least one of them being discordant with the electroclinical hypothesis, or both lesions are located within the same functional network, and it is unclear if one (or both) of them is (are) epileptic.
4. The generated anatomoelectroclinical hypothesis (MRI-negative or MRI-identifiable lesion) involves a potentially highly eloquent cortex (52–54).
In these instances, an invasive evaluation would lead to the formulation of a resective surgical strategy. The recommendation for an invasive monitoring and its type should be made during a multidisciplinary patient management meeting that includes neurologists, neurosurgeons, neuroradiologists, and neuropsychologists. Areas and networks of coverage/sampling should be determined based on a well-formulated AEC hypothesis (based on the results of all available noninvasive studies).
For this purpose, one intraoperative recording technique (ECoG) and two extraoperative invasive methods with chronic recordings—subdural grid electrodes (SDG) and stereotactically placed intracerebral electrodes (SEEG)—have been used to accomplish these goals.
Intraoperative recording is a limited option as it only provides information restricted to interictal activity. However, this type of recording may prove very useful when continuous repetitive discharges are seen from involved cortex, as these have been shown to significantly correlate with the ictal onset zone (4,49,55). Furthermore, acute ECoG with carbon-ball electrodes may be performed in sequential stages during the resection, allowing electrical activity from depths of gyri and adjacent cortical regions to be repetitively probed (55). The influence of anesthetic agents on EEG activity may alter thresholds of afterdischarges and motor responses (52), although this has not been a universal observation and is often not relevant for the localization purposes of the study. Additionally, intraoperative language mapping often requires a cooperative patient who can tolerate being awake during surgery under local anesthesia. This is particularly difficult in the pediatric population.
Extraoperative mapping with subdural grid electrodes (SDG) has the main advantage of allowing an optimal coverage of the subdural space adjacent cortex with adequate and continuous superficial functional mapping capabilities (32,56–58). A major strength of subdural electrodes consists in the comprehensive anatomical coverage of cortical surfaces therefore allowing accurate anatomical electrical and functional mapping of the areas of coverage. Limitations of SDGs include the inadequate/partial/incomplete intrasulcal and interhemispheric coverage (59) and the need for craniotomy in order to implant. These characteristics are highlighted in the published results on the successes and failures following SDG implantation (60). Previous outcome studies showed that the best results following SDG implantation are achieved in patients with clear cortical lesions (in particular tumors), those who underwent lobar resections, and those patients in whom functional mapping was the main purpose of SDG implantation (49,60). These observations suggest that the best candidates for extraoperative invasive evaluation with SDG are those patients with clear FCD surface lesion(s) (excluding the interhemispheric, cingulate gyrus, deep sulcal, and mesial frontotemporal regions). A modified implantation approach of SDG and manually implanted depth electrodes has been used for the last 10 years at some epilepsy surgery programs. This modified approach has the main advantage of combining the two recording methods and thus allowing for excellent cortical coverage and some sampling of deeper lesions. But this modified approach does not allow extensive network coverage of functional networks and lacks the accuracy of the SEEG methodology.
Extraoperative recording with (SEEG) is indicated in patients with deep-seated lesions or in areas that are difficult to cover with SDG such as the interhemispheric regions (frontal, parietal, and occipital, in particular the paracentral lobule), opercular areas, cingulate gyrus, posterior orbitofrontal areas, insula, and depths of sulci. SEEG takes advantage of the more accurate anatomical presurgical workup and the possibility to stimulate into the depth of the sulcus and the subcortical pathways. There is at times a need for a complementation of the electrical localization with other methods of functional mapping such as diffusion tensor imaging, functional MRI, and intraoperative functional mapping (54). Limitations in SEEG include the need for more expensive testing preoperatively and specialized equipment in the operating room. The major risk of SEEG implantation is the higher risk of intracranial hemorrhage (3%) with associated permanent neurologic morbidity when compared to SDG implantation.
RESECTIVE STRATEGY IN FCD
The main principle that governs epilepsy surgery and in particular resection of FCDs is the “complete” resection of the EZ while preserving normal brain and function. This should be based on a clear definition of the anatomical, epileptic, and functional boundaries of the lesion and an accurate hypothesis regarding the extent of the EZ. Every effort should be made to resect the lesion and the areas of ictal onset (from which ictal epileptic patterns were recorded). This is particularly important in patients with tuber-like FCD type 2b lesions, where an incomplete resection of areas adjacent to the visible lesion may exacerbate the epilepsy and results in acute postoperative refractory status epilepticus (61). What remains a subject of debate is the issue of recorded interictal patterns that are at times spatially distinct from the areas of ictal onset. As previous studies showed that some interictal patterns are highly predictive of ictal onset (such as continuous rhythmic discharges and paroxysmal fast activities) (4,28), we do recommend an extension of the margin of the resection to these areas. The significance of single spikes in the planning of the resection remains unknown, and although every effort should be made to resect these regions, their frequent location in regions that are noncontiguous with the lesion and the ictal onset zone precludes their complete resection.
Surgical strategy involves resection of involved cortical tissue while preserving blood supply. This is best accomplished by using a subpial resection technique to spare cortical vessels that may be passing through to irrigate noninvolved cortex. The involved cortex has often a more firm consistency, which allows surgical resection by following the plane between the softer normal cortical tissue and the firmer dysplastic tissue. In many of the type 2 FCD cases, the dysplastic cortex can be followed down into the white matter as one might follow the root of a plant into the Earth. It remains unknown whether resecting this deeper firm “tail” or “root” is clinically significant. When functional cortex is involved, the surgical approach must take into account the anticipated effect of operating in or near the functional cortex. Risks should be carefully detailed preoperatively so that postoperative expectations are clear. Intraoperative surgical techniques to identify and preserve function are the same as applies in other fields of neurosurgery and include cortical stimulation and surgery under awake conditions. Resection of some functional areas is well tolerated and should be undertaken when necessary and after proper informed consent to effect a cure from seizures. In general, surgery for FCD involves larger resections and outcomes improve when larger pieces of cortex are removed.
A final word is needed on the surgical strategies for children with severe epilepsies and mental retardation, in whom the association of (i) subtle asymmetries of gray–white matter volume (see Fig. 83.2
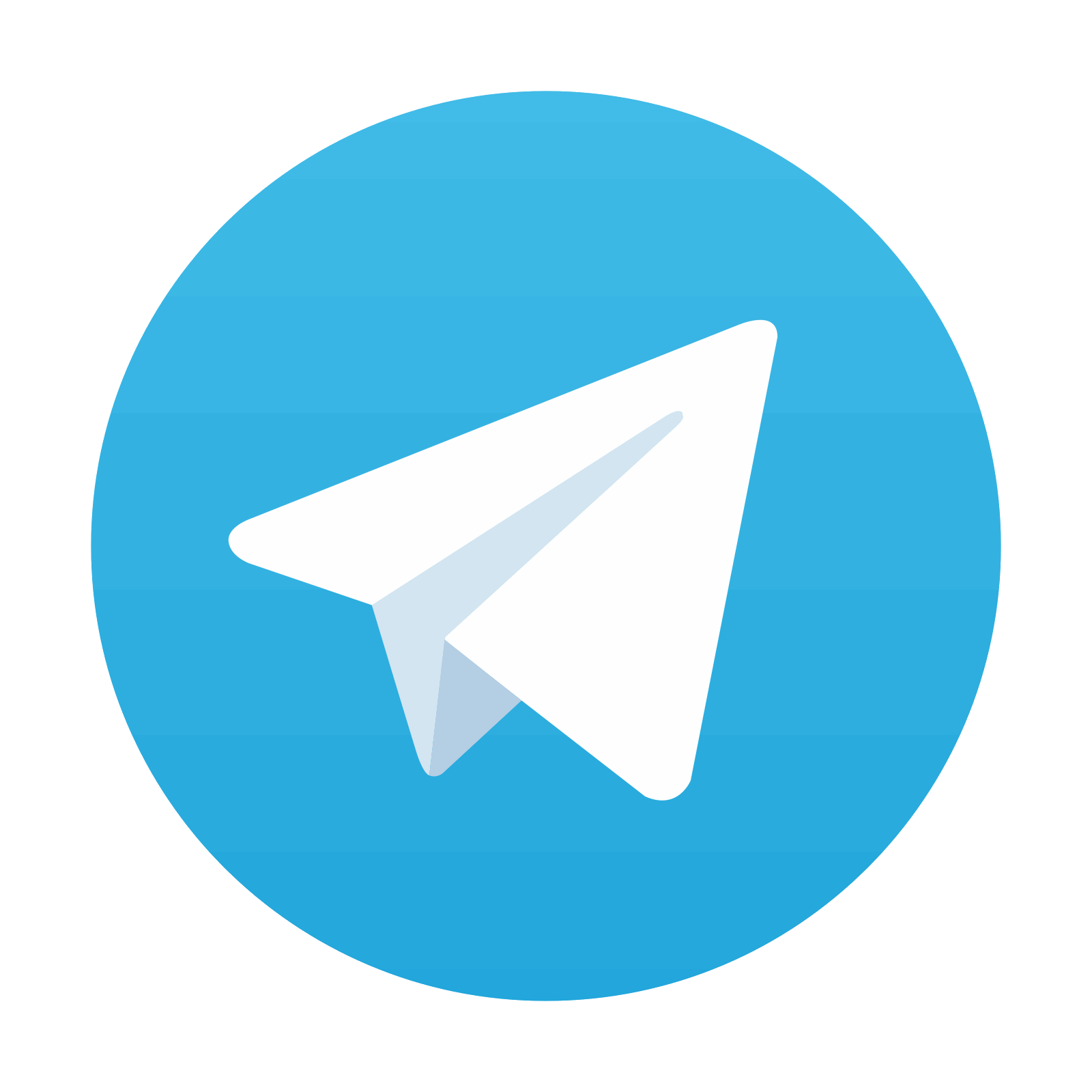
Stay updated, free articles. Join our Telegram channel

Full access? Get Clinical Tree
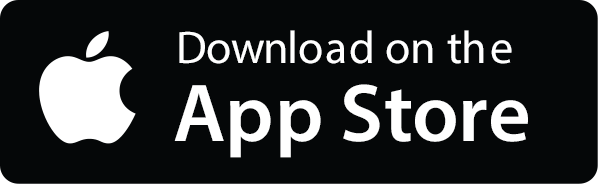
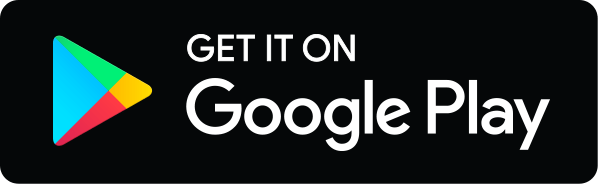