INTRODUCTION
Despite the recent remarkable advances in science that have delivered new pharmaceutical and other therapeutic strategies for Parkinson’s disease (PD) treatment, there remains a select group of patients who may be eligible for surgical therapy. Surgery for PD is most often brought into service when medication complications (motor fluctuations and dyskinesias, or medication-refractory tremor) begin to adversely affect quality of life (1). Based on recent experience, about 10% to 20% of PD patients may be good candidates for deep brain stimulation (DBS) surgery. Current surgical therapies for PD include procedures that aim to reduce or to restore abnormal neuronal activity (lesional or ablative therapies and DBS), and also procedures that may enhance dopaminergic transmission (transplantation and gene therapy) (2). The main focus of this chapter will be DBS therapy.
LESION THERAPIES
HISTORICAL EVENTS
Lesion therapies were first reported for movement disorders in 1942, when Dr. R. Meyers performed a partial caudate and also a pallidofugal fiber resection for control of unilateral tremor and rigidity in a PD patient. He was able to accomplish this procedure without inducing motor paresis (3). Later in 1953, Cooper (4) accidentally ligated the anterior choroidal artery during a pedunculotomy operation in a PD patient and he observed a reduction in tremor and rigidity without loss of strength. Guided by his findings, and convinced of the important role of structures supplied by the anterior choroidal artery (globus pallidus internus [GPi], subthalamic nucleus [STN], thalamus, substantia nigra, red nucleus, and ansa lenticularis), he published a large PD surgical series, revealing the potential clinical benefit that may be achieved by ligating this artery (5). After the introduction of stereotactic surgery by Spiegel et al. (6), different stereotactic techniques were developed to address movement disorders, including leukotomies, cryosurgery, hot wax, or alcohol injections (7,8). Each of these techniques was used to create a brain lesion. In an attempt to improve surgical stereotactic outcomes, Svennilson (9) introduced the thermolesion or radiofrequency (RF) pallidotomy. He heated the uninsulated tip of an electrode to a defined temperature in an attempt to improve Parkinson’s symptoms. In the late 1960s, surgical therapies were temporarily abandoned with the discovery of levodopa (10); however, surgeries resurfaced in the late 1980s and early 1990s with the appreciation of levodopa-induced motor fluctuations and complications (11). Stereotactic RF procedures replaced the former surgical techniques, and have been used successfully for decades with reasonable outcomes in appropriately selected patients (12,13). The introduction of DBS led to an immediate decline in the widespread popularity of lesion therapy (14). Table 47.1 shows significant milestones in surgical therapies for PD.
Though producing a lesion in specific sites in the brain fell out of favor, the potential positive effects of these approaches should not be underestimated (15). Lesion therapies remain a reasonable therapeutic option for a select group of patients. We will provide an update on ablative therapies and address two important issues in lesion therapy prior to our discussion of DBS: (1) How do lesional therapies compare to DBS? (2) What is the current role of other ablative procedures such as the gamma knife (GK) and MR-guided focused ultrasound (MRgFUS)?
PALLIDOTOMY
Stereotactic pallidotomy was introduced in the 1950s by Spiegel and Wycis (16) and it quickly became a popular procedure in PD patients. Subsequently, Hassler and Riechert (17) published results of placing a lesion in the thalamus or thalamotomy. Throughout the 1960s, different thalamic targets were lesioned. The ventral intermediate (VIM) nucleus emerged as the most effective target for tremor suppression in PD and also essential tremor (ET). Finally, subthalamotomy was introduced by Hullay (18) in the early 1970s; however, the resulting hemiballism limited its widespread use. The majority of ablation studies published have been retrospective case series. We will briefly review the use of RF lesions in PD, and will utilize only the available Level I evidence-based studies.
In the first randomized trial, 13 patients were assigned to receive unilateral pallidotomy, and these patients were compared to DBS patients. At 3-month follow-up, there was an improvement of almost 30% in Unified Parkinson Disease Rating Scale (UPDRS) motor scores in the pallidotomy group, and no significant differences with stimulator group (19). In a second study, unilateral pallidotomy was performed in a randomized methodology in 34 advanced PD patients, and the results showed a significant improvement of the UPDRS part III off scores when compared to control group (47 to 32.5 vs. 52.5 to 56.5, p<0.001) at 6-month follow-up, as well as significant improvements in functional status and UPDRS part II follow-up off scores. In addition, the study confirmed a 50% reduction of levodopa-induced dyskinesias (LIDs) in the pallidotomy group. In a third randomized trial, 36 PD patients were assigned to receive either medical therapy or unilateral pallidotomy (21). Compared with the medical group, the surgical group had a significantly reduced total UPDRS score at 6-month follow-up (p<0.001), and improved 23% in section II, and 32% in section III of the UPDRS scale. There were also significantly lower numbers of motor fluctuations and dyskinesia when compared to a medication-only group. In the 2-year analysis, there was a sustained improvement in the surgical group. In the most recent trial, 34 patients with advanced PD were assigned to have unilateral pallidotomy or bilateral STN stimulation (22). At 6 months, the stimulation group had significant greater improvement than the pallidotomy group in the off-state UPDRS motor score (46.5–37 versus 51.5–26.5; p = 0.002). Bilateral DBS was more effective overall in controlling parkinsonian symptoms, which would be expected given the lesion group was limited to a unilateral intervention. In the subsequent 1-year follow-up study, the larger beneficial effects of stimulation were sustained (p = 0.002), but interestingly, both groups reported an additional improvement in the UPDRS part III (23). In summary, all available studies provide clear evidence that unilateral pallidotomy can be an effective treatment for the motor symptoms in advanced PD patients, for reducing LIDs, and also that this benefit may be sustained past 1 year.
| Historical Milestones of Surgical Therapies for Parkinson’s Disease |
Year | Therapy |
1942 | Meyers’ partial caudate and pallidofugal fiber resection |
1947 | Spiegel and Wycis introduced stereotactic surgery |
1950s | Stereotactic techniques: leucotomy, cryosurgery, hot wax, alcohol |
1953 | Cooper’s accidental ligation of anterior choroidal artery in a PD patient |
1954 | Spiegel and Wycis introduced stereotactic pallidotomy |
1957 | Introduction of thalamotomy by Hassler |
1960 | Introduction of RF by Svennilson |
1967 | Discovery of levodopa |
1970 | Stereotactic subthalamotomy introduced by Hullay |
Late 1980s | Reemergence of stereotactic RF procedures |
1987 | Introduction of DBS by Benabid |
1993 | Rand introduced radiosurgery for movement disorders |
Late 1990s | First reports on STN DBS for advanced PD |
2006 | First randomized trial of DBS versus medical therapy by German PSG |
Late 2000s | New targets in DBS (caudal zona incerta, pedunculopontine nucleus) |
2010–present | Introduction of ultrasound thalamotomy for movement disorders Controlled trials for fetal cell transplantation Studies of gene therapy Introduction of optogenetic for PD |
No controlled studies are available assessing the efficacy of bilateral pallidotomy. The increased incidence of side effects when performing bilateral lesions, such as worsening in speech, swallowing, cognition, or falling, will make such a study unlikely to be attempted (24–27).
THALAMOTOMY
It is generally agreed among most experts that the current optimal target for tremor control is the VIM nucleus, which receives its input primarily from the cerebellum. Although lesioning in the ventralis oralis anterior and posterior (Voa/Vop) (28) has also revealed potential benefits in rigidity, akinesia, and dyskinesia, further clinical trials with precise imaging and follow-up of the other thalamic nuclei would be required to confirm their superiority. A recent prospective trial randomized 45 PD patients to receive DBS or alternatively to receive thalamotomy. At 6 months, although the stimulation group improved more (as we will discuss in the next section), the thalamotomy group also showed improvement in tremor suppression and functional status (29), and this improvement persisted at 5 years (30). Though bilateral thalamotomy has been shown to be effective for tremor, there has generally been significant reluctance to perform bilateral thalamotomy because of the high incidence of adverse effects (speech and swallow problems, and cognitive disorders) (31).
SUBTHALAMOTOMY
The STN was purposely avoided by neurosurgeons, given the awareness that lesions in that region can result in severe hemiballism (32). Nevertheless, different surgical groups have endeavored to prove its effectiveness and secureness. Twenty-one PD patients underwent unilateral subthalamotomy and followed up for minimum 12 months (33), reporting improvement in contralateral parkinsonian symptoms, as well as in contralateral LID. In an open-label study of 89 PD patients (34), unilateral subthalamotomy proved to be effective by reducing significantly “off” motor scores at 12 (50%), 24 (30%), and 36 months (18%) postoperatively. There was also a significant improvement in the cardinal features contralateral to the lesion and a mild effect ipsilaterally. Additionally, the “on” motor state was significantly reduced at 12 and 24 months postoperatively. However, LID increased progressively over the 36 months of follow-up. The effects of bilateral subthalamotomy were evaluated in a study of 18 PD patients (35), leading to a significant reduction in the “off” (49.5%) and “on” (35.5%) UPDRS motor scores. LID reduced by 50%, and the daily levodopa dose was reduced as well by 47%.
To date, there have only had been two prospective randomized trials. In the first, 16 patients were prospectively assigned to bilateral STN DBS, bilateral subthalamotomy, or unilateral subthalamotomy plus contralateral DBS. All of the patients were followed up for 1 year. Overall, the motor performance significantly improved following all three procedures, without major differences noted in outcome. One patient in the bilateral lesion group presented with persistent hemiballismus (36). A comparison of unilateral pallidotomy and subthalamotomy was recently performed. Ten patients were randomized to either group, and this cohort revealed significant improvement in all major parkinsonian motor signs (UPDRS, Hoehn–Yahr, Schwab–England scale) at 6 months (37). One pallidal patient developed a left homonymous hemianopsia, and one patient in the subthalamotomy group developed hemiballistic movements. Overall, these studies suggest STN could be considered a viable option. We will require larger prospective trials to consider STN as a primary lesional target.
In conclusion, unilateral lesions of the pallidum or STN could provide similar beneficial effects to DBS; however, pallidotomy is usually chosen, since thalamic lesioning has been limited to tremor benefit in many case series (38). More information is needed to clarify the evidence supporting subthalamotomy (36). Bilateral lesioning is usually avoided because of the high chances of severe and irreversible side effects.
STEREOTACTIC RADIOFREQUENCY VERSUS DEEP BRAIN STIMULATION
Only a few controlled head-to-head studies have compared the uses of RF with DBS. In a prospective series of 13 patients randomized to either pallidotomy or stimulator implantation, a comparable effect on UPDRS and activities of daily living was observed after both procedures, suggesting that the short-term effects and safety were comparable (19). In another study, 34 patients were randomly assigned to unilateral pallidotomy or to bilateral STN stimulation, and in this study, stimulation was more efficacious than unilateral pallidotomy in reducing parkinsonian symptoms (22) for up to 1 (23) and 4 years after surgery (39). The most recent randomized study analyzed and compared the effects of both therapies in a PD cohort of 45 patients. Patients were randomized to either thalamotomy or thalamic stimulation. At 6 months, both therapies were equally effective for tremor suppression; however, thalamic stimulation had fewer adverse events, and a greater improvement in functional status (29). In the subsequent follow-up study at 5 years, the stimulation group maintained the improvements; however, the lesion group had less hardware-related issues, and no need for programming adjustments (30).
ROLE OF GK THALAMOTOMY
Leksell introduced stereotactic radiosurgery in 1951 when treating a trigeminal neuralgia patient (40). This technique has advanced and proved its potential for the treatment of other neurosurgical pathologies, such as arteriovenous malformations or brain tumors. Fueled by the positive results obtained with RF lesioning, the application of radiosurgery for movement disorders began in the early 1990s (41). PD patients with high surgical risks, such as those on anticoagulants, or those with severe comorbid conditions were considered (42). As an alternative to RF lesioning or to DBS, stereotactic radiosurgical pallidotomy or thalamotomy using GK or a linear accelerator, and MRgFUS thalamotomy can be performed. The long-term effects of stereotactic radiosurgical treatment for the treatment of disabling PD tremor were reported by a few studies as being similar to RF or to DBS (43). However, studies have also reported much variability in results. In a recent study of 59 PD patients, 81% improvement in UPDRS scores (parts II and III) was observed (44). In contrast, another study reported that gamma knife thalamotomy (GKT) provided only modest antitremor efficacy and that there was a delayed development of radiation-induced neurologic adverse events (45). Delayed appearance of neurologic complications secondary to radiation necrosis (sensory, pseudobulbar, visual, and speech problems) should be carefully considered as a potential outcome before subjecting patients to this procedure (46). Overall, the results of radiosurgery pallidotomy are not homogeneous in the literature, and the majority of surgical centers have abandoned this form of pallidotomy due to an unacceptable complication rate (47). Further studies will be necessary to establish the role of radiosurgery in STN. There is little evidence that GK radiosurgery can be safely applied to PD patients; however, it may be potentially suitable use for patients not appropriate for invasive surgical procedures (48).
ROLE OF ULTRASOUND THALAMOTOMY
Recently, studies using MRgFUS thalamotomy for ET have reported the procedure as potentially safe and effective when utilized as a unilateral treatment for medication-resistant tremor. In a recent case series of four patients, the technique revealed immediate and sustained improvements in tremor with a mean reduction in tremor score in the treated hand of 89.4% at 1 month and 81.3% at 3 months (49). In another open-label uncontrolled study targeting the VIM, 15 patients with severe, medication-refractory ET had significant improvement in scores for hand tremor and total tremor scores. The authors additionally reported improvement in disability and quality-of-life scores (50). Finally, in a phase 1 clinical trial investigating the feasibility and safety of the procedure, 15 ET patients underwent MRgFUS unilateral thalamotomy, and there was a reported 75% improvement in limb tremor subscores and a significant improvement in functional activities as well as quality-of-life measures (51). Ongoing clinical studies on patients with PD will have an opportunity to attempt to demonstrate that transcranial MRgFUS thalamotomy could be a novel, noninvasive, alternative treatment option for patients with unilateral therapy-resistant tremor (52). One advantage to ultrasound over conventional lesion therapy has been the ability to use “test lesions.” This potential advantage has not, however, been shown to lessen adverse events. There is a need for larger trials to validate the safety and to determine the efficacy and durability of this new approach to unilateral medication-refractory tremor.
In summary, unilateral parkinsonian symptom suppression was effective when using ablative or DBS therapy; however, stimulation approaches may be favored for the following reasons: (1) side effects’ reversibility, (2) bilateral lead implantation without the risks that have been associated with bilateral lesional therapy (speech, cognitive, and pseudobulbar), and (3) the potential for optimizing therapy during DBS programming sessions. There are patients for whom ablative procedures may be a reasonable option, and this technique may be particularly useful in high surgical risk cohorts, and in those who will have difficulty travelling for follow-up (53).
DEEP BRAIN STIMULATION
HISTORY: FROM THE ANCIENT TO MODERN ERA
Electrical stimulation as a therapeutic intervention possibly dates back to the Ancient Egyptians, where electric eels, capable of generating powerful shocks of up to 600 volts, were used to treat neurologic and to also address pain disorders (54). Similarly, in the first century, Scribonius Largus (55), the court physician to the Roman emperor Claudius, reported that pain could be relieved by standing on an electric torpedo fish. Also, Benjamin Franklin, in the 18th century, conducted experiments using therapeutic electricity on people suffering from palsies (56). Many experts attribute much of the underlying basis supporting neurostimulation to Michael Faraday, a British scientist who discovered that an electrical current can produce a magnetic field (57). This principle was later used to develop the first implantable neurostimulator for pain relief (58); however, it was not until the placement of a “chronic” DBS electrode by the French neurosurgeon Alim Benabid in 1987 that the door was opened into the modern era of human treatment especially for movement disorders (14).
MECHANISMS PROPOSED
DBS is now considered an important therapy for PD, ET, and dystonia, and it has been applied in a research setting for the treatment of other medication-refractory neurologic and neuropsychiatric diseases (59), but exactly how DBS provides symptomatic benefit to patients remains unknown. Several theories have been proposed, implying that more than one mechanism is likely responsible for the therapeutic benefit.
Inhibition Theory
One of the initial DBS mechanism theories was that electrical stimulation inhibits a target neuron’s (and regional axons) activity, thereby decreasing its neural output (60,61). Several authors have reported a reduction of activity surrounding the stimulated electrode, and the possibility of depolarization blockade through K+-mediated effects (62), inactivation of Na+ channels (63), presynaptic depression of excitatory afferents (64), and hyperpolarization of neuronal bodies and dendrites (65). Additionally, it has been reported that glutamate reduction with coincident increases of inhibitory neurotransmitters such as GABA (66) and adenosine (67) may also play a role in the mechanisms underpinning DBS. Most experts now believe that application of electricity to the brain has a strong inhibitory component especially on neuronal cell bodies close to the origin of the current, but that there are also important excitatory mechanisms.
Activation Theory
A number of studies have revealed an increase in overall firing associated with stimulation, suggesting an excitatory output from the stimulated target. This idea has been supported by an observed increase in excitatory neurotransmitters (68) and in dopamine. The dopaminergic mechanisms may be underpinned by the decrease in turnover of the remaining SNc neurons (69). Supporting the notion that DBS has excitatory properties, previous studies have shown that DBS induced an increase in blood flow in the GPi during STN stimulation and also cortical blood flow during thalamic stimulation. These studies are consistent with the activation of output hypotheses, and are further supported by PET and fMRI studies (70,71), which have revealed correlations PD motor symptom improvement (72). Recently, the potential role of astrocytes in DBS mechanisms has been at least partially elucidated. Astrocytes have been postulated to contribute in three different ways, (1) by changing cerebral blood flow, (2) by releasing excitatory neurotransmitters, and (3) by increasing reactive astrocytes and stimulating neurogenesis (73).
The Simultaneous Excitatory and Inhibitory Theory of DBS Mechanism
A fascinating finding by McIntyre (74) was that there was a possibility of inhibition in the cell body, and excitation in the axon. This DBS-induced decoupling may lead to a change in the network activity and some of the overall positive benefits seen resulting from the therapy (75).
The “Information Lesion” Theory
It has also been proposed that electrical stimulation, by disrupting pathologic oscillatory patterns, may generate an “information lesion,” preventing or disrupting the pathologic basal ganglia activity from being transmitted (76). This effect could be achieved by replacing irregular bursting cells, with regular high-frequency firing, by promotion of “prokinetic” frequencies, and abolition of pathologic β-band frequencies (77). This effect may partially or fully be caused by “jamming” the signal (78,79).
Summary of the Mechanisms of DBS
Though it remains unknown what the mechanisms underlying the therapeutic effects of DBS are, we have learned many things over the last two decades. It is likely that DBS results in inhibition of the cell bodies close to the electrical field, and excitation of the axons. It is also likely that DBS induces neurochemical changes that may be induced by stimulation of astrocytes and the induction of a propagating calcium wave that results in release of chemicals such as adenosine and glutamate (73). There are also important neurovascular and neurogenic changes that may be induced by electrical stimulation of the brain (70,71). It is now clear that stimulation of relatively small nodes can result in a large network-wide changes that may profoundly improve the symptoms of PD and other movement disorders (80). Additionally, important changes in oscillatory behavior (i.e., β and θ bands) have emerged as clues to the underlying pathophysiology (81,82). Fig. 47.1 shows the theoretical local effects of brain stimulation in a neural network.
Figure 47.1. Local effects of brain stimulation in neural network. The local effects of DBS tend to result in the inhibition of neuronal cell bodies (−) and the excitation (+) of neighboring axons. In addition, astrocytes are stimulated to release calcium, which may lead to a release of glutamate (GLUT) and adenosine (ADO), as well as local increases in cerebral blood flow. Finally, there is evidence that DBS can induce local and possibly distal proliferation of neural precursor cells. (Adapted from Okun MS. Deep-brain stimulation for Parkinson’s disease. N Engl J Med 2012;367:1529–1538.)
DBS HARDWARE
The basic DBS system is comprised of the following elements: a multicontact electrode (lead), an extension wire with connector, and an implantable pulse generator or neurostimulator that delivers the current (shown in Table 47.2). Presently, two different leads are available in the United States to deliver electrical stimulation (Medtronic, Minneapolis, MN). Both are 40-cm long, contain four platinum/iridium electrodes at the tip, measure 1.27 mm in diameter, and have individual electrodes that are 1.5 mm in length. The difference between the two DBS leads is the spacing between the electrodes, with Model 3387 with 1.5-mm spacing and Model 3389 with 0.5-mm spacing. The DBS extension, a small-diameter wire cable, is used to connect the neurostimulator to the lead and runs subcutaneously along the head, neck, and shoulder regions. Extensions are available in different lengths to accommodate different body areas. The neurostimulator is a titanium unit containing the electronics and power supply of the DBS system. Two types of neurostimulators have been previously available: Kinetra and Soletra. The first one works through two channels to deliver bilateral therapy with a single device, while the latter works through a single channel, so that ultimately two devices are required for bilateral stimulation. There are now three newer neurostimulators available on the market in the United States (Activa PC, Activa RC, and Activa SC), which provide advanced programming options allowing the clinician to reach optimized settings potentially sooner (by including the patient in the programming process) and facilitate tailoring the stimulation field to each patient. The RC is rechargeable, and the SC (single channel) and PC (dual channel) allow for the clinician to offer the patient multiple programs that can be changed by a simple handheld remote. Several other devices (St. Jude, Boston Scientific, Neuropace, Sapiens) are in various stages of development and testing. DBS programming will be reviewed in more detail later in the chapter (83,84).
| Elements Comprising DBS System (Medtronic, Minneapolis, MN) |
Neurostimulators |
|
Activa PC Model 37601 | Battery type: Primary cell Weight: 67 g (2.4 oz) Height: 65 mm (2.6 in) Length: 49 mm (1.9 in) Channels: 2 |
Activa RC Model 37612 | Battery type: Rechargeable Weight: 40 g (1.6 oz) Height: 54 mm (2.2 in) Length: 54 mm (2.2 in) Channels: 2 |
Activa SC Models 37602 and 37603 | Battery type: Primary cell Weight: Model 37602: 45 g (1.6 oz), Model 37603 44 g (1.6 oz) Height: 55 mm (2.2 in) Length: 60 mm (2.4 in) Channels: 1 |
Leads |
|
Model 3387 | Lead length: 40 cm Electrode length: 1.5 mm Electrode spacing: 1.5 mm |
Model 3389 | Lead length: 40 cm Electrode length: 1.5 mm Electrode spacing: 0.5 mm |
Extension |
|
Model 37086 | Lengths: 40, 60, 95 cm Distal connector: Quadripolar, in-line Proximal connector: Octopolar, in-line |
Source: Information acquired from Medtronic’s website (http://professional.medtronic.com/pt/neuro/dbs-md/index.htm#.UuKDAWQo7L8). |
PREOPERATIVE PROCEDURES: SELECTING PATIENTS FOR DBS
Appropriate patient selection is the most critical factor for successful DBS. Although several screening tools for choosing the “right” surgical candidate have been developed to assist clinicians in identifying appropriate patients for DBS therapy, such as the CAPSIT-PD (85), the RAND/UCLA method (86), the FLASQ-PD (87), and recently the Stimulus (88), there is currently no “gold standard” instrument. No screening tool has been universally adopted in DBS therapy. It is important to use a multi- or interdisciplinary evaluation to uncover the potential risks and benefits and also alternative approaches for each patient (89).
Which Factors Must Be Considered When Selecting a Patient for DBS?
Parkinson’s Disease: Diagnosis Confirmation, “Red Flags,” and Disease Duration. Patients need to meet criteria for “probable” idiopathic PD according to the UK PD Brain Bank (90). The evaluating physician should be aware of the potential clinical signs and symptoms that may represent contraindications to surgery, or the so-called red flags in atypical parkinsonisms presented in Table 47.3. A preoperative imaging study, preferably MRI, is mandatory in order to evaluate for the possibility of secondary causes of parkinsonism. Disease duration should also be taken in consideration when selecting patients for surgery. Operating on patients earlier than 5 years following a PD diagnosis could lead to the inclusion of patients with atypical parkinsonism (91). For that reason, a general criterion employed by many centers has been to choose patients with at least 5 years of symptoms. In addition, 5 years has been considered as a reasonable time to attempt to treat a patient with medications before considering a surgical intervention.
Levodopa Responsiveness. Levodopa responsiveness has been accepted as the single best predictive factor for DBS outcome (92) and is generally defined as an improvement of ≥ 30% in UPDRS motor score (part III) following administration of a suprathreshold dose of levodopa (usual effective dose + 50 mg or usual effective dose + 100 mg if taking dopamine agonists, or 1.5 times the equivalent dose of morning medication) compared to a 12-hour period “off dopaminergic medication” (93). It is important to counsel that symptoms unresponsive to levodopa (gait, postural instability, speech, and posture) are unlikely to improve with DBS, and could even worsen (94). Before referring a patient for surgery, it should be carefully considered whether there has been an adequate medication trial. Although an “optimal treatment” trial is not clearly defined, the selection of patients for DBS must include evaluation from a neurologist experienced in Parkinson’s treatment or a movement disorders specialist, since medication adjustments could possibly improve a patient’s symptoms.
< div class='tao-gold-member'>
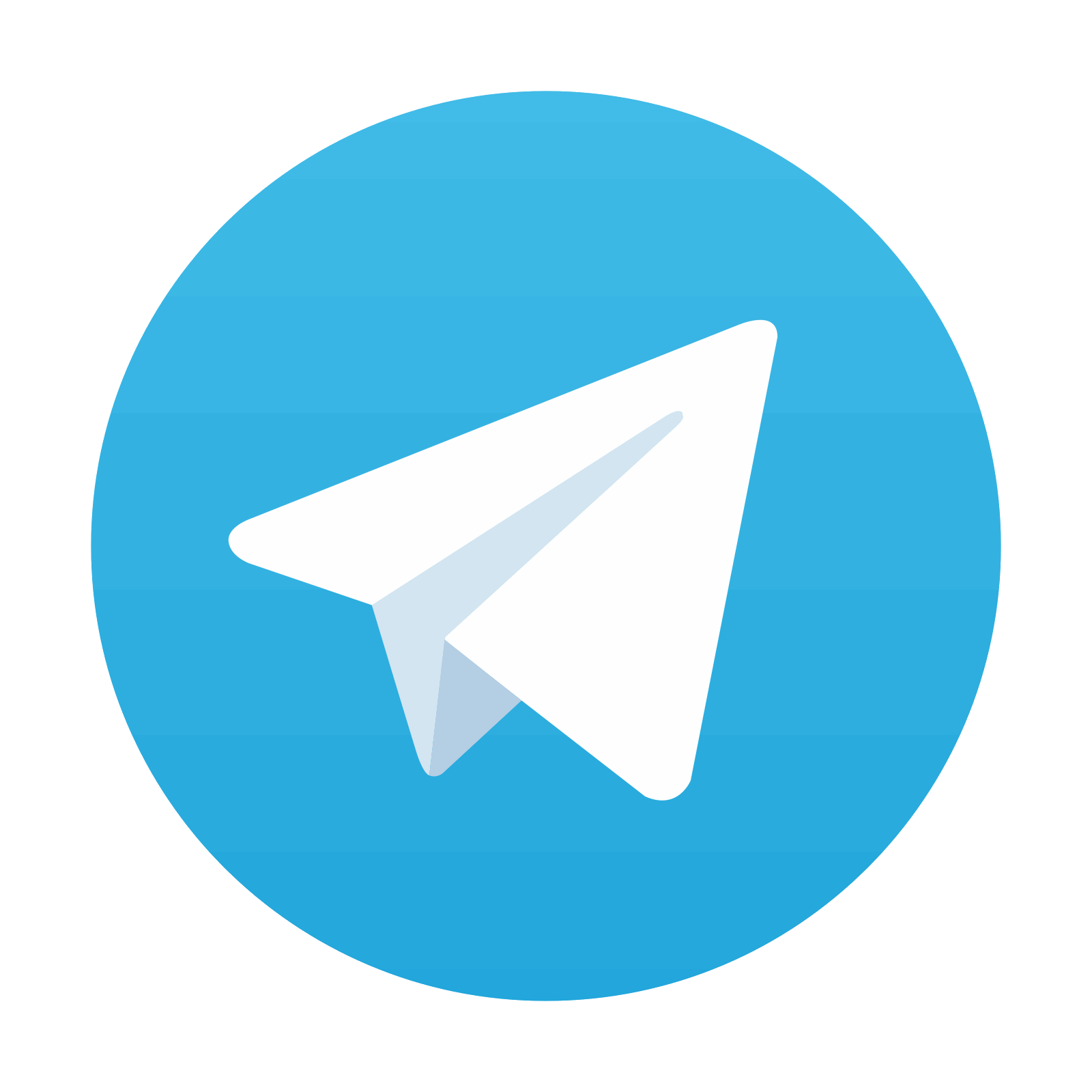