Chapter 7 Surgical treatment of Parkinson disease and other movement disorders
Introduction
A variety of surgical treatments for Parkinson disease (PD), including ablation or deafferentation of motor and premotor cortex, cervical cordotomy, and mesencephalic pedunculotomy, were performed in the first five decades of the twentieth century (Meyers, 1968). These procedures generally yielded relief of the movement disorder at the expense of concomitant weakness and other complications. Surgery at the level of the basal ganglia for PD was pioneered by Meyers in 1939 (Meyers, 1968). These open procedures included removal of the head of the caudate and section of the anterior limb of the internal capsule and pallidofugal pathways. After Spiegel and Wycis introduced the principles of stereotactic surgery in clinical practice in 1947, this method was applied for lesioning the pallidum and ansa lenticularis in an attempt to treat the symptoms of PD and other movement disorders (Mundinger and Reichert, 1963; Hassler et al., 1979; Grossman and Hamilton, 1991). Stereotactic thalamotomy for parkinsonian symptoms was introduced by Hassler and Riechert in 1951 (Hassler et al., 1979). Thalamotomies gradually replaced pallidotomies in the late 1950s and early 1960s (Table 7.1) because thalamotomies were thought to produce more sustained control of tremor. The introduction of levodopa in the late 1960s resulted in a marked reduction in the number of functional stereotactic procedures, and only a few specialized centers continued to perform such operations.
Table 7.1 Milestones in the surgical treatment of Parkinson disease
The renewed interest in surgical treatment of movement disorders has been stimulated in part by improved understanding of the functional anatomy underlying motor control, as well as refinement of methods and techniques in neurosurgery, neurophysiology, and neuroimaging (Krauss et al., 1998; Gross et al., 1999a; Lang, 2000b; Mazziotta, 2000; Jankovic, 2001; Krauss, et al., 2001a; Walter and Vitek, 2004). Furthermore, important strides have been made in assessments of the outcomes of surgery and in providing useful guidelines for inclusion–exclusion criteria (Defer et al., 1999; Tan and Jankovic, 2000, 2009). As a result of increased awareness about surgical options for patients with PD, the attitudes of clinicians toward referring patients for surgery have been changing, and in one survey, 99.4% of neurologists were aware of surgery for PD (Mathew et al., 1999). Furthermore, there is growing appreciation of the importance of holding surgical trials to as stringent evidentiary standards as other clinical studies, and the notion of double-blind design including “sham” operations is increasingly accepted (Prehn et al., 2006). Although this review focuses primarily on surgical treatment of PD, there is growing interest in the application of surgical intervention in the treatment of a variety of movement disorders (Pollak, 1999; Krauss et al., 2001a). While the interest in surgical treatment of movement disorders is growing, there is a remarkable paucity of well-designed, randomized trials (Stowe et al., 2003).
Functional anatomy of the basal ganglia
Before discussing the indications for and the results of surgery for PD, it is helpful to review the current concepts about the functional anatomy of the basal ganglia (Figs 7.1 and 7.2). The basal ganglia (extrapyramidal system) include the striatum, globus pallidus, substantia nigra, subthalamic nucleus (STN) (Hameleers et al., 2006; Benarroch, 2008), and thalamus (Parent and Cicchetti, 1998; Hamani et al., 2004). The caudate and putamen are contiguous and comprise the striatum, and the putamen and globus pallidus are referred to as the lenticular nucleus. The cortical input from the prefrontal supplementary motor area, amygdala, and hippocampus is excitatory, mediated by glutamate. Neurons in the substantia nigra pars compacta provide major dopaminergic input to the striatum. The interaction between the afferent and efferent pathways is mediated by striatal interneurons that utilize acetylcholine as the main neurotransmitter. The substantia nigra is a melanin-containing (pigmented) nucleus in the ventral midbrain, and it consists of dopaminergic neurons. The striatal output system is mediated by the inhibitory neurotransmitter gamma-aminobutyric acid (GABA). However, the basal ganglia appear to be more complex than is indicated by the current models (Parent and Cichetti, 1998; DeLong and Wichmann, 2007). For example, it is now well recognized that the STN provides powerful excitatory projection not only to the globus pallidus interna (GPi), but also to the striatum and globus pallidus externa (GPe) and, in turn, receives input from the cerebral cortex, substantia nigra pars compacta, and various brainstem and thalamic nuclei. Although most reports emphasize the pallidal-thalamic projection, major output from the GPi is to the brainstem nuclei, such as the pedunculopontine nucleus (PPN) (Alam et al., 2011; Jenkinson et al., 2006; Kenney et al., 2007a; Kuo et al., 2008; Zrinzo et al., 2008; Jenkinson et al., 2009; Ferraye et al., 2010; Moro et al., 2010). Some studies have also drawn attention to the role of the PPN in gait and locomotion as PD patients have a markedly reduced number of cholinergic PPN neurons (Rinne et al., 2008).
The reemergence of surgical treatment of PD, particularly pallidotomy and STN/GPi deep brain stimulation (DBS) (see later), has been fueled in part by improved understanding of basal ganglia circuitry, including the recognition that the STN and the GPi are overactive in experimental and human parkinsonism (Bergman et al., 1990; Limousin et al., 1995). Microelectrode-guided single-cell recordings in patients with PD showed that the average firing rate in the GPi was 91 ± 52 Hz and that in the GPe it was 60 ± 21 Hz (Magnin et al., 2000). In addition, rhythmic, low-threshold calcium spike bursts are often recorded in the pallidum and medial thalamus; some but not all are synchronous (in phase) with the typical rest tremor. It has been postulated that the low-threshold calcium spike bursts contribute to rigidity and dystonia by activating the supplementary motor area. Apomorphine, a dopamine agonist, has been found to suppress the abnormal hyperactivity of the GPi and STN and to enhance the activity of the GPe on the basis of cellular recordings during surgery (Lozano et al., 2000). However, marked or complete suppression of GPi activity is associated with an emergence of dyskinesias. Indeed, levodopa- or dopamine-induced dyskinesias are associated with decreased firing frequency of the GPi neurons and a modification in the firing pattern (Boraud et al., 2001). This suggests that dopaminergic drugs and pallidotomy improve parkinsonian symptoms through a similar mechanism. Single-cell recording of the STN in patients with PD showed characteristic somatotopic organization, with neurons responding to sensorimotor stimuli localized chiefly in the dorsolateral region, and were of the irregular or tonic type (Rodriguez-Oroz et al., 2001). These two groups of neurons represent 60.5% and 24% of all STN neurons, respectively; only 15.5% of the STN neurons are oscillatory. Oscillatory activity in the basal ganglia is attracting more and more attention on the basis of various surgery-related neurophysiologic studies (Dostrovsky and Bergman, 2004). Microinjection of 10–23 µL of lidocaine into the STN of three patients with PD produced “striking improvements in bradykinesia, limb tremor and rigidity” in all (Levy et al., 2001). Furthermore, microinjections of 5–10 µL of muscimol, a GABAA receptor agonist, in the region of the STN that showed oscillatory activity resulted in suppression of contralateral tremor in two patients. Simultaneous microelectrode recordings showed suppression of neuronal activity in the near vicinity (up to 1.3 mm) of the injection. In a study designed to explore the effects of GPi on the STN, Sterio and colleagues (2002) showed that GPi stimulation markedly reduced the firing rate of dorsal STN cells in the ventral STN (and substantia nigra pars reticulata). In addition to providing support for STN segregation, this suggests that there is a feedforward GPi–STN interaction that needs to be incorporated in revised models of functional anatomy of the basal ganglia. The oscillatory nature of human basal ganglia activity in relationship to movement has been recently reviewed (Brown et al., 2004).
The basal ganglia models in dystonia are even less clear; some studies have found that the GPi neuronal activity is increased in dystonia (Sanghera et al., 2003), whereas other studies have failed to find any decrease in basal ganglia output (Hutchison et al., 2003). Neurophysiologic studies performed during STN DBS have found that the STN receives direct input from the supplementary motor area and is thus involved in movement preparation, as demonstrated by recorded activity in the nucleus before voluntary movement (Paradiso et al., 2003).
Posteroventral pallidotomy (PVP) as well as GPi and STN DBS (see later) improve motor performance in patients with PD, presumably by interrupting inhibitory pallidal projections to the ventrolateral thalamus. This is supported by measurements by positron emission tomography (PET) of regional cerebral blood flow showing increased activity of supplementary motor area and premotor cortex (but not in primary motor cortex) after pallidotomy (Grafton et al., 1995; Eidelberg et al., 1996). One possible explanation for the apparent improvement of parkinsonian features after STN or GPi ablation or simulation is that the reduced excitability of the GPe in PD prevents the normal “brake” on STN firing and leads to overactivation of the STN and GPi. Despite lack of clear understanding of the mechanisms, surgical approaches are increasingly used in the treatment of patients with PD who fail to obtain satisfactory relief from pharmacologic therapy (Krauss and Jankovic, 1996; Hallett et al., 1999).
Techniques of stereotactic surgery
Stereotactic surgery is based on a Cartesian coordinate system, which implies that any point in space may be determined by three right-angled planes defined as the x, y, and z axes (Krauss and Jankovic, 1996). Functional stereotactic surgery relies on the acquisition of data from various imaging modalities and its transfer to the Cartesian coordinates referenced to an apparatus, the stereotactic frame, which is rigidly fixed to the patient’s head (Hassler et al., 1979; Grossman and Hamilton, 1991). By using computed tomography, magnetic resonance imaging (MRI), or positive-contrast ventriculography, the target coordinates for functional stereotactic surgery are determined by extrapolation referring to the coordinates of the anterior and posterior commissure. The data for the spatial relation of the target to the anterior commissure (AC) and posterior commissure (PC) are derived from stereotactic atlases. For example, the STN target, used chiefly for STN DBS, is 10–12 mm lateral and 2–3 mm posterior to the midcommissural point and 2–4 mm below the AC–PC line. Some surgeons have also advocated the use of the red nucleus as an internal marker for targeting the optimal region of STN stimulation (Andrade-Souza et al., 2005). Various issues related to techniques of stereotactic surgery, particularly related to DBS, have been summarized in several reviews (Gross et al., 2006; Machado et al., 2006; Rezai et al., 2006).
To improve the accuracy despite normal anatomic variability, physiologic verification of the target by microelectrode recordings of spontaneous neuronal activity or by electric stimulation has been considered critical by some (Tasker, 1993; Obeso et al., 1998; Starr et al., 1998; Vitek et al., 2004), but other investigators believe that stereotactic surgery can be performed safely and effectively without microelectrode recording, using MRI-directed targeting (Dewey et al., 2000; Patel et al., 2003b; Hamid et al., 2005).
Different types of stereotactic devices are available. Functional stereotactic operations are generally performed under local anesthesia to allow examination of the patient during the physiologic investigations and during application of the lesions. In some cases, generalized anesthesia may be used safely (Maltete et al., 2004). The choice of the target and the techniques for calculation of the target as well as for physiologic localization differ (Mundinger and Reichert, 1963; Hassler et al., 1979; Grossman and Hamilton, 1991; Tasker, 1993; Tasker and Kiss, 1995). Usually, the target is chosen contralateral to the side that is more severely affected. The stereotactic frame is fixed to the skull with screws. The patient then undergoes stereotactic computed tomography scanning. While the coordinates of the target are calculated, the patient is brought back to the operating room. A small area of the head in the frontal region is shaved. A precoronal parasagittal burrhole is made via a linear incision under local anesthesia. The arch of the stereotactic device is fixed to the frame and the electrode for recording, or stimulation is directed to the precalculated target via a cannula. The tip of the microelectrode that is used for recording has a diameter of 0.01 mm, whereas the tip of the electrode that is used to produce the lesion has a diameter of 1.1 mm. After physiologic localization of the target, one to three lesions are made along the trajectory, heating the tip of the electrode to 75°C for 60 seconds. The symptomatic improvement, particularly the cessation of tremor or levodopa-induced dyskinesia, reduced rigidity, and improved performance of rapid succession movements, is usually noted immediately after placing the lesion. It is advisable to operate when the patient is “off” (before taking his or her morning dose of medication), since the effect of the surgery can be assessed more readily. The duration of the procedure varies between 2–3 hours for a standard thalamotomy and 4–5 hours for a pallidotomy. The hospital stay varies between 2 and 5 days.
Thalamotomy
Prior to the advent of levodopa therapy for PD, thalamotomy offered the most effective means of controlling disabling and embarrassing tremor. Stereotactic thalamotomy has been refined substantially since its introduction in 1947 as a result of improvements in neuroimaging and electrophysiologic and surgical techniques. The application of the procedure has broadened to disorders other than tremor, particularly dystonia, hemiballism, and severe levodopa-induced dyskinesias (Cardoso et al., 1995; Jankovic, 1998; Krauss and Grossman, 1998; Starr et al., 1998; Jankovic et al., 1999c). We analyzed the outcome of 60 patients with medically intractable tremor who underwent a total of 62 stereotactic thalamotomies at Baylor College of Medicine (Jankovic et al., 1995). The ventral intermediate (VIM) nucleus of the thalamus was the target in all patients. The patients were followed for as long as 13 years (mean: 53.4 months) after their surgery. At the most recent follow-up visit, 36 of 42 (86%) patients with PD, 5 of 6 (83%) with essential tremor (ET), 4 of 6 (67%) with cerebellar outflow tremor, and 3 of 6 (50%) with post-traumatic tremor had complete cessation of or moderate to marked improvement in their contralateral tremor. Patients who were taking levodopa (n = 35 patients) were able to reduce their daily dose by approximately 156 mg. Immediate postoperative complications, such as contralateral weakness (34%), dysarthria (29%), and confusion (23%), occurred in 58% of the 60 patients; these complications usually resolved rapidly during the postoperative period. These results are consistent with other reports, confirming the beneficial effects of thalamotomy on tremor and rigidity but no effect on bradykinesia in patients with PD (Zirh et al., 1999). Thalamotomy was also considered to be modestly effective in reducing the amplitude of kinetic tremor associated with multiple sclerosis (Alusi et al., 2001; Matsumoto et al., 2001; Thevathasan et al., 2011). In one series, 11 consecutive patients with multiple sclerosis tremor, permanent tremor reduction was observed in 11 of the 18 upper limbs with tremor (Thevathasan et al., 2011). Some authors have suggested that thalamic stimulation in multiple sclerosis promotes local “demyelinative lesioning.” Furthermore, thalamotomy may improve levodopa-induced dyskinesia. Improved localization of the cluster of thalamic neurons with the largest amount of tremor discharges, correlated with electromyographic activity, should produce even better results. The likelihood of marked or complete tremor relief is high when the thalamic lesion is made within 2 mm of this site (Lenz et al., 1995). High-frequency stimulation (to be discussed later) rather than lesioning of the thalamic nuclei may be more effective and safer in the treatment of tremor (Schuurman et al., 2000). Since bilateral thalamotomy can cause hypophonia, dysarthria, and dysphagia, DBS is emerging as a useful alternative in those patients who require bilateral procedures. Thalamotomy has the advantage over DBS in that there is no need for hardware; and for patients with disabling bilateral tremor, unilateral thalamotomy in combination with contralateral DBS may offer the optimal tremor control with the fewest adverse side effects. Finally, microinjections of muscimol into the region of VIM thalamus that contains the tremor-synchronous cells consistently reduced tremor, suggesting that GABA agonists might be useful in the treatment of tremor (Pahapill et al., 1999).
Ablative lesions of the pallidum and subthalamic nucleus
Although a common procedure in the 1950s and 1960s, anterior pallidotomy was later abandoned because of inconsistent results, particularly concerning tremor, and because of improved results with posterior pallidotomy and later with DBS (Okun and Vitek, 2004). While some investigators had noted improvement of bradykinesia, this observation was not described by others (Hassler et al., 1979). Most surgeons at that time targeted the anterior dorsal portion of the GPi. More favorable results, with improvement of rigidity, bradykinesia, and tremor, were reported by the group of Leksell, who had chosen a different target, namely, the posterior and ventral aspect of the GPi. After Laitinen had reevaluated Leksell’s approach in the early 1990s (Laitinen et al., 1992), pallidotomy was quickly reintroduced in North America and Europe (Sterio et al., 1994; Dogali et al., 1995; Lozano et al., 1995). Lesioning of the most ventral segment of the GPi provides the most antidyskinetic effect (Kishore et al., 2000).
The tentative target in the posteroventral GPi is located most commonly 20–21 mm lateral to the midline, 4–5 mm below the intercommissural line, and 2–3 mm anterior to the midcommissural point. The accurate localization of the target within the pallidum is essential not only for optimal therapeutic results, but also to avoid lesioning of adjacent structures. Single-cell microelectrode recording helps in delineating the borders of the GPi (Alterman et al., 1999). Different neuronal signals are identified along the pathway through the putamen, GPe, and GPi (Grafton et al., 1995). Cells with bursting discharges and low-frequency activity interrupted by pauses are characteristic for the GPe, while irregular, high-frequency discharges at a frequency of 60–130 Hz mark the GPi. GPi neurons may change their firing rates on movements of various joints of the limbs. It is particularly important to identify the ventral border of the GPi and the adjacent optic tract, which might be located at a distance of only 2–3 mm from the derived target. Stimulation via the microelectrode, which may elicit visual phenomena, is also helpful in recognizing the optic tract. The mapping might require several trajectories before the final localization for the lesion is determined. Then the radiofrequency lesioning electrode is advanced, and “macrostimulation” is applied to identify whether and at what threshold the electric current spreads to the adjacent internal capsule. If no unwanted responses are encountered, the final lesion is then made.
Because of the importance of proper localization, several reports have raised concerns about the role of gamma knife (GK) in the treatment of various movement disorders (D.P. Friedman et al., 1999
; Okun et al., 2001). Okun and colleagues (2001) described eight patients who, over a period of 6 months, were treated with GK surgery for PD and developed serious complications; one died as a result of aspiration pneumonia secondary to dysphagia. Other complications included hemiplegia, hemianopsia, limb weakness, speech and voice impairment, sensory deficit, and uncontrollable laughter. The authors concluded that these complications were related in all cases to missing the intended target and a resultant involvement of adjacent structures. Some problems may also relate to delayed effects of radiation necrosis, which might not have been fully appreciated in earlier reports on GK. Since their report represents only a small subset of patients treated with GK in their institution, the overall frequency of GK-related complications is not known. Nevertheless, this study sounds a loud alarm by drawing attention to the possibility that this procedure, often promoted as safer than the surgical treatment requiring penetration of the skull and brain parenchyma with a lesioning or stimulating electrode, can be associated with serious complications. This study must be interpreted cautiously, however, as this is not a controlled study in which patients are randomized to receive GK, ablative procedure, or DBS. Nevertheless, the report by Okun and colleagues (2001) is important because it highlights two major limitations of GK in the treatment of movement disorders: (1) it does not allow microelectrode recordings to verify the location of the target, and (2) it is associated with an unacceptably high rate of immediate and delayed complications. Although GK thalamotomy has been reported to improve essential tremor (Niranjan et al., 2000), a study of 18 patients concluded that this procedure provides “only modest antitremor efficacy” (Lim et al., 2010). Nevertheless some reports have suggested that GK thalamotomy is effective in ameliorating action tremor associated with multiple sclerosis (Niranjan et al., 2000) and with other movement disorders (J.H. Friedman et al., 1999).Lesioning of the posteroventral portion of the GPi (Laitinen et al., 1992; Dogali et al., 1995; Iacono et al., 1995; Lozano et al., 1995; Baron et al., 1996; Lai et al., 1996; Olanow, 1996; Kishore et al., 1997; Lang et al., 1997; Uitti et al., 1997; Kumar et al., 1998b; Masterman et al., 1998; Starr et al., 1998; Bronstein et al., 1999; Dalvi et al., 1999; Gross et al., 1999b; Hallett et al., 1999; Lang et al., 1999; Samii et al., 1999; Schrag et al., 1999; Lai et al., 2000; Counihan et al., 2001) and the STN (Bergman et al., 1990; Guridi et al., 1993; Limousin et al., 1995; Obeso et al., 1998; Barlas et al., 2001; Guridi and Obeso, 2001; Strutt et al., 2009) has an advantage over thalamotomy because this procedure improves not only tremor but also bradykinesia and rigidity. Although some investigators (Subramanian et al., 1995) have suggested that PVP is as effective as thalamotomy in controlling parkinsonian tremor, others (Dogali et al., 1995) feel that pallidotomy provides only partial relief of tremor. The latter authors suggest, however, that thalamotomy has a higher complication rate, particularly with respect to dysarthria and impairment of balance. In our series, only 6 of 60 (10%) patients had persistent dysarthria, and none had persistent loss of balance (Jankovic et al., 1995). However, because the two procedures have never been compared in a controlled fashion, it is difficult to comment on possible differences in efficacy and complication rates.
When a lesion is precisely localized to the GPi by neuroimaging (Krauss et al., 1997; Desaloms et al., 1998; Kondziolka et al., 1999) or by microelectrode recording techniques (Lang et al., 1997), the benefits can be quite dramatic. Subsequent follow-up of 39 patients who were followed for 6 months, 27 who were followed for 1 year, and 11 who were followed for 2 years provided additional evidence of long-term efficacy of this procedure (Lang et al., 1997). There was a 28% reduction in the “off” motor score in 6 months and an 82% improvement in contralateral “on” dyskinesias. The motor improvement was generally sustained during the 2-year follow-up, although the improvement in ipsilateral and axial symptoms gradually waned. In another study of 15 PD patients who were followed postoperatively for 1 year, the total Unified Parkinson’s Disease Rating Scale (UPDRS) score improved by 30% at 3 months, and the score remained improved at 1 year (P < 0.001) (Baron et al., 1996). In addition, there was a marked improvement in contralateral rigidity, tremor, and bradykinesia as well as improvement in gait, balance, and freezing. Although contralateral dyskinesia and tremor remain improved, all other symptoms of PD usually worsen 3 years after the surgery (Pal et al., 2000).
The most robust beneficial effect of pallidotomy is improvement in levodopa-induced dyskinesia (Jankovic et al., 1999b, 1999c; Fine et al., 2000; Lai et al., 2000; Lang, 2000a; Counihan et al., 2001). At Baylor College of Medicine, we followed 101 consecutive patients who underwent PVP procedures performed at our center and returned for at least one postoperative evaluation after 3 months (Lai et al., 2000). All had standardized clinical evaluations within 1 week before surgery and every 3–6 months after surgery. Data were collected during “on” and practically defined “off” periods for the UPDRS, Hoehn and Yahr stage, Schwab and England Activities of Daily Living (ADL) scale, and movement and reaction time. In addition, the severity and anatomic distribution of dyskinesia, neuropsychologic status, average percent of “on” time with and without dyskinesia, and clinical global impression were assessed during a longitudinal follow-up. Eighty-nine patients (46 men and 43 women) underwent unilateral PVP, and 12 patients (6 men and 6 women) had staged bilateral PVP. At 3 months after unilateral or staged bilateral PVP, 84 of the 101 patients reported marked or moderate improvement in their parkinsonian symptoms. Postoperative UPDRS mean total motor score improved in the “off” state by 35.5%, and the mean ADL score improved by 33.7% (P < 0.001). Rigidity, bradykinesia, and tremor scores also markedly improved after PVP, particularly on the contralateral side. Levodopa-induced dyskinesia was markedly reduced, while daily “on” time increased by 34.5% (P < 0.001). Seven patients had transient perioperative complications, including confusion, expressive aphasia, pneumonia, and visual changes. Improvements in parkinsonian symptoms were maintained in both “off” and “on” states in 67 patients at 12 months after PVP and in 46 patients who were followed for a mean period of 26.3 months. Patients who underwent staged bilateral PVP benefited further from the second procedure. Five of 12 patients experienced some adverse event. On the basis of this large series of patients with extended follow-up, we conclude that PVP is an effective and relatively safe treatment for medically resistant PD, especially for dopa-induced dyskinesia, tremor, rigidity, and bradykinesia. Motor fluctuations also improved. Benefits are most noticeable on the side contralateral to the PVP. Sustained (>1 year) improvement in motor function after bilateral pallidotomy has been also demonstrated by others (Counihan et al., 2001). Clinical improvement has been sustained for longer than 2 years. In a “blinded” review of videotapes, Ondo and colleagues (1998b) showed a significant improvement in “off” UPDRS scores in patients undergoing pallidotomy. Unilateral pallidotomy was found to be an effective treatment in a randomized, single-blind, multicenter trial (de Bie et al., 1999). In comparison to a control group that did not receive surgery, the pallidotomy patients improved their UPDRS III “off” motor score from 47 to 32.5, whereas the score in the control group increased from 52.5 to 56.5 (P < 0.0001). Furthermore, “on” UPDRS scores improved by 50%, chiefly as a result of marked improvement in dyskinesias. Most important, there was a significant improvement in the quality of life in patients who were treated surgically in comparison to those who were treated medically. In a follow-up study, the investigators showed that the benefits persist for at least 1 year and that patients with 1000 levodopa equivalent units or lower were most likely to improve (de Bie et al., 2001). An improvement in the quality of life, using various measures, has been demonstrated by other pallidotomy series (Martinez-Martin et al., 2000). This improvement may persist for up to 5.5 years (Baron et al., 2000; Fine et al., 2000). Evidence-based analysis of the effects of medical and surgical interventions on health-related quality of life (HRQoL) measures concluded that only unilateral pallidotomy, STN DBS, and rasagiline have been shown to be efficacious in improving HRQoL, but there is “insufficient evidence” that many well-established treatments, including levodopa and dopamine agonists, improve HRQoL (Martinez-Martin and Deuschl, 2007). The longest follow-up, over 10 years, after pallidotomy showed that while the patients clearly benefited from the procedure, the levodopa dosage had to be increased as a result of the disease progression, and most patients gradually became troubled by various mental and medical complications associated with the disease and aging (Hariz and Bergenheim, 2001). In a randomized trial of pallidotomy versus medical therapy, Vitek and colleagues (2003) found pallidotomy more effective as suggested by a 32% reduction in total UPDRS compared to 5% at 6 months.
Pallidotomy improves not only levodopa-induced dyskinesias, but also PD-related bradykinesia. This is best demonstrated by the finding of improved movement time and reaction time during the practically defined “off” state following pallidotomy (Jankovic et al., 1999a). Unilateral pallidotomy was also associated with improved simple and choice reaction times during the optimal “on” period (Hayashi et al., 2003). Kimber and colleagues (1999) suggested that the improvement in bradykinesia after pallidotomy may be explained by “greater efficacy of external cues in facilitating movement after withdrawal of the abnormal pallidal discharge.” Pfann and colleagues (1998) also showed that “off” bradykinesia improves after pallidotomy but could not demonstrate any improvement in “on” bradykinesia. We also found a remarkable improvement in freezing contralateral to the lesion in several of our patients as well as objective evidence of benefits in gait and balance (Robert-Warrior et al., 2000; Jankovic et al., 2001). Improvements in gait (Baron et al., 1996; Siegel and Verhagen Metman, 2000) and postural stability (Melnick et al., 1999) were also reported in other pallidotomy series (Bakker et al., 2004).
Pallidotomy requires a multidisciplinary approach involving skilled neurologists, neurosurgeons, neuroradiologists, physiologists, physiatrists, and nurses to obtain optimal results (Bronstein et al., 1999). Even when performed by a team of experienced clinicians, pallidotomy can be associated with potentially serious complications. The reported complications include transient confusion, expressive aphasia, hemiparesis, facial paresis, pneumonia, and visual changes, such as homonymous hemianopia (Laitinen et al., 1992; Shannon et al., 1998).
Cognitive function and various neuropsychologic measures have been studied extensively in patients following surgery for PD, and these domains have been found to be generally preserved, particularly after unilateral pallidotomy (Masterman et al., 1998; Perrine et al., 1998; Rettig et al., 1998; York et al., 1999; Lombardi et al., 2000; Rettig et al., 2000; Saint-Cyr and Trépanier, 2000; Green et al., 2002; Contarino et al., 2007), although subtle changes in verbal fluency and possibly executive functions have been noted after left pallidotomy (Schmand et al., 2000) and after bilateral pallidotomy (Scott et al., 1998). Staged bilateral pallidotomy, although beneficial in most patients, results in increased risk of complications, particularly worsening of speech and other bulbar functions (Intemann et al., 2001). Bilateral simultaneous pallidotomy may be associated with even more frequent and severe complications, such as depression, obsessive-compulsive disorder, abulia, pseudobulbar palsy, apraxia of eyelid opening, and visual field deficits (Ghika et al., 1999). In a systematic review of morbidity and mortality associated with unilateral pallidotomy, de Bie and colleagues (2002) found that the risk of permanent adverse effects was 13.8%, and symptomatic infarction or hemorrhage occurred in 3.9%; mortality was 1.2%. Several investigators have used implanted DBS electrodes to produce lesions in the thalamus for treating tremor and in the pallidum for treating levodopa-induced dyskinesias (Raoul et al., 2003). Although pallidotomy is used primarily to improve parkinsonian symptoms and levodopa-induced dyskinesias, bilateral pallidal lesions in otherwise normal individuals result in inadequate anticipatory and compensatory postural reflexes, bradykinesia, and other signs of motor impairment (Haaxma et al., 1995). This apparent paradox is difficult to explain with the current models of basal ganglia circuitry, but it suggests that nigrostriatal dopaminergic deficiency causing activation of the GPi is a necessary prerequisite for the beneficial effects of pallidotomy. Pallidotomy has now been essentially abandoned in favor of DBS and prior pallidotomy has been shown to be a poor predictor of outcome from STN DBS (Ondo et al., 2006).
The mechanism by which pallidotomy improves levodopa-induced dyskinesia is not known, but single-cell recordings in the GPi of parkinsonian monkeys show a marked reduction in firing rates only when dyskinesias were present (Papa et al., 1999). The average firing rate decreased from 46 Hz during the “off” state to 26 Hz during the “on” state and to 7.6 Hz during dyskinesia. It has been hypothesized that either overactive GPi (in a parkinsonian state) or low GPi activity (during dyskinesias) results in an abnormal (“noisy”) input to the thalamocortical circuit. Pallidotomy tends to eliminate the “noise” and “normalize” the output.
Since pallidotomy has such a robust effect on levodopa-induced dyskinesia, including dystonia, the procedure has been applied in the treatment of primary and secondary dystonia (Jankovic, 1998; Ondo et al., 2001b; Yoshor et al., 2001). In a series of patients with generalized dystonia, about 50% improvement on various dystonia rating scales was observed following pallidotomy (Ondo et al., 1998a). Some patients, particularly those with primary generalized dystonia, however, had a marked improvement, and as a result of the surgery, their dystonia-related disability changed from a dependent state to completely independent functioning.
Since the greatest effect of GPi ablation or DBS is on levodopa-induced dyskinesias, these procedures have been also tried in the treatment of other hyperkinesias, such as generalized dystonia (Jankovic, 1998; Coubes et al., 2000; Ondo et al., 2001b; Albright, 2003; Coubes et al., 2004; Diamond and Jankovic, 2005; Vidailhet et al., 2005; Kupsch et al., 2006; Vidailhet et al., 2007; Tisch et al., 2007; Isaias et al., 2008, 2009; Sensi et al., 2009), cervical dystonia (Krauss et al., 1999; Parkin et al., 2001; Krauss et al., 2002; Hung et al., 2007; Kiss et al., 2007; Moro et al., 2009), cranial-cervical dystonia (Hebb et al., 2007; Ostrem et al., 2007, 2011), chorea and ballism (Thompson et al., 2000; Hashimoro et al., 2001; Krauss and Mundinger, 2001), and tics associated with Tourette syndrome (TS) (Cosgrove and Rauch, 2001). In a study of 9 patients with primary cervical dystonia, STN DBS resulted in an improvement of the TWSTRS total score from a mean of 53.1 (± 2.57) to 19.6 (± 5.48) (P < 0.001) at 12 months (Ostrem et al., 2011).
High-frequency stimulation of the subthalamic nucleus (STN) has become an accepted treatment option for patients with moderately advanced PD (see later), but subthalamotomy has not been studied extensively (Tarsy, 2009). Because of its key role in the pathogenesis of PD, the STN has become a primary target for surgical treatment of PD. Although hemichorea/hemiballism is a well-recognized complication of a lesion in the STN, such hyperkinesias is very rare when the STN is lesioned (or stimulated) in the setting of PD (Barlas et al., 2001; Guridi and Obeso, 2001). This suggests that as a result of reduced activity of the “direct” GABAergic pathway from the striatum to the GPi, the parkinsonian state increases the threshold for such hyperkinesias. In PD, STN lesion reduces excitation of the GPi and simultaneously further reduces the hypoactivity of the GPe, compensating for the GPi hypoactivity, self-stabilizing the basal ganglia output, and reducing the risk of hemichorea/hemiballism. Alvarez and colleagues (2005) reported their experience in 11 patients after unilateral dorsal subthalamotomy. They found a significant reduction in UPDRS score, which was maintained in four patients for 24 months. Despite the location of the lesion, the procedure was not complicated by hemiballism. They followed up on their initial experience in 89 patients treated with unilateral subthalamotomy, 68 of whom were available for evaluations after up to 36 months (Alvarez et al., 2009). In addition to significant reduction in the UPDRS scores, levodopa daily dose was reduced by 45%, 36%, and 28% at 12, 24, and 36 months after surgery. Postoperative hemichorea-ballism was noted in 14 patients (15%) and it required pallidotomy in eight. Thus subthalamotomy seems to be a useful alternative to STN DBS when the latter is not accessible for economic or other reasons. In another study, unilateral dorsal subthalamotomy, particularly when combined with lesions in the H2 field of Forel and the zona incerta, resulted in a marked improvement in contralateral tremor, rigidity, and bradykinesia (Patel et al., 2003a). In one patient, a lesion confined to the STN produced “dyskinesia” that required H2/zona incerta DBS. In a series of 12 patients who underwent unilateral subthalamotomy, Su and colleagues (2003) showed a 30–38% improvement in UPDRS II and UPDRS III and an 85% improvement in dyskinesia, with 42% reduction in levodopa dosage. The benefits persisted for about 18 months. Complications included three (25%) cases of hemiballism; two of these patients recovered spontaneously, and one died of aspiration pneumonia. In a long-term (>3 years) follow-up of 18 patients with PD, bilateral subthalamotomy was associated with a significant improvement of ADL, reduction of levodopa-related dyskinesia by 50%, and lowering of levodopa dose by 47%, but the response was quite variable (Alvarez et al., 2005). Bilateral subthalamotomy was performed through DBS electrodes in a 60-year-old man with PD as a rescue option for DBS-device-related infection (Deligny et al., 2009). One potential advantage of subthalamotomy compared to pallidotomy is that the latter may adversely affect subsequent response to levodopa, DBS, or other restorative therapies, since these depend on the normal function of the outflow nuclei. Subthalamotomy, however, also seems to reduce the metabolic activity of the ipsilateral GPi, midbrain, pons, and thalamus (Su et al., 2001).
Deep brain stimulation
Ablative procedures have been largely replaced by DBS following the 1987 discovery that high-frequency stimulation of the thalamus mimics the therapeutic effects of lesioning in controlling tremor (Benabid et al., 1987, 1991, 2009). It has long been known that high-frequency (>100 Hz) stimulation employed during thalamotomies at the site of the planned lesion temporarily suppresses tremor (Mundinger and Reichert, 1963; Meyers, 1968; Hassler et al., 1979). Because of the ability to customize the stimulation parameters and the relatively low risk of complications, DBS is now considered the preferred surgical treatment for disabling PD-related tremor, ET, levodopa-related complications, generalized dystonia, and other movement disorders (Tasker, 1998). This application of chronic thalamic stimulation was later adopted for the treatment of chronic pain. Hypothalamic DBS is currently used for the treatment of various pain disorders, including migraines and cluster headaches (Leone et al., 2003). The Food and Drug Administration (FDA) approved DBS for the treatment of ET in 1997, PD in 2002, and granted a special Humanitarian Device Exemption for dystonia in 2003.
Depending on the desired effects, various subcortical nuclei, such as VIM nucleus of the thalamus, GPi, STN, zona incerta, and PPN, and other subcortical nuclei and even cortical areas, have been targeted for stimulation (Benabid et al., 1991; Caparros-Lefebvre et al., 1993; Limousin et al., 1995; Benabid et al., 1996; Koller et al., 1997; Krack et al., 1998a, 1998b; Limousin et al., 1998; Ondo et al., 1998c; Pollak et al., 1998; Starr et al., 1998; Tasker, 1998; Koller et al., 1999; Limousin et al., 1999; Deep-Brain Stimulation for Parkinson’s Disease Study Group, 2001; Krause et al., 2001; Lopiano et al., 2001; Volkmann et al., 2001; Kumar, 2002; Okun and Foote, 2005; Perlmutter and Mink, 2006; Halpern et al., 2007; Stefani et al., 2007; Yu and Neimat, 2008; Limousin and Martinez-Torres, 2008) (Tables 7.2 and 7.3; Fig. 7.3).
Table 7.2 Advantages and disadvantages of deep brain stimulation
Advantages |
Disadvantages |
Table 7.3 Targets for deep brain stimulation in the treatment of various movement and other disorders
Thalamus |
Globus pallidus interna |
Subthalamic nuclei |
Other targets |
VIM, ventral intermediate nucleus of the thalamus.
DBS involves the implantation of the following hardware: (1) a DBS lead with four electrodes that are surgically inserted into the desired target and fixed at the skull with a ring and cap, (2) an extension wire that passes from the scalp area under the skin to the chest, and (3) an implantable pulse generator, a pacemaker-like device (unilateral Soletra or bilateral Kinetra, Medtronic Activa, ITREL model), which can deliver pulses with adjustable parameters (frequency, amplitude, width, modes, and polarities) (Kumar, 2002; Vesper et al., 2002). The implantable pulse generator is placed under the skin in the upper chest area near the collarbone. The patient can activate or deactivate the DBS system by placing a magnet or Access Review Device, a small mouse-like computer, over the chest area overlying the implantable pulse generator. Guidance on how to troubleshoot hardware complications and the utility of measuring impedance need to be better defined (Farris et al., 2008b). In the Medtronic Soletra and Kinetra devices the impedance measurement should be <50 Ω (ohms) and current >250 µA, but for an open circuit the impedance is usually >2000 Ω and current <7 µA for Soletra and >4000 Ω and current <15 µA for Kinetra. In seven patients with poor response to STN DBS, reimplantation of the electrodes presumably corrected misplacement and resulted in improvement in PD symptoms (Anheim et al., 2008).
In addition to appropriate selection of patients, it is critical that the most relevant and sensitive measures are used to assess the response to the therapeutic intervention, especially surgery as this intervention is particularly susceptible to a placebo effect. In this regard, instruments have been developed utilizing questionnaires, such as questions on life satisfaction: “general life satisfaction” (QLSM-A) and “satisfaction with health” (QLSM-G), in which each item is weighted according to its relative importance to the individual. In one study these instruments were validated against the 36-item short form health survey (SF-36) and the EuroQol (EQ-5D) (Kuehler et al., 2003). When the initial questionnaires were reduced to 12 items for a “movement disorder module” (QLSM-MD), and five items for a “deep brain stimulation module” (QLSM-DBS), psychometric analysis revealed Cronbach’s α values of 0.87 and 0.73, and satisfactory correlation coefficients for convergent validity with SF-36 and EQ-5D. Several quality-of-life instruments have been used in assessing the response to DBS (Kuehler et al., 2003; Diamond and Jankovic, 2005; Martinez-Martin and Deuschl, 2007; Diamond and Jankovic, 2008). Using the Sickness Impact Profile (SIP), improvements in various dimensions were maintained at 3–4 years after STN (n = 45) or GPi DBS (n = 20); 40% of STN DBS and 24% of GPi DBS patients were able to sustain their 6-month improvements at 3–4 years after surgery (Volkmann et al., 2009). In a prospective analysis of 21 patients before and after STN DBS for medically refractory PD, patients experienced an improvement in HRQoL as measured by various items of the movement disorder and health modules of the QLSM; specifically, QLSM items pertaining to energy level/enjoyment of life, independence from help, controllability/fluidity of movement, and steadiness when standing and walking showed significant improvements, although items concerning general life issues (e.g., occupational function, interpersonal relationships, leisure activities) did not improve (Ferrara et al., 2010). Thus, following STN DBS, symptomatic and functional improvements translate into higher HRQoL, with high satisfaction in domains related to movement disorders and general health. The cost associated with STN DBS has been estimated to be about $60 000 per patient over 5 years (McIntosh et al., 2003).
The mechanism of benefits of DBS produced by the electrical stimulation is not known, but in some cases improvement can be observed even before the stimulator is activated (Table 7.4). This microlesioning effect may last several days or even weeks (Maltete et al., 2009). Besides microlesion effect, the following mechanisms have been suggested to explain the beneficial effects of DBS: (1) disruption of the network (“jamming” of feedback loop from the periphery), (2) depolarization block, (3) functional ablation by desynchronizing a tremorigenic pacemaker, (4) preferential activation of large axons that inhibit GPi neurons, (5) stimulation-evoked release of GABA (Dostrovsky et al., 2002; Garcia et al., 2005), (6) release of adenosine (Bekar et al., 2008), (7) overriding of pathological bursts and oscillations and replacing them with more regular firing (Birdno and Grill, 2008; Johnson et al., 2008; Hammond et al., 2008), and (8) activation and excitation of the target nucleus (rather than inhibition as seen with lesioning) (Hilker et al., 2008). Studies have shown that electrical stimulation consisting of short (<1 ms) duration pulses preferentially activates axons rather than somas (Nowak and Bullier, 1998). In support of the hypothesis of DBS preferentially activating large axons is the observation in one patient in whom stimulation inhibited GPi firing recorded with another microelectrode 600–1000 µm away (Wu et al., 2001). The effects of stimulation appear to be restricted to an area of 2–3 mm from the macroelectrode (at 2 mA, 2 V and impedance of 1000 Ω). This would be similar to a bipolar stimulation with two adjacent contacts 1.5 mm apart. Unipolar stimulation seems to have a significantly higher efficacy than bipolar stimulation, but this is accompanied by a higher rate of side-effects (19% vs. 0%); approximately 0.4–0.5 V higher amplitude was required for bipolar than unipolar stimulation to achieve the same effect (Deli et al., 2011).
Table 7.4 Possible mechanisms of deep brain stimulation
Adapted from Hilker R, Voges J, Ghaemi M, et al. Deep brain stimulation of the subthalamic nucleus does not increase the striatal dopamine concentration in parkinsonian humans. Mov Disord 2003;18:41–48 and Bekar L, Libionka W, Tian GF, et al. Adenosine is crucial for deep brain stimulation-mediated attenuation of tremor. Nat Med 2008;14:75–80.
Some studies suggest that the observed 15–30 Hz oscillations of the STN might reflect synchronization with cortical beta oscillation via the corticosubthalamic pathway and might relate to mechanisms of bradykinesia, since stimulation at the 15 Hz rate worsens bradykinesia and dopaminergic drugs promote faster oscillations (about 70 Hz) and improve bradykinesia, similar to the high-frequency stimulation associated with DBS (Levy et al., 2002). Studying the effects of STN DBS in 1-methyl-4-phenyl-1,2,3,6-tetrahydropyridine (MPTP) monkeys, Hashimoto and colleagues (2003) showed that the activation of the STN efferent fibers results in a change in firing pattern of pallidal neurons, and they postulated that this could underlie the beneficial effects of chronic STN DBS. In contrast to the popular notion that DBS inhibits the target nucleus, DBS has been shown actually to activate the cerebellothalamocortical pathway (Molnar et al., 2004). Indeed, recent studies have suggested that stimulation-induced, time-locked modulation of pathologic network activity represents the most likely mechanism of the effects of DBS (McIntyre et al., 2004). DBS could have a dual effect, switching off a pathologically disrupted activity but also imposing a new discharge (in the upper gamma-band frequency) that results in beneficial effects (Garcia et al., 2005; Hammond et al., 2008). The most suitable target for DBS electrode is probably a region of the brain where DBS can most effectively interfere with spontaneous pathologic patterns by introducing regular rhythmical activity without causing additional adverse effects (Hammond et al., 2008). The frequency of stimulation might markedly influence the effects. For example, low-frequency (0.1–30 Hz) stimulation of STN has been shown to depolarize glutamatergic and GABAergic synaptic terminals, evoking excitatory and inhibitory postsynaptic potentials. Animal studies have shown that STN DBS (130 Hz for 1 hour) increases extracellular dopamine in the ipsilateral denervated striatum (Meissner et al., 2003). Although this has not yet been confirmed in patients, indirect evidence from [11C]raclopride PET studies has not indicated that STN DBS induces dopamine release (Hilker et al., 2003). In untreated parkinsonian animals and patients, the neuronal activity in STN and GPi is dominated by low-frequency (11–30 Hz) oscillation that is increased with levodopa to >70 Hz. The therapeutic effects of high-frequency DBS might be mediated through the same mechanism (Brown et al., 2004). The role of adenosine in the effects of DBS on tremor has been suggested by the observation of a marked increase in the DBS-induced release of ATP from local astrocytes, which metabolizes to adenosine (Bekar et al., 2008). Previous studies have demonstrated that adenosine A1 receptor activation depresses excitatory transmission in the thalamus and reduces tremor, whereas caffeine, a nonselective adenosine receptor antagonist, can trigger or exacerbate tremor. The various mechanisms by which DBS improves neurologic and psychiatric function have been a subject of several reviews (Perlmutter and Mink, 2006; Kringelbach et al., 2007; Tye et al., 2009).
The abnormal motor cortical overactivity associated with PD is reduced with STN DBS (Payoux et al., 2004). Frontal cortex function, as measured by contingent negative variation, has been improved by bilateral STN DBS (Gerschlager et al., 1999). Metabolic changes in ipsilateral premotor cortex and cerebellum bilaterally, measured by [18F]fluorodeoxyglucose and PET, correlated with clinical improvement related to GPi DBS (Fukuda et al., 2001b). Other regional cerebral blood flow studies showed that when the STN DBS is on, the ipsilateral rostral supplementary motor area and premotor cortex are activated during contralateral movement, but there was a reduction in regional cerebral blood flow in primary motor cortex during rest (Ceballos-Baumann et al., 1999). Modulation of cortical activity may be also responsible for improved bladder function with STN DBS (Herzog et al., 2006).
The placement of the electrode can be verified radiographically. Although computed tomography scan, rather than MRI, has been recommended by some, several studies have concluded that standard MRI (Tronnier et al., 1999; Jech et al., 2001) and functional MRI (Arantes et al., 2006) can be safely performed in patients with implanted neurostimulation systems, although transient and permanent neurologic deficits have been rarely reported following MRI (Henderson et al., 2005; Rezai et al., 2005; Spiegel et al., 2003).
Using PET, Ceballos-Baumann and colleagues (2001) found that VIM DBS in patients with ET was associated with increased regional cerebral blood flow in the ipsilateral motor cortex and a decrease in regional cerebral blood flow in the retroinsular (parietoinsular vestibular) cortex. They suggested that the latter affects function of the vestibular-thalamic-cortical projections and might therefore explain the frequent occurrence of disequilibrium in patients treated for tremor with VIM DBS, a reversible complication of this therapy. The authors also postulated that the increased synaptic activity in the motor cortex overrides the abnormal tremor-related rhythmic neuronal bursting. In another study (Ceballos-Baumann et al., 2001), the authors suggested that the beneficial effects of VIM DBS are due to nonphysiologic activation of thalamofrontal projections or frequency-dependent neuroinhibition. This has been also confirmed by Perlmutter and colleagues (2002). In another study involving functional MRI during DBS of STN (three patients) and thalamus (one patient), Jech and colleagues (2001) showed an increase in blood oxygenation level-dependent signal in the subcortical regions ipsilateral to the stimulated nucleus. The authors concluded that this effect cannot be simply explained by a mechanism of depolarization blockade; rather, it is caused by “overstimulation” of the target nucleus resulting in the suppression of its spontaneous activity.
DBS in tremor
Thalamic stimulation appears to be particularly effective in the treatment of parkinsonian tremor and ET (Koller et al., 1997; Ondo et al., 1998c; Pollak et al., 1998; Koller et al., 1999; Pahwa et al., 1999; Koller et al., 2001; Krauss et al., 2001b; Rehncrona et al., 2003) (Videos 7.1 and 7.2). In the North American Multi-Center Trial, 25 ET and 24 PD patients were followed for 1 year after implantation (Koller et al., 1997). Combined blinded tremor ratings (0–4) in ET patients randomized to “on” were 0.9 compared to 2.7 for those randomized to “off” stimulation. All subjective functional measures improved, and 9 of 29 patients (31%) had complete tremor cessation. In PD patients, “on” randomized scores were 0.6 compared to 3.2 for those who were randomized to “off.” Fifty-eight percent (14 of 24) of patients had complete tremor cessation. Subjective functional measures (UPDRS part II), however, were not significantly improved. Complications were manageable and included paresthesia, headache, disequilibrium, dystonia, and device failure. Results were similar at 1 month and 1 year after implantation. Although some loss of efficacy and device-related complications have been encountered after 2 years of follow-up, the authors concluded that unilateral DBS of the thalamus has long-term efficacy in patients with ET (Koller et al., 2001). In a multicenter European study in which 37 patients with ET were followed for a mean of 6.5 years, unilateral or bilateral VIM DBS offered long-term benefits and safety (Sydow et al., 2003). Our experience at Baylor is similar to that reported in other centers. In a blinded and open-label trial of unilateral thalamic DBS in 33 patients (14 ET and 19 PD) with severe tremor refractory to conventional therapy, ET and PD patients demonstrated an 83% and 82% reduction (P < 0.0001), respectively, in observed contralateral arm tremor (Ondo et al., 1998c). All measures of tremor, including writing samples, pouring tests, subjective functional surveys, and disability scores, significantly improved. We found that bilateral thalamic DBS is more effective than unilateral DBS in controlling bilateral appendicular and midline tremors of ET and PD, and thalamic DBS does not seem to improve meaningfully any parkinsonian symptoms other than tremor (Ondo et al., 2001a). Although unilateral VIM DBS can markedly improve midline tremor, this improvement is significantly enhanced by the bilateral procedure (Putzke et al., 2005). Several studies have found that gait and balance may be impaired in some, but not all, patients treated for ET with VIM DBS (Earhart et al., 2009). One long-term study found that bilateral VIM DBS was often associated with dysarthria, loss of balance, and incoordination (Pahwa et al., 2006). Similar findings were reported in a 6-year follow-up of 38 PD patients treated with VIM DBS in a multicenter European study (Hariz et al., 2008b). The authors suggest that unilateral VIM DBS should be reserved for elderly PD patients with predominant unilateral tremor, but STN DBS may be a better choice for other patients with tremor-dominant PD. This recommendation is supported by other studies showing benefit of STN DBS in PD patients with prominent tremor (Fraix et al., 2006; Diamond et al., 2007b). VIM DBS has been found to be associated with modest improvement, rather than tremor augmentation as was previously suggested, in ipsilateral tremor in patients with ET (Ondo et al., 2001c). Other studies have demonstrated bilateral effects of unilateral thalamic DBS (Kovacs et al., 2008). A review of long-term efficacy of VIM DBS in 39 patients (20 PD, 19 ET) showed that the benefits might be maintained for at least 6 months (Rehncrona et al., 2003). In one study, three of eight patients with PD no longer required DBS after 3–5 years because the tremor markedly improved (Kumar et al., 2003). In addition to reducing the amplitude, VIM DBS increased the frequency of ET by 0.5–2 Hz at low inertial loads, made the tremor more irregular, and reduced the tremor-electromyography coherence (Vaillancourt et al., 2003).
To compare thalamic DBS with thalamotomy, Schuurman and colleagues (2000) conducted a prospective, randomized study of 68 patients with PD, 13 with ET, and 10 with multiple sclerosis. They found that the functional status improved more in the DBS group than in the thalamotomy group, and tremor was suppressed completely or almost completely in 30 of 33 (90.9%) in the DBS group and in 27 of 34 (79.4%) in the thalamotomy group. Although one patient in the DBS group died after an intracerebral hemorrhage, DBS was associated with significantly fewer complications than was thalamotomy. Similar results were obtained on this group after a 5-year follow-up, with the outcomes still favoring the DBS group (Schuurman et al., 2008). In one study of VIM DBS, dysarthria worsened when both stimulators were turned on in three of six patients (Pahwa et al., 1999). DBS also has been found to be effective in rare patients with disabling task-specific tremors (Racette et al., 2001). In addition to improving distal tremor associated with PD and ET, VIM DBS can effectively control ET-related head tremor, which usually does not respond to conventional therapy (Koller et al., 1999). Other midline tremors, such as voice, tongue, and face tremor, also may improve with unilateral VIM DBS, although additional benefit can be achieved with contralateral surgery (Obwegeser et al., 2000; Putzke et al., 2005). In addition, VIM DBS appears to improve postural stability (Pinter et al., 1999). Furthermore, unilateral thalamic DBS for ET has been found to be cognitively safe and to improve anxiety and quality of life (QoL) in terms of ADL and psychologic well-being (Tröster et al., 1999). Several studies have demonstrated that VIM DBS improves ADL and QoL, and one study showed that these improvements may be sustained for up to 7 years after implantation (Hariz et al., 2008a).
Several studies have sought to determine the optimal stimulation parameters. Algorithms for programming of the stimulation parameters have been suggested (Volkmann et al., 2006) but programming is generally based on the judgment of the programmer. Charge is the product of pulse width and current (current = voltage/impedance). Charge density is defined as the charge divided by the geometric surface area of the electrode. Short pulse widths require high current but low charge, and are thus more efficient at exciting neural elements and reducing the risk of neural damage. Linear voltage increases above 3 V do not correlate to a linear increase in the volume of neural elements excited, but significantly increase power consumption (Kuncel and Grill, 2002). While the amount of tremor suppression does not substantially increase in frequencies above 100 Hz, power consumption increased markedly at the higher frequencies (Kuncel et al., 2006). Power consumption (and battery drain) is determined by calculating the voltage squared (V2) times the pulse width (PW) and frequency (F) divided by the impedance (Z).
Bin-Mahfoodh et al. (2003) evaluated 14 implantable pulse generators (IPGs) that needed battery replacement out of 163 single channel batteries implanted over 7 years and found that the higher the total electrical energy delivered as calculated by the power consumption formula, the shorter the battery lifespan. A review of all battery replacements in patients at Baylor College of Medicine found 122 batteries needed replacement in 73 patients. The main predictors of a shorter battery life were greater amplitude, pulse width, and not using exclusive bipolar settings (Ondo et al., 2007). O’Suilleabhain et al. (2003), studied 13 VIM thalamic stimulators (8 for ET and 5 for PD) and measured tremor with an electromagnetic tracker. They randomly programmed 78 combinations of voltages (0, 1, 2, 3, or 4 V), pulse widths (60, 90, or 120 µs), and frequencies (130, 160, or 185 Hz) for both monopolar and bipolar polarity configurations. The voltage response curve for ET was flatter than for PD patients. Monopolar configurations were up to 25% more effective than bipolar but this configuration tended to shorten calculated battery life by 35%. The longest pulse width tested was up to 30% more effective than the shortest, but frequency changes had little effect on tremor amplitude. The authors recommended that there was no need to increase the frequency above 130 Hz and another study concluded that stimulation frequency of 100 Hz is generally the optimal frequency and that there is little if any additional benefit from a higher frequency (Ushe et al., 2006). Low-frequency (10 Hz) STN DBS has been shown to improve verbal fluency but worsen motor function in a double-blind randomized crossover study of 12 patients with PD (Wojtecki et al., 2006). Moro et al. (2006) studied 44 patients with PD who had a long-term (mean, 3.5 years), stable response to STN DBS. Each underwent reprogramming of their stimulation by a neurologist expert in both PD and DBS, along with medication adjustments. Scores on the UPDRS were compared before reprogramming and up to 14 months thereafter. In half the patients (24, or 54.6%), UPDRS parts II and III scores improved significantly after reprogramming, by 15.0% and 29.5%, respectively. Anti-PD drug use decreased significantly as well, by 29.5%, in these responders. No improvement was seen in 16 patients (36.4%), and the condition of 4 patients (9.1%) worsened. These results suggest that patient outcomes with STN DBS can be improved when a neurologist expert in movement disorders and DBS programming is directly responsible for patient care.
VIM DBS has been found to be useful not only in the treatment of troublesome tremors associated with ET and PD but also in orthostatic tremor (Espay et al., 2008), and cerebellar outflow tremor associated with multiple sclerosis and post-traumatic tremors (Montgomery et al., 1999; Matsumoto et al., 2001; Wishart et al., 2003; Lyons and Pahwa, 2008) (Video 7.3). Thalamic DBS may be also effective in the treatment of levodopa-induced dyskinesia, possibly owing to involvement of the center median and parafascicularis complex (Caparros-Lefebvre et al., 1999). Thalamic DBS, however, does not seem to provide any benefit in PD-related gait difficulty (Defebvre et al., 1996). Bilateral ventralis oralis anterior thalamic DBS has been also reported to be effective in a patient with severe postanoxic generalized dystonia and bilateral necrosis of the basal ganglia (Ghika et al., 2002). Medically intractable myoclonus was reported to improve 80% with VIM DBS in one case of myoclonus–dystonia syndrome (Trottenberg et al., 2001a), and GPi DBS has been found to be effective in relieving myoclonus–dystonia syndrome (Magarinos-Ascone et al., 2005).
In addition to VIM as a target for patients with disabling tremor, STN DBS has been also found to be useful in the treatment of severe proximal tremor (Kitagawa et al., 2000) as well as rest and postural tremor in PD (Sturman et al., 2004).
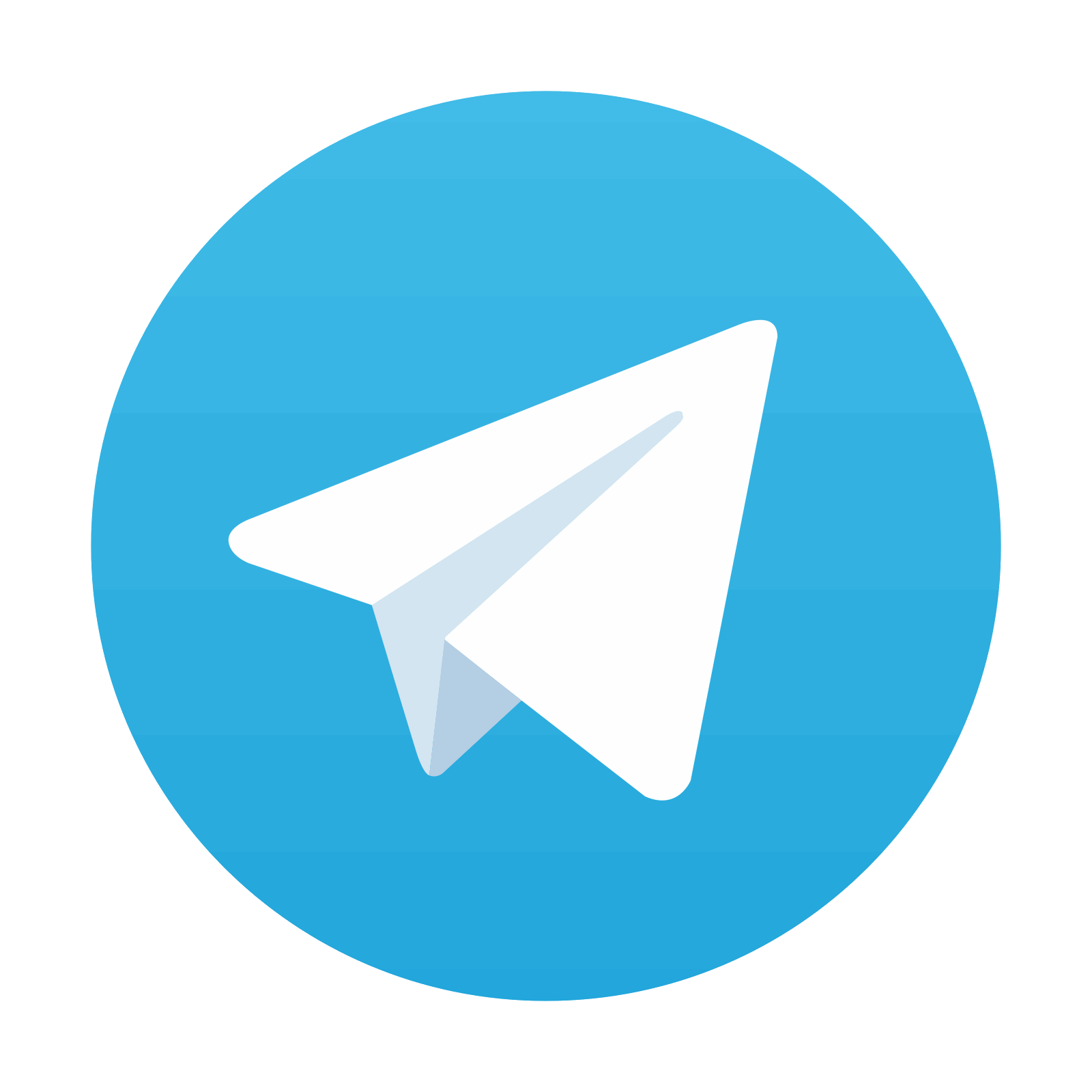
Stay updated, free articles. Join our Telegram channel

Full access? Get Clinical Tree
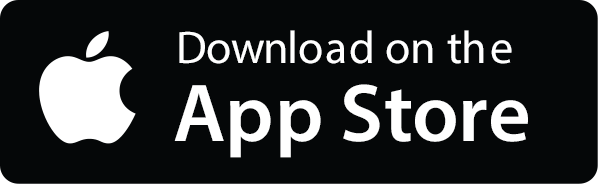
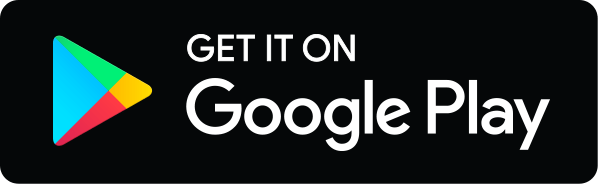