FIGURE 17.1 The right osseous labyrinth in the temporal bone viewed from above.
FIGURE 17.2 The right osseous labyrinth, lateral view.
The bony labyrinth is filled with perilymph, a thin watery fluid similar to cerebrospinal fluid. The membranous labyrinth is an arrangement of sacs and ducts that lies within the bony labyrinth, generally follows its outline, and is filled with endolymph (Scarpa’s fluid [Antonio Scarpa was an Italian surgeon, anatomist, and artist who first described many structures of the ear]). The membranous labyrinth has two major components: the vestibular apparatus and the cochlear duct (Figure 17.3). The ossicles span the middle ear cavity and transmit the oscillations of the tympanic membrane to the footplate of the stapes, which sits in the oval window (fenestra vestibuli). The ossicles function as an amplifier and help to compensate for the loss of energy as sound waves are transmitted from the air to the perilymph behind the oval window. The tensor tympani muscle, which inserts on the malleus, and the stapedius, which inserts on the stapes, provide reflex protection against sudden, loud noise. The oval window opens into the vestibule of the inner ear, which connects on one side to the cochlea and on the other to the semicircular canals. The cochlea spirals for 2.5 to 2.75 turns to reach its apex. The base of the cochlea faces the internal acoustic meatus and contains myriad fenestrations that admit the filaments of the cochlear nerve. The middle ear cavity acts as an impedance-matching device to transfer sound energy from the low impedance of air to the high impedance of fluid in the cochlea.
FIGURE 17.3 The membranous labyrinth.
The central axis of the cochlea is the modiolus; from it projects a delicate bony shelf, the spiral lamina, which partially divides the cochlear passageway into two parallel channels—the scala tympani and the scala vestibuli. The scala media, or cochlear duct, is part of the membranous labyrinth. It lies in the center of the spirals of the cochlea, completing the partition between the scala tympani and scala vestibuli (Figure 17.4). At the tip of the modiolus, the cochlear duct ends blindly; a narrow slit at the very apex of the cochlea, the helicotrema (Gr. “hole in a helix”), allows for communication and the flow of perilymph between the scala tympani and vestibuli.
FIGURE 17.4 Structure of the cochlear duct and the spiral organ of Corti. (Modified from Kiernan JA. Barr’s: The Human Nervous System: An Anatomical Viewpoint. 7th ed. Philadelphia: Lippincott Williams & Wilkins, 1998, with permission.)
The basilar membrane of the cochlear duct projects from the spiral lamina of the modiolus to the outer wall of the cochlea. The spiral ganglion of the cochlear nerve lies in the spiral canal of the modiolus (Rosenthal’s canal). The organ of Corti rests on the basilar membrane and contains inner and outer hair cells. The inner hair cells are the receptors, or end organs, of the cochlear nerve. From the apex of each inner hair cell, a stereocilium extends to just beneath the tectorial membrane (Figure 17.4). Sound waves induce vibrations in the cochlea, which cause movement of the basilar and tectorial membranes. This movement flexes the stereocilia, which activates the hair cell, causing impulses in the spiral ganglion.
Because of the varying width of the basilar membrane, sound of a certain frequency induces harmonic oscillations maximal at a certain point along the cochlear duct, which focally activates certain hair cells and encodes the frequency (Box 17.1). The round window (fenestra cochlea) lies below the oval window (Figure 17.2). It is covered by a delicate membrane, the secondary tympanic membrane, which allows for compliance in the perilymph system and permits the waves of vibration initiated at the oval window to dissipate.
Tonotopic Organization
The organ of Corti is tonotopically organized. The width of the basilar membrane of the cochlear duct is least at the base of the cochlea, where the spinal lamina of the modiolus extends farthest into the coils of the cochlea. This part of the cochlea is most efficiently activated by high frequencies. Near the apex, the basilar membrane is wider and responds to low-pitched tones. The inner hair cell-spiral ganglion cell complex at a given point along the organ of Corti is frequency dependent, responding best to a particular pitch and coding for that pitch by its discharges in the cochlear nerve. Tonotopic organization continues to varying degrees throughout the auditory system.
Imagine a spiral staircase in the center of a silo—steps winding around a central core, steps wider at the bottom and progressively narrowing, and from each step a cable of violin string extending to the wall of the silo (Figure 17.5). The central core represents the modiolus; the steps, the spiral lamina; and the violin strings, the basilar membrane. A low tone sounding in the silo would set the long strings near the top of the silo in vibration; a high-pitched tone would affect the short strings near the bottom. Coil the silo into a conch to match the turns in the staircase to complete the resemblance to the cochlear duct.
FIGURE 17.5 The cochlear duct and organ of Corti as a spiral staircase in a silo (see box).
The spiral ganglion consists of type I and type II bipolar neurons that lie in the modiolus. Inner hair cells synapse on type I neurons, which make up 95% of the ganglion. Axons of the spiral ganglion cells form the cochlear nerve, which contains some 30,000 fibers (Figure 17.6). Axons from type I cells are myelinated and form the bulk of the nerve. The type II cells connect with the outer hair cells and modulate the activity of the inner hair cells (Box 17.1).
FIGURE 17.6 The acoustic nerve and its connections.
The acoustic nerve traverses the internal auditory canal (IAC), where it lies lateral and inferior to the facial nerve. It crosses the cerebellopontine angle, passes around the inferior cerebellar peduncle, and enters the upper medulla at its junction with the pons near the lateral recess of the fourth ventricle (Figure 17.7). Each entering fiber bifurcates to synapse in both the dorsal (posterior) and ventral (anterior) cochlear nuclei. The ventral nucleus may be divided into anteroventral and posteroventral portions. This dual termination is the beginning of a great deal of redundancy in the auditory system.
FIGURE 17.7 Section through the junction of the pons and medulla at the level of the cochlear nuclei.
Tonotopic organization is maintained in the auditory nuclei and throughout the higher auditory relay centers; the location of fibers is related to their site of origin in the cochlea, which in turn is a reflection of the activating frequency. In the cochlear nuclei, low-frequency tones are processed ventrally, and high frequencies dorsally. Second order neurons in the cochlear nuclei give rise to the dorsal, ventral, and intermediate acoustic stria. The dorsal acoustic stria consists of fibers from the dorsal cochlear nucleus that pass over the inferior cerebellar peduncle, cross the floor of the fourth ventricle under the striae medullares (fibers of Piccolomini), then pass ventrally into the pons, near the superior olivary nucleus, to join the contralateral lateral lemniscus (Figure 17.8). The intermediate and ventral acoustic striae arise from the ventral cochlear nuclei. The intermediate stria passes over the inferior peduncle and crosses the tegmentum to join the contralateral lateral lemniscus. Fibers of the ventral stria pass ventral to the peduncle. Some cross the pons as trapezoid fibers to synapse in the contralateral nucleus of the trapezoid body. Others synapse ipsilaterally in the nucleus of the trapezoid body.
FIGURE 17.8 The cochlear pathway.
Auditory fibers ascend from the trapezoid body as the lateral lemniscus. Fibers in the dorsal and intermediate acoustic stria run to the contralateral inferior colliculus, most directly, some after a relay in the nucleus of the lateral lemniscus. This crossed, monaural auditory pathway primarily carries information about sound frequency. Fibers of the ventral acoustic stria are both crossed and uncrossed and may synapse in the nuclei of the trapezoid body, superior olive, or lateral lemniscus. The binaural pathway, especially the superior olivary complex component, can determine the time difference between the two ears and aid in the localization of sound. Ascending auditory fibers send collaterals to the brainstem reticular formation and to the nuclei of CN V and VII; these connections mediate various reflexes related to hearing.
The stereocilia of the outer hair cells are imbedded in the tectorial membrane and have contractile properties. They help adjust and control the oscillations of the membrane and thereby regulate to some degree the activation of the inner hair cells. The outer hair cells receive innervation from the efferent cochlear, or olivocochlear, bundle, which arises from the superior olivary nucleus in the pons. By controlling the outer hair cells, the olivocochlear bundle helps regulate afferent cochlear traffic and may be involved in attentiveness to auditory stimuli.
Fibers from the lateral lemnisci ascend to synapse in the central nucleus of the inferior colliculus, an auditory reflex center that is also tonotopically organized. The inferior colliculus is the central relay nucleus of the auditory pathway and receives both ascending and descending input. Axons from the inferior colliculus pass through the brachium of the inferior colliculus to the medial geniculate body (MGB), a special sensory nucleus of the thalamus that is the final relay station in the auditory pathway. In the MGB, fibers conveying high tones lie medially and low tones laterally. From the MGB, auditory fibers pass through the posterior limb of the internal capsule as the geniculotemporal tract, or auditory radiations, which runs through the sublenticular portion of the internal capsule. The fibers terminate in the cortex of the transverse temporal convolutions (Heschl’s gyrus) and the adjacent planum temporale portion of the superior temporal gyrus. The transverse temporal gyri and parts of the planum temporale make up the primary and secondary auditory cortex (Brodmann’s areas 41 and 42). The primary auditory cortex is tonotopically organized with high frequencies medial and low frequencies lateral. The auditory association cortex (Wernicke’s area in the dominant hemisphere) lies just posterior to the primary auditory cortex.
There is extensive crossing of the central auditory pathways above the level of the cochlear nuclei. Commissures connect the nuclei of the lateral lemniscus (commissure of Probst) and the inferior colliculi (commissure of the inferior colliculus). There are connections through the brachium of the inferior colliculus between the central nucleus of the inferior colliculus on one side and the contralateral MGB. In addition, there is the direct, tonotopically organized auditory pathway, the core projection, and an additional, less-organized pathway, the belt projection. The core system includes the central nucleus of the inferior colliculus, portions of the MGB, and the primary auditory cortex. The belt projection includes the pericentral region of the inferior colliculus, the nonlaminated portion of the MGB, and the secondary auditory cortex. The corpus callosum contains fibers that connect the auditory cortices of the two hemispheres.
Descending auditory projections run parallel to the ascending fibers and are concerned with auditory reflexes. Descending pathways include the corticogeniculate, corticocollicular, geniculocollicular, and collicular efferents. The efferent cochlear bundle projects from the superior olive to the cochlea (Box 17.1).
Clinical Examination
Some information about hearing may be obtained simply by observation and gauging the patient’s ability to understand soft and loud tones and low and high pitches; note signs of deafness, such as a tendency to turn the head when listening, lip reading, or speaking with a loud voice. Any history of hearing difficulty, such as trouble using the telephone or hearing conversation in noisy environments, or complaints from family members, should prompt a careful evaluation. Before testing hearing, otoscopic examination should be done to ensure the tympanic membrane is intact and to exclude the presence of wax, pus, blood, foreign bodies, and exudate. The mastoid region should be examined for swelling and tenderness.
Conductive hearing loss (CHL) is that due to impaired conduction of sound to the cochlea and may be due to occlusion of the external auditory canal, middle ear disease (e.g., otitis), or abnormality of the ossicular chain (e.g., otosclerosis). Sensorineural hearing loss (SNHL) is that due to disease of the cochlea (e.g., Ménière’s disease) or eighth CN (e.g., acoustic neuroma). As a generality, CHL affects low frequencies and SNHL affects high frequencies. Ménière’s disease is a notable exception, causing predominantly low-frequency hearing loss, at least early in the course. Central hearing loss is that due to disease of the central pathways. Central hearing loss is very rare because of the bilaterality and redundancy of the auditory system; unilateral lesions of the central auditory pathways typically do not cause any deficit detectable by routine clinical testing.
There are many ways to assess hearing at the bedside. All are crude compared to the information that can be obtained with a formal audiogram. The subject of audiography is complex. Box 17.2 summarizes some of the basic principles.
Audiometry
Detailed assessment of hearing is done with audiometry, which is usually performed as a battery of tests. In the past, audiometry required patient cooperation for reliable results, but increasingly objective methods have been developed that allow for hearing assessment in patients where reliable voluntary behavioral responses cannot be obtained. The range of human hearing is 20 to 20,000 Hz (about 11 octaves). Speech usually falls in the 300-to 3,000-Hz range. Auditory acuity declines with age, especially for higher frequencies.
An audiometer is an instrument by which sounds of varying intensity and frequency are presented to a patient. There are many different audiologic techniques; those used most commonly for neurologic purposes are pure tone and speech audiometry. An audiogram is a plot of the threshold of audition for short pure tones as a function of frequency on a logarithmic decibel scale. Air conduction (AC), assessed with earphones, tests the entire auditory pathway. Bone conduction (BC) sends a signal directly to the cochlea, bypassing the outer and middle ear structures. White noise is often presented to the untested ear (masking). The pure tone audiogram displays the severity of any hearing loss in relation to established reference values, and the pattern may suggest the etiology. As with tuning fork testing, a decrease in AC with normal BC, an air-bone gap, indicates conductive hearing loss, and a decrease in both AC and BC indicates sensory or neural loss. The pure tone audiogram is usually normal with lesions involving the central auditory pathways.
Speech audiometry uses spoken words and sentences instead of pure tones. The speech reception threshold is considered the intensity level at which the patient can correctly understand 50% of the material presented. Speech discrimination, or intelligibility, is the proportion of the material the patient can understand when presented at a level that should be easily heard. The loss of discrimination is proportional to the severity of the hearing loss in patients with cochlear lesions. Poor speech discrimination, out of proportion to pure tone hearing loss, is characteristic of a retrocochlear lesion, such as a cerebellopontine angle tumor. In cranial nerve (CN) VIII lesions, discrimination may even paradoxically decline as intensity is raised.
Impedance audiometry uses an electroacoustic device, which measures the impedance, or compliance, of the conductive hearing mechanism, like measuring the tightness of a drumhead. A very stiff drumhead has high impedance, or low compliance, and reflects sound back to the source. Low impedance allows for greater transmission of sound through the system and less reflection. A tympanogram measures the impedance of the tympanic membrane. An abnormal tympanogram is seen in such conditions as otitis media, tympanic membrane perforation, ossicular dislocation, otosclerosis, cerumen impaction, and eustachian tube dysfunction.
Contraction of the stapedius muscle changes the compliance in the conductive pathways. The stapedius reflex, or acoustic reflex, measures the change in compliance in response to loud sounds to assess the function of the stapedial muscle. The reflex arc is via CN VIII, brainstem interneurons, and CN VII. In the absence of severe hearing loss, an abnormal stapedius reflex may suggest a lesion of CN VII or VIII or the brainstem. Békésy audiometry, primarily of historical interest, compares the hearing for continuous and pulsed tones. Its usefulness has been supplanted by neuroimaging, auditory evoked potentials (AEPs), and other audiometric techniques.
The AEP, also known as the auditory evoked response, or brainstem auditory evoked potential/response (BAEP/BAER), is a minuscule potential produced by auditory stimuli and recorded using electroencephalogram (EEG) electrodes. The potential is discernible from the much more prominent background EEG activity because it is time locked to the auditory stimuli. This temporal relationship is only apparent after averaging a large number of responses. The background EEG activity is random and not time locked to the stimuli. It tends to cancel itself out if enough auditory stimuli are given, and the signal (the BAER) gradually emerges from the noise (the background EEG activity). The waves that occur in the first 10 ms after an auditory stimulus are short-latency far-field potentials due to electrical activity at various points along the auditory pathway. These are reproducible and reliable wave forms. BAERs are used primarily for evaluating suspected CN VIII and brainstem lesions. There are five to seven waves in the AEP; the correlation of the waveforms with specific anatomic structures is based primarily on animal studies and remains somewhat uncertain. Wave I is the auditory nerve action potential. Wave II is thought to reflect activity in the cochlear nuclei, although it may be generated by the intracranial segment of the auditory nerve. Wave III is thought to come from the superior olive, and waves IV and V, the inferior colliculus. The origin of waves VI and VII is unclear, but wave VI may come from the medial geniculate body and wave VII from the auditory radiations.
The relationship of the BAER waveforms to the anatomic pathways allows for lesion localization predicated on changes in interpeak latencies and differences in latencies between the two ears. A delay between waves I and III suggests a lesion between the eighth nerve near the cochlea and the lower pons; an interpeak latency delay between waves III and V suggests a lesion between the lower pons and midbrain. The primary clinical applications of BAERs have been in the evaluation of patients with cerebellopontine angle tumors and demyelinating disease, and in coma and brain death. It is also of considerable value in newborn and infant hearing assessment.
Bedside clinical testing of hearing may theoretically use any available instrument that is capable of making a sound. Because the ability to hear and understand speech is the most important functional aspect of audition, whispered voice is useful. Inability to whisper at exactly the same level for testing each ear, and inter-and intraindividual variation in the intensity and pitch of the voice, is a theoretical limitation, but clinically significant hearing loss is usually detectable with this simple technique. Whispered voice has been recommended as an excellent screening test. Inability to perceive a whispered voice has a likelihood ration of 6.1 (95% CI, 4.5 to 8.4) for clinically significant hearing loss; normal perception has an LR of 0.03 (95% CI, 0 to 0.24).
Despite its disability for the patient, high-frequency SNHL of the type associated with presbycusis and acoustic trauma is not generally of neurologic significance; the use of high-pitched sounds, such as a ticking watch, seldom provides useful information for neurologic examination purposes. In certain types of deafness, loss of speech discrimination is of clinical significance, even though pure tone and even speech thresholds are normal. Few conditions of neurologic importance cause bilaterally symmetric hearing loss, and an examination designed to detect auditory asymmetry usually suffices. Other useful sounds for bedside testing include finger rub—the noise made by rubbing the thumb and index finger together beside the external auditory meatus—and pure tones created by a tuning fork.
Detailed testing of hearing is done monaurally, ideally while occluding the opposite ear, as by pressing the tragus over the canal. For screening purposes in low-yield situations, occlusion of the opposite ear is sometimes omitted, and occasionally finger rub is done binaurally. In each instance, the patient is asked to compare the sound intensity between the two ears. The examiner may also compare the distance from each ear at which a sound of the same intensity can be heard. An occasionally useful method is to place the earpieces of a stethoscope into the patient’s ears. Then whisper into, scratch softly on, or hold a tuning fork to the chest piece, and ask the patient to compare the sounds heard. One side of the tubing can be occluded to direct the sound into one ear. Ross’ method is to stand at a fixed distance (e.g., 6 ft) at a right angle from the patient, have the patient occlude the far ear, and whisper; repeat for the other ear, and compare the auditory acuity on the two sides.
When using whispered voice, certain tones are heard better and at a greater distance than others. Sibilants, and the short vowels such as a, e, and i, are heard at a greater distance than broad consonants such as 1, m, n, and r, and such vowels as o and u. “Seventy-six” and “sixty-seven” can be heard at a greater distance than “ninety-nine” and “fifty-three.” One key to the effective use of whisper is unpredictability of the stimulus, for example, the numbers “1, 2, 3” in one ear and “7, 8, 9” in the other. Monosyllables are preferable to common stock questions such as “How are you?” in which hearing a small part may enable the patient to “hear” the rest in context. Alternating words and numbers is a challenging test of hearing.
In the CALFRAST (calibrated finger rub auditory screening test), the patient’s hearing of a strong or faint finger rub at various distances is compared to the examiner’s. The examiner’s arms fully extended at a distance of 6 to 10 inches approximates 70 cm. The ability to hear the softest rub that the examiner can hear with arms fully extended is normal. With inability to hear at this distance, the stimuli are brought closer (35 cm is approximately equal to examiner’s elbows flexed 10 cm to a handbreadth and 2 cm as close as possible without touching) until the patient can hear. The CALFRAST was judged simple, accurate, inexpensive, and reliable. All subjects unable to hear strong finger rub with full arm extension had hearing impairment that ranged from mild to severe on audiometric testing.
Tuning forks—typically 128, 256, or 512 Hz—are sometimes used to give more specific information and to assess air conduction (AC) and bone conduction (BC). When testing AC, the tuning fork should be kept in gentle motion to avoid null points. The patient may be asked to compare the loudness of the vibrating fork in the two ears, or the examiner may compare the distance on each side at which the fork begins or ceases to be heard. The gradual dampening of the oscillations makes precision difficult. The examiner with good hearing may compare the patient’s air and BC with his own (Schwabach test). In evaluating BC, be certain the patient hears rather than feels the tuning fork. How useful tuning fork tests are for general screening has been questioned. But the primary usefulness of both the Weber and Rinne tests (see below) is not as a screening tool but to make an initial differentiation between SNHL and CHL in a patient complaining of unilateral symptoms of hearing loss or tinnitus.
The Rinne test compares the patient’s AC and BC; it can be done in at least two ways. An activated fork may be placed first on the mastoid process, then immediately beside the ear (or vice versa), and the patient is asked which is louder; it should always be louder by the ear. The more time-consuming, traditional method is to place the tuning fork on the mastoid and when no longer heard there move it beside the ear, where it should still be audible. The fork should be heard twice as long by AC as by BC. The Rinne test is normal or positive when AC is better than BC either by subjective assessment of loudness or by the length of time the tuning fork is heard in the two locations. In CHL, BC is better than AC, and the Rinne is said to be negative. Sound is not conducted normally through the canal or from the tympanic membrane through the ossicular chain to the cochlea, but the sensorineural mechanisms are intact; AC is impaired but BC is preserved. In CHL, BC may even be exaggerated beyond the normal because the middle ear cavity becomes a resonating chamber. In sensorineural deafness, both AC and BC are impaired while retaining their normal relationship of AC better than BC; the Rinne is positive or normal. With severe sensorineural deafness, BC may be lost while slight AC is preserved. Because of inconsistent use of the terms positive and negative, it is preferable to state that AC is better than BC or that the Rinne is normal.
In the Weber test, a vibrating tuning fork is placed in the midline on the vertex of the skull. It may be placed anywhere in the midline, over the nasal bridge, forehead, or maxilla, but works best over the vertex. Normally, the sound is heard equally in both ears or seems to resonate somewhere in the center of the head; it is “not lateralized.” In CHL, the sound is heard better (“lateralized”) to the involved side. A simple way to remember this phenomenon is for the examiner to place a tuning fork over his own vertex and then induce conductive loss by inserting a finger in one ear; the sound will be louder on the side of the occluded canal. In sensorineural deafness, the sound is heard best in the normal ear.
In summary, with unilateral CHL, AC is less than BC (the Rinne is negative), and the Weber lateralizes to the involved side. In unilateral SNHL, AC is greater than BC (Rinne positive or normal), and the Weber lateralizes to the normal side (Table 17.1).
TABLE 17.1 Rinne and Weber Tests
Auditory reflex responses are occasionally useful in evaluating hearing in children, patients with altered mental status, and in hysteria or malingering. The auditory-palpebral reflex (auro-or acousticopalpebral, cochleopalpebral, or cochleoorbicularis reflex) is a blink or reflex eye closure in response to a loud, sudden noise. The cochleopupillary reflex is pupillary dilation, or contraction followed by dilation, in response to a loud noise. The auditory-oculogyric reflex is eye deviation toward a sound. The general acoustic muscle reflex is a general jerking of the body in response to a loud, sudden noise.
The laboratory evaluation of hearing is done primarily by electronic audiometry and auditory evoked potentials. These are discussed in Box 17.2.
Disorders of Function
Dysfunction of the cochlear nerve and its connections usually causes either diminution or loss of hearing (hypacusis or anacusis), with or without tinnitus. Hyperacusis occurs most often with paralysis of the stapedius muscle in disorders affecting CN VII, but it may occur as an epileptic aura, in migraine (sonophobia or phonophobia), and in certain psychiatric conditions and drug-related disorders. Dysacusis (sometimes called auditory agnosia) is impairment of hearing that is not primarily a loss of auditory acuity; rather, it is related to dysfunction of the cochlea or central auditory pathways. Diplacusis is a condition in which there is a difference in the pitch or intensity of the same sound as heard in the two ears, or when a single sound is heard as having two components; it is usually due to disease of the cochlea. Paracusis is perversion or distortion of hearing. Paracusis of Willis (paracusis willisii) is an interesting phenomenon in which the ability to hear improves in the presence of loud noises. Thomas Willis described a patient who heard better when a drum was beating loudly nearby. It is a feature of otosclerosis. Disturbances of hearing due to CNS lesions are rare.
CHL is due to interference with the transmission of sound to the cochlea. SNHL is due to disease of the cochlea or its central connections. In essence, CHL is due to disease external to the oval window, and SNHL is due to disease central to the oval window. With CHL, there is primarily loss of AC; BC is preserved or even exaggerated (Table 17.1). The Schwabach response is shortened; the Weber is referred to the involved side. Low tones are lost, as are some of the broad or flat consonants and vowels such as m, n, l, r, o, and u. Impairment of speech discrimination parallels the loss for pure tones. There is no recruitment, and tone decay is normal. Patients with CHL tend to hear speech better in a noisy background than in a quiet setting. Mixed hearing loss, with elements of both CHL and SNHL, is not uncommon. Some causes of hearing loss are listed in Table 17.2.
TABLE 17.2 Causes of Hearing Loss
With unilateral SNHL, AC and BC are both diminished, but AC remains better than BC (Table 17.1); the Schwabach response is shortened, and the Weber lateralizes to the normal ear. The hearing loss is worse for higher frequencies (Figure 17.9), and there is greater difficulty with sibilants, sharp consonants, and short vowels (e.g., in the words sister, fish, twenty, water, and date). A clearly enunciated whisper is sometimes more easily understood than a loud, indistinct shout.
FIGURE 17.9 Audiogram of a patient with sensorineural deafness.
SNHL may be due to disease of the cochlea (end-organ deafness), such as in Ménière’s disease, or to disease of CN VIII or more central structures (retrocochlear), as in acoustic neuroma. Typical of cochlear disease are loss of acuity for pure tones with a parallel impairment of speech discrimination, recruitment, and tone decay. Recruitment is an abnormal loudness of sounds due to cochlear dysfunction, which can cause a paradoxical increase in the perception of louder sounds, sometimes accompanied by sound distortion. Recruitment occurs when there is a reduction in the number of hair cells, which causes a loss of the ability to process fine gradations in sound intensity. A small increase in intensity causes an abnormally large recruitment of nerve fibers responding, and the sound is perceived as abnormally loud. Tone decay measures auditory adaption by assessing the ability to maintain the perception of a pure tone continuously. Tone decay does not occur with cochlear lesions. Retrocochlear lesions tend to cause a loss of speech discrimination out of proportion to the loss for pure tones, no recruitment, and abnormal auditory adaptation by tone decay. There is a debate about the existence of a syndrome of purely cochlear Ménière’s disease.
SNHL may be bilateral and slowly progressive, as with presbycusis or exposure to ototoxic drugs, for example, aminoglycoside antibiotics or loop diuretics. Gabapentin may cause reversible hearing loss in patients with renal insufficiency. Presbycusis is divided into a sensory form, due to degeneration of the organ of Corti and causing high-frequency hearing loss, and a neural form, due to degeneration of the cochlear neurons, causing loss of speech discrimination. SNHL may be unilateral and progressive, as in Ménière’s disease or acoustic neuroma. It may be unilateral and relatively sudden, over hours to days, in vascular disease (e.g., internal auditory artery [IAA] occlusion), viral infection, or autoimmune hearing loss. The syndrome of sudden, unilateral SNHL is due to dysfunction involving the cochlea or CN VIII with variable, often poor, recovery. The pathogenesis is unknown; autoimmunity, viral infection, and vascular disease are suspected etiologies. The IAA may arise from the anterior inferior cerebellar artery or directly from the basilar. Isolated IAA ischemia causes hearing loss often associated with vestibular dysfunction. Ischemia in the AICA distribution produces other CNS manifestations (see Chapter 21).
The cochlear and vestibular nerves run together in a common sheath from the brainstem to their respective end organs, and disorders of the eighth nerve between the cochlea and brainstem may cause hearing loss. Some disease processes affect both divisions peripherally (e.g., labyrinthitis) or centrally (e.g., brainstem neoplasm). In its course across the CPA, the most important disorder to affect both divisions is a neoplasm. Acoustic neuroma (acoustic neurinoma, acoustic schwannoma) is most common, but neurofibroma, meningioma, facial nerve schwannoma, cholesteatoma, epidermoid cyst, and other tumors may arise here as well. Acoustic neuromas usually present with insidious, progressive hearing loss; rarely, sudden deafness may occur, sometimes as the presenting manifestation, presumably because of intratumoral hemorrhage or IAA ischemia. Vague dysequilibrium and imbalance are more common symptoms than true vertigo. Subsequent manifestations depend on the direction of tumor extension. With anterior extension, CNs V and VI are involved. With inferior extension, CNs IX, X, and XI are involved. CN VII is commonly affected in either case, causing all the signs of a far proximal lesion (see Chapter 16). With medial extension, there is mass effect on the brainstem and cerebellum, often leading to ipsilateral ataxia and evidence of increased intracranial pressure. Other conditions of CN VIII that may cause hearing loss include toxins, postinflammatory scarring, as from meningitis, and hereditary conditions. Other conditions that may cause both hearing loss and vertigo include Ménière’s disease, labyrinthitis, viral infection (especially herpes), trauma, meningitis, vascular occlusion (internal auditory or anterior inferior cerebellar), Susac’s syndrome, Cogan’s syndrome, Fabry’s disease, perilymphatic fistula, toxins, and drugs.
Lesions of the central auditory pathways (brainstem and central connections) rarely cause clinical loss of hearing, although detailed audiometric testing and brainstem auditory evoked potentials (BAERs) may show abnormalities. However, midbrain lesions or tumors of the posterior third ventricle—or the aqueduct region with compression of either the medial geniculate bodies or the inferior colliculi—may cause bilateral hearing deficits, presumably because the auditory pathways run close together in this region. Impairment of sound localization contralateral to a temporal lobe lesion has been described. Wernicke’s (auditory receptive) aphasia is characterized by inability to interpret or comprehend spoken words despite normal hearing; it may occur with dominant temporal lobe lesions. Pure word deafness follows bilateral damage to the posterior superior temporal lobes bilaterally, causing an inability to comprehend speech with intact hearing and reading. Other cortical syndromes involving hearing include auditory agnosia, amusia, and disturbances in the temporal analysis of sounds.
Pseudohypacusis refers to hearing loss in the absence of any organic disease, or hearing loss that is exaggerated. It is more common for real hearing loss to be exaggerated in severity than for it to be feigned with entirely normal hearing. The mainstay of diagnosis is inconsistency in the performance on hearing tests and the absence of verifiable abnormalities on objective tests. A diagnosis of pseudohypacusis is easier to establish in children because they are less able to reproduce factitious abnormalities on repeated testing.
Nonorganic hearing loss may be partial or total, unilateral or bilateral. It is often bilateral and total, and the patient makes no attempt to hear what is said or to read the speaker’s lips. In most instances, it is a transient symptom related to acute emotional stress. Psychogenic hearing loss may be associated with other nonorganic symptoms, such as mutism and blindness. When there are also nonorganic motor and sensory disturbances, the hearing loss is usually incomplete and on the same side. In malingering, the deafness is usually unilateral and occurs after trauma in the face of potential secondary gain. Organic posttraumatic hearing loss is typically associated with impairment of vestibular function. Normal labyrinthine responses suggest the claimed hearing loss is either simulated or exaggerated. Inconsistent responses on bedside hearing tests suggest nonorganicity. Discrepancies and inconsistencies on repeated audiometric examinations are typical. The BAER is normal.
Patients simulating bilateral deafness do not behave as a deaf person does. Deaf individuals usually raise their voices during conversation and keep their eyes fixed on the speaker’s face and lips, watching for any gesture that may help understanding. A deaf man eager to hear will automatically turn his best ear toward the speaker. Experienced lip readers have difficulty with sound-alike words; the dissembler may do better than expected because the words are actually heard.
Many tests have been devised for detection of unilateral nonorganic deafness. The diagnosis is best made audiometrically. With some trickery, a stethoscope—with one earpiece occluded—can be put with the occluded earpiece in the good ear and the open earpiece into the bad ear to demonstrate that the “deaf” ear can hear. In the yes-no test, the examiner whispers into the patient’s deaf ear after instructions to “say yes if you hear it and no if you don’t.”
Tinnitus
Tinnitus is spontaneous noise in the ears originating inside the head. There are many types, and the causes are protean. In many cases, no precise etiology can be established. The most common identifiable cause is noise exposure, either acute or chronic. Objective tinnitus refers to noise audible to both the patient and the examiner, as occurs in carotid stenosis. Most tinnitus is subjective tinnitus (tinnitus aurium). It may vary in pitch and intensity and may be continuous or intermittent. It may be described in many ways, such as ringing, buzzing, blowing, whistling, swishing, or roaring. Tinnitus is commonly associated with deafness. It is common in presbycusis and in other types of SNHL and is a fairly constant feature of otosclerosis. It is caused by the abnormal excitation of the auditory apparatus or its afferent pathways, but the exact mechanism is often unclear. Most cases are due to disease of the cochlea or eighth nerve; some are due to CNS disease. Tinnitus is often more noticeable at night when environmental noises are diminished, and it may interfere with sleep. To the patient, tinnitus may be more distressing than the accompanying deafness, and it may cause depression in elderly individuals.
Pulsatile tinnitus is synchronous with the pulse; it is in reality a bruit. Causes include carotid stenosis, arteriovenous malformations, particularly of the dura, glomus tumors, venous hums, and hypertension. Pulsatile tinnitus is fairly common in pseudotumor cerebri, and it occurs occasionally in increased intracranial pressure of other origins. The perilymphatic duct connects the perilymph-filled spaces of the cochlea and an extension of the subarachnoid space in the region of the jugular foramen. Through this channel, pulsations in the subarachnoid space are transmitted to the cochlea. Vascular tinnitus may occasionally be affected by carotid artery compression. Rhythmic tinnitus not synchronous with the pulse may occur with palatal myoclonus (palatal microtremor). Gaze-evoked tinnitus is tinnitus associated with eye movements; it may be due to abnormal communications between the cochlear and vestibular nuclei.
Other causes of tinnitus include cerumen impaction, medications (particularly ototoxic drugs), Ménière’s disease, acoustic neuroma, acute or chronic acoustic trauma, Paget’s disease, anemia, labyrinthitis, and Arnold-Chiari malformation. Muscle spasm, contraction of the tensor tympani, nasopharyngeal sounds, and temporomandibular joint clicking may also simulate tinnitus. Tinnitus may be psychogenic. Bizarre types of tinnitus may occur with pontine and cerebral lesions. Auditory hallucinations may occur in lesions of the temporal lobe; these are frequently epileptic auras. More bizarre hallucinations occur in psychotic and drug-induced states. Tinnitus that is unilateral, pulsatile, fluctuating, or associated with vertigo is more likely to have a serious underlying condition.
THE VESTIBULAR NERVE
The vestibule of the labyrinth connects with five structures that are involved in vestibular function: the utricle, the saccule, and the three semicircular canals. Each of these components lies in the membranous labyrinth, is bathed in endolymph, and contains sensory neuroepithelium. The sensory epithelium consists of cells bearing microvilli, which are referred to as hair cells. The hair cells are the peripheral receptors of the vestibular apparatus. Each hair cell bears a single long kinocilium and an array of shorter stereocilia. The cilia are imbedded in the maculae of the utricle and saccule and in the cupulae of the semicircular canals. Movement of the macula or cupula bends the cilia. Endolymph flows throughout the membranous labyrinth. Changes in endolymph flow in response to external forces or head movement, as well as the effects of gravity and changes in head position, affect neural impulses arising from the areas of sensory epithelium. This is the substrate for vestibular function.
The hair cells function as transducers, converting mechanical deformation of their cilia into receptor potentials. Because of the location of the stereocilia and the kinocilium, each hair cell is structurally polarized. The orientation of each individual hair cell and the arrangement of its microvilli determine its functional response to mechanical stimuli. The cilia contain actin filaments. Bending of the cilia in a specific direction causes the cell to become either depolarized or hyperpolarized. Bending in the opposite direction causes the opposite response. Deformation causes ion fluxes in mechanically sensitive channels in the cilium. Calcium influx due to the mechanical deformation depolarizes the cell and causes release of neurotransmitter. A few channels remain open even in the erect cilia, which produces a moderate level of tonic activity in the vestibular system. The receptors send signals by increasing or decreasing this tonic discharge.
The utricle and saccule constitute the otolith organ and are referred to as the static labyrinth. It is designed to detect gravitational effects and linear acceleration and to monitor head position. The utricle is an oblong sac that extends from the posterosuperior portion of the vestibular part of the membranous labyrinth (Figure 17.3). The saccule is a smaller expansion that lies near the opening of the scala vestibuli of the cochlea. The utriculosaccular duct connects the saccule to the utricle and endolymphatic duct, and the ductus reuniens connects it to the cochlea. The osseous ampulla of a semicircular canal is a bulbous expansion at the point where the canal joins the vestibule, and it is about twice the diameter of the rest of the canal (Figure 17.2). The semicircular ducts are membranous labyrinth tubules that follow the semicircular canals in the same way the cochlear duct follows the spirals of the cochlea. The ampullae of the ducts open off the utricle.
The utricle and saccule each contain a macule. Covering the maculae is a gelatinous layer, the otolithic or statoconial membrane. Embedded in the otolithic membrane are millions of crystals, the otoliths (statoliths, otoconia, or statoconia). The utricle and saccule respond to linear acceleration and to gravity because of the mass of the otoliths. They monitor the position of the head and movement of the head in relation to gravity. In the ampulla of each semicircular canal is a gelatinous structure called a cupula. The canals do not respond to gravity because there are no otoliths in the semicircular canals and because the cupula has the same specific gravity as the endolymph. Instead, movement of the head causes endolymph to flow, which displaces the cupula and stimulates or inhibits the hair cells.
The macula of the utricle lies horizontally in the floor of the utricle, parallel to the skull base. The macula of the saccule lies vertically in the wall of the saccule. Hair cells are oriented in every conceivable direction. Bending of the cilia either depolarizes or hyperpolarizes the cell, depending on the direction of movement. Because of the multidirectional orientation of the hair cells and the geometry of the maculae, head movement in any direction can be detected. Because of its orientation, the macula of the utricle responds maximally to head movement in the sagittal plane, whereas the macula of the saccule responds maximally to head movement in the coronal plane.
The semicircular canals are the kinetic or dynamic labyrinth and are designed to detect angular acceleration or rotation. The crista ampullaris, or ampullary crest, is a focal thickening in the membrane lining the ampullae of the semicircular canals. The cristae are covered with the sensory neuroepithelium of the canals. The tips of the cilia of the hair cells are imbedded in the cupula, which forms a dome-shaped cap over the cristae. When rotation of the head occurs, the endolymph lags behind, tilting the cupula and affecting the neural discharges in the hair cells of the cristae.
The semicircular canals are designed to detect rotation. Their orientation in three perpendicular planes and their oval structure guarantee that head movement in any direction will be detected. The three canals are the horizontal (lateral), vertical (anterior or superior), and posterior (inferior). The labyrinth is imbedded deep in the petrous ridge. In turn, the petrous ridge is set at an angle of about 45 degrees from the sagittal plane of the skull. The canals are named because of their anatomical relationships to the labyrinth and to each other, more so than their relationship to the skull. Different names for the same canal compound the difficulty. The following is a useful approximation for the orientation of the canals. The horizontal canal lies horizontally; the anterior and posterior canals stand vertically. The horizontal canal is convex laterally, and it is also called the lateral canal; it actually slants downward from anterior to posterior at an angle of about 30 degrees. The posterior canal arcs posteriorly parallel to the long axis of the petrous bone, toward the base of the petrous pyramid. The anterior canal lies perpendicular to the long axis of the petrous bone, anterior to the other canals, and toward the apex of the petrous bone. In addition, it extends above the other canals and is also known as the superior canal. If the head is placed forward 30 degrees, the lateral canals are horizontal and the vertical canals are vertical. The canals are maximally stimulated by movement in the plane of their anatomical axis. The horizontal canal best detects rotational head movement in the side-to-side (“no-no”) direction (with the chin tucked to bring the canal fully horizontal). The posterior canal best detects movement in the anteroposterior plane (“yes-yes”), and the anterior canal is oriented to detect lateral tilting movement. The canals on the two sides have been said to form functional pairs. The horizontal canals work together. The anterior canal of one side is approximately parallel to the posterior canal on the opposite side, forming a spatial pair. How closely these angles actually match has been questioned.
The hair cells of both the maculae and the cristae produce a tonic discharge in the vestibular nerve. The discharge rate increases and decreases in response to the bending of the hair cells. Endolymph flow toward the utricle is excitatory. Normally, the two labyrinths are in balance, with symmetric activity in the two vestibular nerves and reciprocal changes induced by head movement. When this balance is disturbed, the clinical signs and symptoms of vestibulopathy follow.
Afferent impulses from the hair cells travel centrally via the peripheral processes of bipolar neurons in the vestibular (Scarpa’s) ganglion in the internal acoustic meatus. Central processes of the vestibular ganglion cells form the vestibular nerve. There are three peripheral divisions of the vestibular nerve, which arise from different portions of the labyrinth. These join to form the vestibular nerve proper (Figure 17.6). The vestibular component of CN VIII joins the cochlear component in a common sheath; the vestibular is the larger of the two. The nerve passes through the IAC in company with the facial nerve and the nervus intermedius. It crosses the cerebellopontine angle and enters the brainstem between the inferior cerebellar peduncle and the olive. The cochlear nerve is slightly lateral and caudal to the vestibular. Within the IAC, CN VIII is lateral and inferior to CN VII. At the pontomedullary junction, CN VIII is slightly lateral and posterior to CN VII.
Entering vestibular fibers pass between the inferior cerebellar peduncle and the spinal tract of CN V. They divide into ascending and descending branches that end primarily in the four vestibular nuclei: lateral, medial, superior, and inferior (Figure 17.10). The vestibular nuclei lie in the rostral medulla and caudal pons. Some fibers form the vestibulocerebellar tract and pass directly to the cerebellum, without synapsing in the vestibular nuclei, in the juxtarestiform body. The medial (Schwalbe’s) vestibular nucleus is the largest subdivision of the vestibular nuclear complex, extending from the medulla into the pons. It lies in the floor of the fourth ventricle, beneath the striae medullares. The inferior (descending, spinal, Roller) nucleus lies lateral to the medial, between the medial nucleus and inferior cerebellar peduncle, and descends further inferiorly to reach lower medullary levels. The lateral (Deiters’) and superior (Bechterew’s) subnuclei are smaller than the medial and inferior. The lateral nucleus is lateral to the rostral end of the medial nucleus. The superior nucleus extends higher into the pons than other subdivisions, forming a cap on the nuclear complex. Vestibular afferents to the superior and medial subnuclei arise predominantly from the semicircular canals and less so from the otolith organs. Afferents to the lateral and inferior subnuclei arise predominantly from the otolith organs and less so from the semicircular canals. The vestibular nuclei also receive afferent cerebellovestibular fibers through the juxtarestiform body (part of the inferior cerebellar peduncle), primarily from the flocculonodular lobe, as well as afferents from the spinal cord and reticular formation.
FIGURE 17.10 The vestibular pathway.
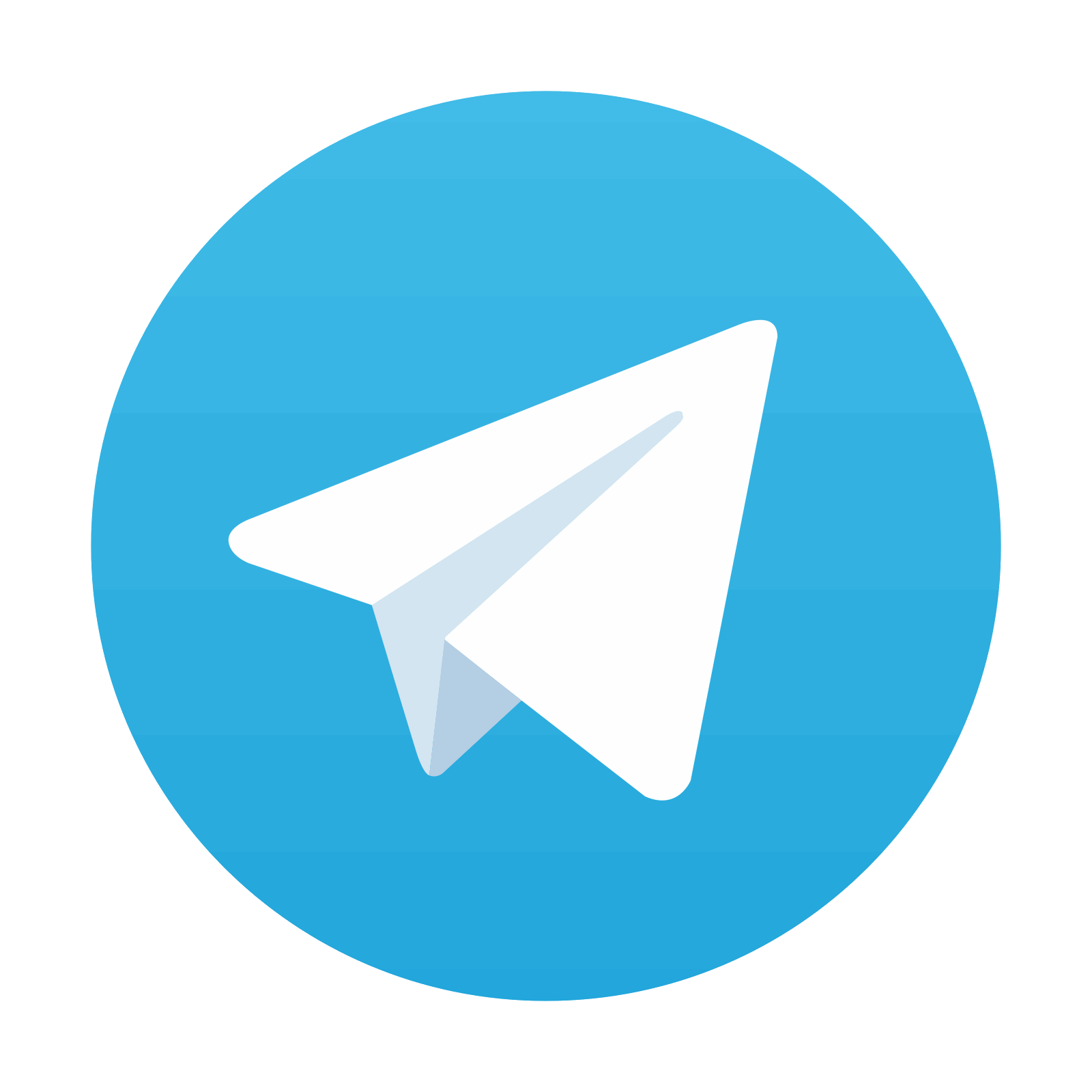
Stay updated, free articles. Join our Telegram channel

Full access? Get Clinical Tree
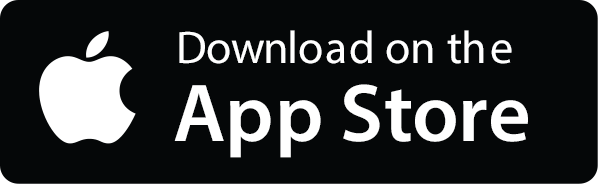
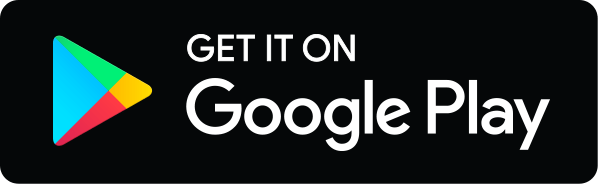