The Basal Ganglia
The Basal Ganglia Consist of Several Interconnected Nuclei
The Motor Circuit Plays a Role in Multiple Aspects of Movement
Dopaminergic and Cholinergic Inputs to the Striatum Are Implicated in Reinforcement Motor Learning
Abnormalities in the Basal Ganglia Motor Circuit Result in a Wide Spectrum of Movement Disorders
A Deficiency of Dopamine in the Basal Ganglia Leads to Parkinsonism
Reduced and Abnormally Patterned Basal Ganglia Output Results in Hyperkinetic Disorders
THE TRADITIONAL VIEW THAT THE BASAL ganglia play a role in movement stems largely from the fact that diseases of the basal ganglia, such as Parkinson and Huntington disease, are associated with prominent disturbances of movement and from the earlier belief that basal ganglia neurons send their output exclusively to the motor cortex by way of the thalamus. However, we now know that the basal ganglia also project to nonmotor areas of the cerebral cortex, providing a mechanism whereby they may participate in a wide variety of nonmotor functions, and that diseases of the basal ganglia are associated with complex behavioral and neuropsychiatric disturbances.
In this chapter we first describe the individual nuclei of the basal ganglia anatomically and then discuss their function in the context of the larger networks in which they participate. The delineation of brain circuits into which the basal ganglia are incorporated has enabled researchers to better understand the pathophysiology of some of the major diseases affecting basal ganglia functions. These disease states are described at the end of the chapter.
The Basal Ganglia Consist of Several Interconnected Nuclei
The basal ganglia comprise four principal structures: the striatum, globus pallidus, substantia nigra, and subthalamic nucleus (Figure 43–1).
Figure 43-1 The basal ganglia and surrounding structures. The nuclei of the basal ganglia are identified on right in this coronal section. (Adapted, with permission, from Nieuwenhuys, Voogd, and van Huijzen 1981.)
The striatum is separated by the internal capsule into the caudate nucleus and the putamen. The striatum is the major input structure of the basal ganglia, receiving prominent projections from the cerebral cortex, brain stem, and thalamus. The globus pallidus consists of two separate nuclei, the external and internal segments, each with different connectivity and functions. The internal segment is one of the major output structures of the basal ganglia, whereas the external segment is part of their intrinsic circuitry.
The substantia nigra includes two separate nuclei, the pars compacta and pars reticulata. Along with portions of the ventral tegmental area and other midbrain areas, the pars compacta, or mediodorsal portion of the substantia nigra, contains dopaminergic cells that project heavily to the striatum and to the other nuclei of the basal ganglia. The pars reticulata, or ventrolateral portion of the substantia nigra, is the other major output nucleus of the basal ganglia. In fact, the pars reticulata of the substantia nigra and the internal segment of the globus pallidus can be viewed as a single output structure divided by the internal capsule.
The fourth principal structure of the basal ganglia, the subthalamic nucleus, is a small nucleus situated between the thalamus and the substantia nigra. This nucleus receives projections from the external segment of the globus pallidus, the cerebral cortex, thalamus, and brain stem, and sends output to both segments of the globus pallidus and to the substantia nigra pars reticulata. The cortical inputs to the subthalamic nucleus and the related subthalamopallidal projections are referred to as the hyperdirect pathway (Figure 43–2).
Figure 43-2 The basal ganglia–thalamocortical circuitry. The circuitry of the basal ganglia includes the striatum (here represented by one of its components, the putamen), the external and internal segments of the globus pallidus (GPe and GPi, respectively), the substantia nigra pars reticulata (not shown) and pars compacta (SNc), and the subthalamic nucleus (STN). Cortical input enters the striatum and subthalamic nucleus. Basal ganglia output is conveyed to several thalamic nuclei (the centromedian and parafascicular nuclei and the ventral anterior and ventral lateral nuclei) and the pedunculopontine nucleus. Excitatory connections are shown in red, inhibitory pathways in gray. The dopaminergic SNc projection to the striatum regulates corticostriatal transmission along direct and indirect pathways.
The striatum, the main input nucleus of the basal ganglia, projects to the two basal ganglia output nuclei, the internal pallidal segment and the substantia nigra pars reticulata. The axons from the striatum follow two different pathways: a direct monosynaptic connection, and an indirect polysynaptic pathway that passes first to the external pallidal segment and from there to both output nuclei, either directly or via the intercalated subthalamic nucleus.
The output nuclei project to specific thalamic and brain stem areas. Projections to the thalamus are directed to the ventral anterior, the ventrolateral, and the intralaminar nuclei. Thalamic projections to the frontal lobe then transmit the output of the basal ganglia to the same areas of frontal cortex that provide input to the basal ganglia. In addition, descending pallidal and nigral projections to the brain stem, such as those to the pedunculopontine nucleus and superior colliculus, provide pathways by which the basal ganglia may directly influence brain stem and spinal motor circuits, especially those related to gait and balance. The brain stem nuclei may integrate basal ganglia inputs with cerebellar inputs. The pedunculopontine nucleus is part of several feedback circuits through its projections back to the basal ganglia and thalamus. Output of the substantia nigra pars reticulata is also directed to the superior colliculus, which is involved in the control of head and eye movements.
In the striatum the most common neuronal cell type is the GABAergic (γ-aminobutyric acid) medium spiny neuron. These cells are so named because of the abundance of spines on their dendrites. They receive inputs from the cerebral cortex and thalamus as well as from several classes of local interneurons in the striatum, including large cholinergic interneurons and smaller GABAergic interneurons.
The activities of medium spiny neurons are modulated by other neurotransmitters, specifically inputs from dopaminergic neurons in the substantia nigra pars compacta and the ventral tegmental area. Some of the dopaminergic fibers terminate on the necks of dendritic spines of medium spiny neurons, where they are in a position to influence corticostriatal transmission (Figure 43–3). Dopamine released from terminals close to the dendritic spines may have similar effects through spillover and diffusion of the neurotransmitter.
Figure 43-3 The medium spiny neurons in the striatum have extrinsic and intrinsic inputs. Glutamatergic inputs from the cerebral cortex and dopaminergic inputs from the substantia nigra pars compacta terminate on dendritic spines of medium spiny neurons. The reward-related dopaminergic inputs are thought to modulate the strength of cortical inputs and to play a role in synaptic changes and reinforcement learning in the striatum. Glutamatergic inputs from the thalamus end on the spines and shafts of dendrites of medium spiny neurons. Medium spiny neurons also receive cholinergic and GABAergic input from interneurons in the striatum.
The cytoarchitecture of the other basal ganglia nuclei is distinctly different from that of the striatum. Both segments of the globus pallidus consist of large GABAergic neurons that receive input from the striatum. The substantia nigra pars reticulata is histologically similar to the internal pallidal segment, containing GABAergic neurons that interdigitate with the more dorsal dopaminergic cells of the substantia nigra pars compacta. The subthalamic nucleus is a densely packed structure whose projection neurons, unlike those in the other basal ganglia nuclei, are glutamatergic.
A Family of Cortico–Basal Ganglia–Thalamocortical Circuits Subserves Skeletomotor, Oculomotor, Associative, and Limbic Functions
Areas of the cerebral cortex project in a highly topographic manner onto the striatum.
The topographic termination pattern establishes functional domains that are replicated throughout the basal ganglia–thalamocortical circuits by virtue of highly topographic projections at each synaptic relay. The different pathways that pass through the basal ganglia are named after the presumed functions of the regions of the frontal cortex from which they originate: the skeletomotor, oculomotor, pre-frontal (associative), and limbic circuits. The frontal lobe origins of these circuits in the cerebral cortex are shown in Figure 43–4 and the synaptic relays are depicted in Figure 43–5.
Figure 43-4 Four basal ganglia–thalamocortical circuits originate in four functionally distinct areas of frontal cortex. (ACA, anterior cingulate area; DLPFC, dorsolateral pre-frontal cortex; FEF, frontal eye field; LOFC, lateral orbitofrontal cortex; M1, primary motor cortex; MOFC, medial orbitofrontal cortex; PM, premotor cortex; SEF, supplementary eye field; SMA, supplementary motor area.) (Adapted, with permission, from Alexander and Crutcher 1990.)
Figure 43-5 Global anatomy of cortico–basal ganglia–thalamocortical circuits. (ACA, anterior cingulate area; CMA, cingulate motor area; DLPFC, dorsolateral pre-frontal cortex; FEF, frontal eye field; GPi, internal segment of the globus pallidus; LOFC, lateral orbitofrontal cortex; M1, primary motor cortex; MDpl, mediodorsal nucleus of thalamus, lateral part; MOFC, medial orbitofrontal cortex; PMC, premotor cortex; SEF, supplementary eye field; SMA, supplementary motor area; SNr, substantia nigra pars reticulata; VAmc, ventral anterior nucleus of thalamus, magnocellular part; VApc, ventral anterior nucleus of thalamus, parvocellular part; VLcr, ventrolateral nucleus of thalamus, caudal part, rostral division; VLm, ventrolateral nucleus of thalamus, medial part; VLo, ventrolateral nucleus of thalamus, pars oralis.) (Adapted, with permission, from Wichmann and DeLong 2006.)
Additional projections from the parietal, temporal, and occipital lobes that are reciprocally interconnected with the frontal areas converge onto the same areas in the striatum. Importantly, however, although each circuit receives both pre- and postcentral cortical inputs, output of the different circuits terminates only in the frontal lobe areas of their respective origin.
Ascending output in each functional pathway is projected in a somatotopical manner to the thalamus and from there to the frontal cortical area from which the circuit originated, thus partially closing a system of cortico-subcortical loops. The subcortical segregation of the functionally distinct circuits may allow different aspects of behavior to be processed in parallel.
The Cortico–Basal Ganglia–Thalamocortical Motor Circuit Originates and Terminates in Cortical Areas Related to Movement
Most of our knowledge about the anatomy and physiological functions of the basal ganglia–thalamocortical circuits comes from studies of the motor circuit. This circuit has attracted the attention of researchers because pathology within its anatomical elements has been implicated in several major disorders of movement.
The motor circuit originates in the pre- and postcentral sensorimotor cortical fields, which project to the putamen in a somatotopical manner. This arrangement has been demonstrated not only with anatomical methods but also with electrophysiological recordings of neuronal activity while animals were subjected to passive movements or carried out active movements of individual body parts. These studies showed that neurons responding to leg movements are found in a dorsolateral zone of the putamen, neurons responding to orofacial movements are located ventromedially, and neurons responding to arm movement are found in a zone between the leg and orofacial areas.
Neurons in the putamen project to the caudoventral portions of both segments of the pallidum and to the lateral portions of the substantia nigra pars reticulata. In turn, the motor portions of the internal pallidal segment and the substantia nigra pars reticulata project to specific motor-related areas of the ventral lateral, ventral anterior, and centromedian nucleus of the thalamus. The motor circuit is then closed by projections from the ventral lateral and ventral anterior nuclei to the motor cortex, supplementary motor area, and premotor cortex. The centromedian nucleus, one of the intralaminar nuclei of the thalamus, projects largely to the putamen as part of a subcortical feedback loop.
The larger motor circuit consists of segregated subcircuits, each centered on an individual precentral motor field. These subcircuits are believed to be responsible for different aspects of motor processing, such as motor planning, coordination of sequences of movement, or movement execution. Evidence for the subcircuit organization comes from anatomical studies. Several stages of the basal ganglia circuitry in the same animal have been traced by Peter Strick and his colleagues using small intracerebral injections of herpes and rabies viruses. Taken up by neurons and transported transsynaptically, the virus particles can be stained in anatomical slices. Using this technique at different time points after the injection, one may visualize circuit elements that lie two or more synapses away from the injection site.
Separate injections of the primary motor cortex, supplementary motor area, and lateral premotor area produces retrograde labeling of separate populations of neurons in specific areas of the ventral lateral nucleus in the thalamus and separate populations of neurons in the internal pallidal segment, demonstrating that the separate cortical domains remain segregated throughout the basal ganglia networks. Segregated anterograde transsynaptic transport of input from cortical areas to the striatum and pallidum has likewise been shown, providing further support for the segregated circuit concept.
Because axons of cortical neurons terminate on a far smaller number of striatal neurons, there is considerable convergence of cortical information in the striatum. Similarly, the number of neurons in the pallidum and substantia nigra is smaller than that in the striatum, allowing further convergence along the direct and indirect pathways. However, given the somatotopic arrangement of striatopallidal and pallido-subthalamic projections, it appears that convergence occurs largely within rather than between the different basal ganglia–thalamocortical circuits and subcircuits.
The Motor Circuit Plays a Role in Multiple Aspects of Movement
The motor circuit has been examined in experimental studies in which portions of the basal ganglia were activated or inactivated, in studies using extracellular electrophysiological recordings of the activity of single neurons, as well as imaging and behavioral studies. Based on these investigations the motor circuit has been implicated in a wide range of motor behaviors including action selection, preparation for movement, movement execution, sequencing of movement, self-initiated or remembered movements, the control of movement parameters, and reinforcement learning.
The idea that the basal ganglia have a role in action selection and the initiation of movement was first suggested by early clinical observations in patients with movement disorders. The concept that the basal ganglia play a role in action selection, in the broadest sense, implies that they also participate in the acquisition of behaviors that lead to a reward or reinforcement and the avoidance of acts that lead to punishment or adverse outcomes. Reward may be simply the delivery of a pleasurable stimulus such as food, but may also involve the successful reaching of a goal or intended action. By modulating the strength of specific corticostriatal synapses, dopamine is widely implicated in this action selection function, as described below.
The general concept that the basal ganglia play a role in the acquisition and selection of beneficial behaviors later evolved into the idea that the basal ganglia act to focus specific movements through interactions between the direct (permissive) and indirect (inhibitory) pathways at the level of the internal pallidal segment, somewhat equivalent to the center-surround inhibition in a sensory system. This “focusing model” stems from the observation that the basal ganglia provide sustained inhibitory output to thalamocortical neurons. According to the model, cortical phasic activation of striatal neurons that contribute to the direct pathway transiently suppresses the high spontaneous discharge rate of movement-related neurons in the output nuclei of the basal ganglia. This in turn removes inhibition from specific thalamocortical neurons and allows cortical areas to become active, thus facilitating the selected movement. In contrast, phasic activation of striatal neurons that contribute to the indirect pathway, or of cortical neurons that project to the subthalamic nucleus, transiently increases inhibition of thalamocortical neurons and thereby inhibits movement. If the direct pathway is activated in anticipation of intended movements, and the indirect pathway is activated simultaneously to broadly inhibit pallidal inhibitory output, the combination facilitates intended movements and suppresses competing ones.
Although this focusing model is attractive, there are several strong arguments against it. For instance, the hypothesis would require that axons in the indirect and hyperdirect pathways diffusely target large areas of the pallidum in order to prevent unwanted movements, whereas the direct pathway would act on small areas of the internal pallidal segment to selectively facilitate the selected movement. Neither of these anatomical prerequisites is supported by anatomic studies, which indicate instead that inputs of the indirect pathway to the internal pallidal segment from the subthalamic nucleus are highly topographic. Furthermore, pallidal activation during movement initiation is generally considered to occur too late to play a significant role in the selection of movement.
Evidence that the motor circuit has a role in the preparation of movement comes from single-neuron recordings in monkeys performing delayed-response motor tasks. The animals were required to move an arm to a specified target after a delay period. In such studies the firing frequency of neurons in frontal and pre-frontal cortical areas changed after animals were presented with a visual cue that specified the desired direction of movement (see Chapter 38). Changes similar to those found in the cortex are also found in the motor portions of the putamen, the internal segment of the globus pallidus, and the substantia nigra pars reticulata. These changes in activity occur while the animal is preparing the movement but not during the execution of the movement itself. Such changes are interpreted as involvement in a preparatory stage of motor control referred to as motor set.
Other basal ganglia neurons change their firing frequency phasically in relation to the onset of a movement, suggesting that they may be concerned with movement execution. As mentioned above, these changes in neural activity in a variety of stimulus-triggered movement tasks occur well after movement-related activities in the cerebral cortex or cerebellum, indicating that the basal ganglia do not participate in the initiation of such movements. This conclusion is reinforced by the results of a study with primates trained on a simple reaction time task: Pallidal lesions involving the motor circuit did not alter the reaction time between a cue and the movement triggered by that cue.
Changes in the activity of movement-related neurons in the internal pallidal segment correlate with the amplitude and velocity of arm movement, suggesting a role in the scaling of movement. In monkeys the activity of 30% to 50% of all movement-related neurons in the supplementary motor area, motor cortex, putamen, and pallidum is correlated with the direction of limb movement, but not with the activity of individual muscles. This suggests a role in the higher-level aspects of movement. The finding that individual neurons in the basal ganglia tend to be concerned with either the preparation or the execution of motor action suggests that these functions are mediated by separate subcircuits in the motor circuit.
Positron emission tomography and functional magnetic resonance imaging of humans have demonstrated that during simple finger or arm movements the peak activation of the basal ganglia occurs in the post-commissural putamen, and that changes in fundamental kinematic parameters, such as movement velocity, correlates with activity in the posteroventral pallidum, an area that has been identified as part of the motor territory of the basal ganglia in nonhuman primates (Figure 43–6). By contrast, in cognitively demanding tasks—for example, tasks that require subjects to generate novel sequences of movement or to imagine hand movements—anterior portions of the striatum (caudate nucleus and putamen rostral to the anterior commissure) are activated, along with the pre-frontal cortex and the anterior cingulate area.
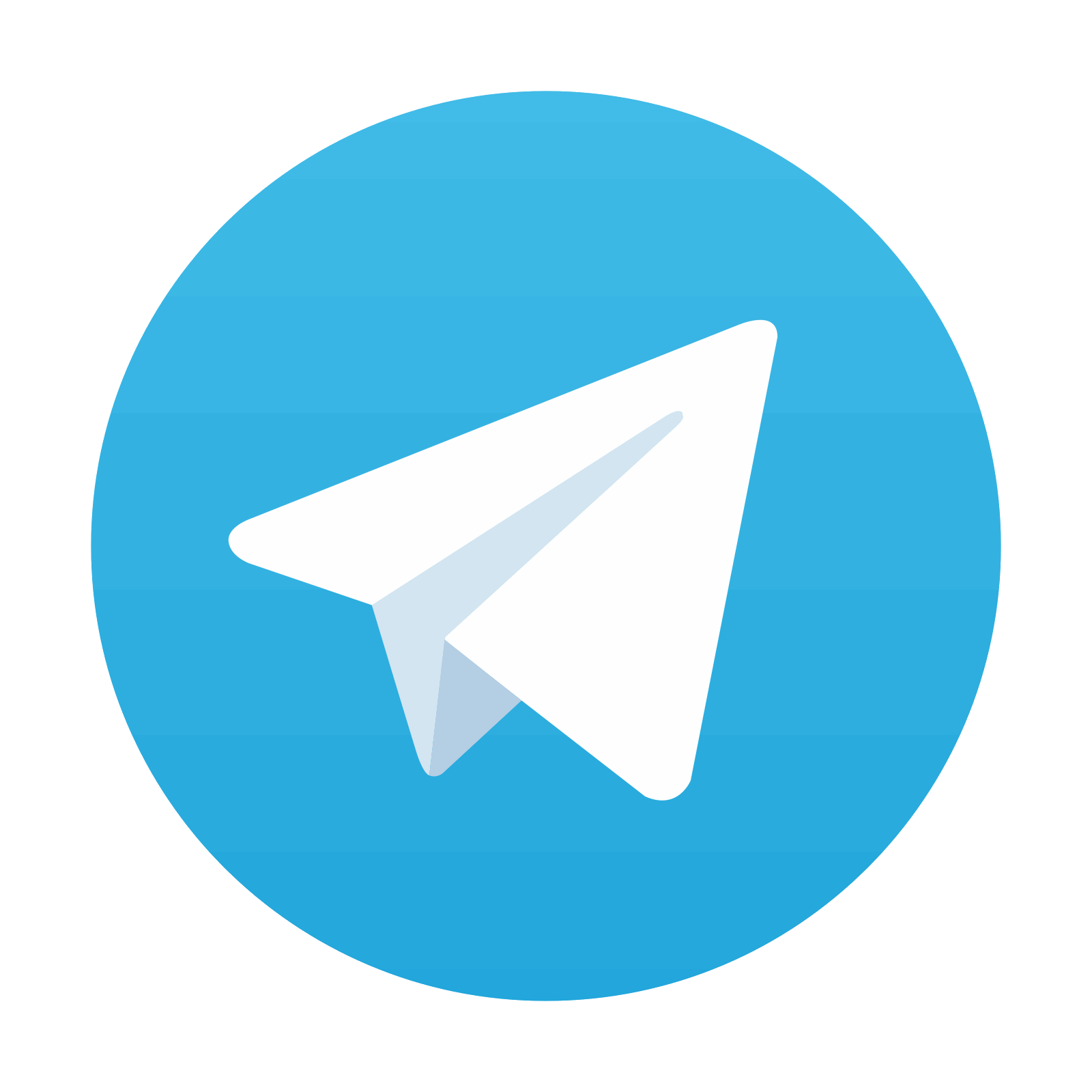
Stay updated, free articles. Join our Telegram channel

Full access? Get Clinical Tree
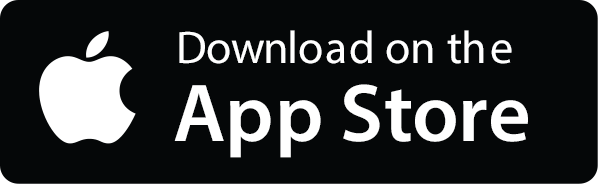
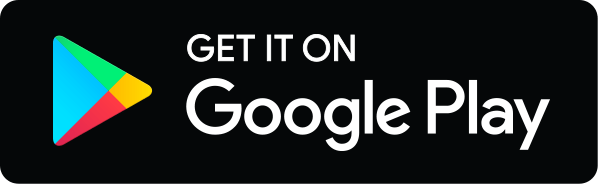