6 The Biology and Biomechanics of the Spinal Degenerative Cascade Kern Singh, Daniel K. Park and Frank M. Phillips More than 25 years ago, Kirkaldy-Willis et al presented the concept of a cascade of spinal motion segment degeneration invoking progressive wear of the intervertebral disc (IVD) and facet joints (Fig. 6.1).1 They emphasized the interdependence of the disc and facet joints for normal spinal function and described how derangement or injury to either of these articulations leads to abnormal forces and impairment of the other, the so-called tripod effect. They further described the morphologic features of spinal degeneration and postulated how these might be associated with various clinical syndromes. Although insightful, this algorithm was quite mechanistic and, in keeping with the times, highlighted biomechanical disturbances associated with degeneration of the motion segment. Over the decades since, we have come to appreciate that spinal degeneration involves a complex interplay of biologic and bio-mechanical events that are predisposed by genetic factors and modulated by environmental influences. Fig. 6.1 Kirkaldy-Willis schematic demonstrating a proposed mechanism for disc and facet degeneration. (From Kirkaldy-Willis WH, Wedge JH, Yong-Hing K, Reilly J. Pathology and pathogenesis of lumbar spondylosis and stenosis. Spine 1978;3:319–328. Reprinted with permission.) Degeneration of the spine is an inevitable consequence of aging. Miller et al reported an increase in disc degeneration from 16% at age 20 to ~98% at age 70 years based on macroscopic disc degeneration grades of 600 autopsy specimens. Interestingly, the authors noted that lumbar disc degeneration was already present in 11- to 19-year-old males and 10 years later in females.2 Although spinal degeneration is inevitable with aging and invariably seen on MRI studies, it is typically asymptomatic.3 Kirkaldy-Willis et al postulated that injury or repetitive strain to the facet joint is a cardinal event in the spinal degenerative sequence.1 More recently, the IVD has received considerable attention as the source of initial spinal motion segment dysfunction. Butler et al suggested that disc degeneration likely predates facet arthrosis based on a CT and MRI study.4 The authors noted that in 68 patients (330 discs/390 facet joints) there were 144 degenerated discs and 41 levels with facet osteoarthritis. Disc degeneration without facet osteoarthritis was found at 108 levels, whereas all but one of 41 levels with facet degeneration also had disc degeneration.4 The widespread acceptance that spinal pain is thought to originate from the IVD is evidenced by the host of diagnostics (including discography) and therapeutic interventions directed toward the disc. Most treatments for painful discs, however, have met with inconsistent clinical outcomes,5 probably reflecting a relatively unsophisticated approach to understanding spinal pain. Recent data supporting the idea of facet (zygapophyseal) joint mediated pain have come from studies of patients sustaining cervical whiplash injuries.6,7 Lord et al evaluated cervical zygapophyseal joint pain after whiplash in a diagnostic double-blind study using placebo-controlled local anesthetic blocks. Sixty-eight patients with a predominant complaint of neck pain and headaches after a whiplash injury were evaluated. The authors noted that among patients with dominant headache, comparative blocks revealed that the prevalence of C2-C3 zygapophyseal joint pain was 50%. Overall, the prevalence of cervical zygapophyseal joint pain was 60%.6,7 These studies support the complex interplay of the IVD and facet joints in health and disease of the spine. Our understanding of spinal degeneration has advanced as we have appreciated that the degenerative cascade involves interplay of both biologic and biomechanical factors. Biochemical events are important in the pathogenesis of the degenerative process as well as in the pain-signaling pathways responsible for the clinical features of the condition. As we better appreciate the biologic aspects of spinal degeneration, less invasive, nonablative treatments designed to reverse these biologic processes and restore the disc and facet functioning may become a reality. IVD degeneration is a major cause of musculoskeletal disability in humans.8–10 Degeneration has been linked to low back pain; however, the exact relationship between the two remains uncertain.11,12 The macroscopic features characterizing disc degeneration include the formation of tears within the anulus fibrosus (AF), and progressive fraying and dehydration of the nucleus pulposus (NP) with eventual loss of the anular-nuclear distinction.8,9,13 These pathologic alterations result in substantial changes in the functioning of the disc. Unquestionably, disc degeneration is a multifactorial process influenced by genetics, lifestyle conditions (including obesity, occupation, and smoking), biomechanical loading, and biochemical event.14,15 The disc is capable of converting axial spinal loads into tensile hoop stresses in the outer AF, while allowing motion of the vertebral segment. This behavior of the IVD is dependent on the distinct biomechanical properties of the NP and AF. The proteoglycan (PG)-rich NP acts as an internal semifluid mass, whereas the collagen-rich AF acts as a laminar fibrous container.16 The hydrostatic properties of the disc arise from its high water content, which allows it to support such large loads.17,18 The NP in a young adult acts as a viscid fluid under applied pressure but also exhibits considerable elastic rebound, assuming its original physical state upon release.19 Whereas a major function of the NP is to resist and redistribute compressive forces within the spine, the major function of the AF is to withstand tension. The unique combination of biochemical and biomechanical properties of the AF and NP allows the IVD to absorb and disperse the normal loading forces experienced by the spine.19,20 When one of these two units, either the AF or NP, is compromised, degenerative changes ensue because of the alteration in mechanical force distribution across the functional spinal unit. Horst and Brinckmann found that the stress distribution across the IVD and vertebral endplate depends on the degree of disc degeneration.21 Under pure compressive and eccentric-compressive loading, the healthy lumbar IVD demonstrated a uniform stress distribution across the entire endplate area. Severely degenerated discs demonstrated the same uniform shape of stress distribution under compressive loading but a nonuniform stress distribution when loaded eccentrically. The asymmetry of the stress distribution in degenerated discs was found to increase with both angle of inclination and degree of degeneration. The asymmetric stress distribution was presumed to occur because of the relatively solid nature of the degenerated disc and its inability to conform to the eccentric loads. These results have been further supported by more recent studies as well.22,23 With advancing degeneration, it appears that the proportion of load transmission shifts to the posterior elements. Yang and King indirectly measured facet forces by using an intervertebral load cell to measure the load transferred through the disc.11 The model predicted a significant increase in facet load for segments with degenerated discs. The increase was more prominent as the eccentricity of the applied compressive load increased posteriorly. This biomechanical sequence of disc degeneration leading to posterior element load bearing may, in fact, be what is observed clinically in that disc degeneration typically precedes facet arthrosis.4 Clinically, a common observation is that disc degeneration creates instability of the lumbar spine and, therefore, increases range of motion.24 The interplay between the IVD geometric and material properties as well as facet joint competence is important in defining the stability of the involved motion segment.25 Biomechanical studies suggest that changes in stability with disc degeneration are quite complex. The kinematic behavior of a simulated degenerative model under compressive and shear loading was studied by Frei et al.26 The authors found greater axial translations under compression in the degenerated model (nucleotomy) compared with the normal disc. In anterior shear, the anterior translation was smaller in the degenerated specimens versus the normal specimens. Anterior shear was accompanied by a significant increase in coupled flexion rotation in the degenerative model, which could explain the counterintuitive decrease in translation. This was attributed to an increase in facet load in degenerated specimens during anterior shear loading. In addition, Fujiwara et al27 found that in vitro cadaveric specimens had segmental motion changes, which were much greater in axial rotation compared with lateral bending, flexion, and extension. Ochia et al28 also found an increase in torsional and flexion and extension movements in vivo. These kinematic studies ultimately can be related clinically to the concept that excessive motion beyond normal soft tissue or bony constraints causes compression or stretching of the neural elements, or deformation of the soft tissue.29 These instabilities can cause abnormal motion and contact forces, as well as accelerate facet degeneration and osteoarthritis. Eventually as pointed out by Kirkaldy-Willis, with advancing degeneration the motion segment ultimately becomes less mobile, although the remaining motion may certainly be painful.24 As the disc becomes less mobile, this may, in turn, decrease the intrinsic disc strength and may decrease nutrition to the disc.30 Besides spinal instability creating degenerative disc disease, another competing biomechanical cause for disc degeneration is the “wear and tear” hypothesis. In this mechanism, a series of minor mechanical traumas to the disc accumulate, eventually creating disc weakening. This weakening results in further injury, and a vicious cycle ensues ultimately leading to disc degeneration.31,32 If this model was the main reason for disc degeneration, a logical assumption would be that those who experienced heavy physical disc loading, particularly laborers, would have an elevated risk of disc degeneration. Most studies have shown an association between heavy physical loading and disc degeneration.33–42 A study by Friberg and Hirsch, however, did not find an association between occupational and spine degeneration radiographically.43 Other studies as well have not demonstrated a clear association.33,44–48 Whatever the biomechanical etiology for disc degeneration, researchers have attempted to define a relationship between biomechanical IVD alterations and symptomatology. More recently, disc dysfunction associated with axial back pain giving rise to so-called internal disc derangement has received considerable attention. MRI is a valuable diagnostic tool in assessing for internal disc derangement.49 MRI allows determination of the proton density of the disc indicative of the state of hydration and can also identify the presence of annular tears. Aprill and Bogduk described the MRI high-intensity zone (HIZ), which they believe to be representative of an annular tear extending to the periphery of the disc.50 The HIZ can be seen on spin echo T2-weighted (T2W) MRI scans as a high-intensity signal located in the substance of the posterior AF (Figs. 6.2, 6.3). The HIZ has been suggested as, but by no means confirmed to be, associated with discogenic axial back pain.51,52 Modic et al described adjacent bony endplate changes that occur with degeneration of the IVD.53,54 Type 1 changes (decreased signal intensity on T1-weighted [T1W] spin-echo MRI scans and increased signal intensity on T2W MRI scans) were identified in 20 patients, type 2 changes (increased signal intensity on T1W MRI scans and isointense or slightly increased signal intensity on T2W MRI scans) in 77 patients, and type 3 changes (decreased signal on T1W and T2W MRI scans) in 16 patients. Histopathologic sections in cases of type 1 change demonstrated disruption and fissuring of the endplates and vascularized fibrous tissue, type 2 changes demonstrated yellow marrow replacement, and type 3 changes demonstrated loss of marrow and advanced bony sclerosis. These signal intensity changes appear to reflect a spectrum of vertebral body marrow changes associated with degenerative disc disease.53 As disc degeneration progresses, the resulting abnormal motion or instability is believed to be a cause of spinal pain, likely related to stretching of soft tissues and stimulation of free nerve endings.24,25,55 Although a precise understanding of what constitutes spinal “instability” remains elusive, numerous treatments aimed at reducing painful spinal motion have been described. Physical therapy using stabilizing exercises has been proposed as an attempt to restabilize the “unstable” spine.56,57 This approach may be more effective when painful segmental motion is the consequence of injury and dysfunction of the paraspinal muscle system that renders the motion segment biomechanically vulnerable in the neutral zone. The clinical diagnosis is based on the report of pain and the observation of movement dysfunction within the neutral zone and the associated finding of excessive intervertebral motion at the symptomatic level. Fig. 6.2 Biology of disc disruption. ALL, anterior longitudinal ligament; PLL, posterior longitudinal ligament. Fig. 6.3 A sagittal magnetic resonance imaging scan demonstrating a degenerated, collapsed L5-S1 disc space as evidenced by the loss of disc height and decreased T2-weighted signal. The white arrow points to an area along the posterior anulus exhibiting an increased T2-weighted signal representative of a high intensity zone. Other reported techniques for restabilizing the spine include intradiscal therapies such as intradiscal electrothermal therapy (IDET), which purportedly attempt to stiffen the motion segment by altering collagen fibers within the IVD.58,59 Histologic studies of IVD material after IDET have reported histologic changes of collagen fibril denaturation in the posterior AF.60 Another restabilization approach involves the use of posteriorly implanted “dynamic devices” that limit, but do not eliminate motion. These devices have been extensively implanted in patients in Europe for select cases of mechanical back pain with “instability.” Total disc replacement, which provides axial stability while allowing for motion, is being increasingly used for the treatment of painful disc degeneration. Facet joints are true synovial articulations and undergo degenerative changes similar to those of osteoarthritis seen in other synovial joints.11,61 The facet joints are one of the primary stabilizing structures of the spinal motion segment.62,63 As the degenerative cascade progresses and anterior column support is lost, the facet joints bear more weight and the fulcrum moves dorsally to balance the motion segment.64 With progressive spinal degeneration, the load-bearing patterns of the facet joints are altered.27 Fujiwara et al performed a biomechanical and imaging study of human cadaveric spinal motion segments to determine the effect of disc degeneration and facet joint osteoarthritis on the segmental flexibility of the lumbar spine.27 The authors noted that axial rotation was most affected by disc degeneration. Facet cartilage degeneration, especially thinning of the cartilage, causes capsular ligament laxity, which may allow abnormal motion or hypermobility of the facet joint. The authors noted a significant linear correlation between facet cartilage thinning and disc degeneration in the male cadavers. Cartilage degeneration appeared to further increase the segmental movements already present in the hypermobile, degenerated disc. Facetectomy studies have been performed by Sullivan et al in the lumbar spine of immature white rabbits to create a facet-mediated degenerative model.65 The researchers resected the inferior articular process on one side at a selected vertebral level and on the opposite side at the adjacent level. The disc height was decreased at the surgical level in 50% of the discs at 6 months and 74% at 12 months. At 9 to 12 months, the discs showed thinning of the posterior AF, circumferential slits in the peripheral AF, and an increased area as well as decreased organization of the NP. The facet joints opposite the facetectomy began to show degeneration at 6 months. The authors concluded that the facet joint protects the IVD from rotational stresses. Unquestionably, the facet joint complex has an important role in stabilizing the segmental spinal unit.27,32,62,66,67 As disc disease progresses, increased stress is applied posteriorly accelerating facet osteoarthrosis. The resultant facet joint osteoarthrosis is likely to change the segmental spinal motion, altering the mechanical forces experienced by the IVD. Cells residing in both the AF and NP actively regulate the homeostasis of IVD tissue. These cells maintain a balance between anabolism and catabolism by modulating a variety of substances including cytokines, enzymes, enzyme inhibitors, and growth factors in a paracrine and/or autocrine fashion.13,68–70 Anabolic regulators include polypeptide growth factors, such as insulin-like growth factor (IGF), transforming growth factor-β (TGF-β), and bone morphogenetic proteins (BMPs). Other small molecules such as the synthetic peptide of link proteins have also been reported to be regulators of matrix synthesis.13,70,71 The catabolic process is also mediated by various enzymes, such as matrix metalloproteinases, aggrecanases, and cytokines.72,73 The degeneration of an IVD results from an imbalance between the anabolic and catabolic processes, or the loss of steady-state metabolism that is maintained in the normal disc. This delicate homeostatic balance affects the biomechanics of the IVD as well. A healthy IVD is populated by at least two morphologically distinct cell types.74–79 Most cells are small and round, similar to chondrocytes. The second cell type is thought to be a remnant of the primitive notochord and has a vacuolated appearance and prominent intracellular glycogen deposits. Surrounding these cells is a matrix rich in large aggregating PGs. This matrix imbibes water allowing the NP to resist compressive forces. With disc degeneration, chondrocytic cells are replaced by fibrocytes synthesizing type I collagen.9 The baseline synthesis of type II collagen also declines, altering collagen fiber cross-linking.72,73,80 Additionally, a progressive loss of the PG matrix occurs, resulting in IVD dehydration and desiccation within the NP. These changes create a weaker biomechanical construct to resist compression and shear forces.81 Last, an overall decrease in disc cell density with age and degeneration is seen. In studies of human IVDs, Gruber et al reported that apoptosis, or programmed cell death, largely accounts for this depopulation over time, and that interventions which delay or halt apoptotic cell death may constitute a means of treating degenerative disc disease.74 In addition to mediating disc degeneration, biochemical events appear to play a significant role in producing disabling spinal pain.13,81,82 Biochemical events involved in discogenic pain production appear to include the production and release of inflammatory mediators and cytokines from the disc, vascular ingrowth into annular fissures, and the stimulation of free nerve endings in the outermost region of the disc.83–85 Studies have suggested nutrition as an important factor in the pathogenesis of disc disease.9 To maintain the steady-state metabolism of cells, the IVD requires proper nutrition, which is accomplished by diffusion of nutrients through the endplates and into the IVD. Trauma, cigarette smoking, and other factors that affect the integrity of the endplates and endplate vasculature may affect diffusion and disturb the nutrition of the disc cells.86 Vascular channels in the endplate of the IVD are particularly vital for maintaining the nutrition of the avascular NP. In degenerative discs, the diffusion capacity decreases creating a lower oxygen tension, decreased pH, and accumulation of catabolic byproducts. Typically, vascular channels at the endplate proliferate to maintain adequate nutrition of the disc. It has been claimed that the induction of new blood vessels in the endplate is facilitated by the activation of enzymes such as matrix metalloproteinases87; leading to the belief that with IVD injury the activation of these enzymes is the cause of increasing inflammation within the disc. This inflammation is the harbinger of further degeneration, culminating in a vicious cycle of accelerated degeneration. There are also reports that these channels ultimately disappear with disc degeneration and eventually become obliterated with calcification.88,89 Further research using microangiography and immunohistochemical analysis is needed to determine if the loss in vascularity at the end-plate can be reversed. Genetic factors play a significant role in the degenerative spinal cascade. A twin study by Sambrook et al examined the hypothesis that disc degeneration has a major genetic component. Spine MRI scans were obtained for 86 pairs of monozygotic (MZ) twins and 154 dizygotic (DZ) twins. A substantial genetic influence on disc degeneration was found.90 Further genetic predispositions to disc degeneration have been suggested by other studies on vitamin D receptor gene polymorphism.91,92 The authors noted that in 205 young adults, allelic variation (Tt allele) in the vitamin D receptor gene was associated with multilevel and severe disc degeneration. Unquestionably, the genetic effect on the disc degeneration cascade requires further analysis. Current treatment options for degenerative disc disease address its clinical symptom, pain, as opposed to the pathophysiologic root of the disorder. Furthermore, traditional strategies such as fusion of the involved motion segment are not reliable and may even create instability at adjacent levels or even adjacent level degeneration.93 In recent years, technologies such as disc replacement, aimed at restoring some degree of motion at the involved segment while eliminating pain, have begun to be studied.94 However, these motion preserving techniques are appropriate for more advanced stages of spinal degeneration. With a better understanding of the sequence of biologic and biomechanical events associated with spinal degeneration comes the opportunity for earlier interventions (Fig. 6.4). With early disc and/or facet degeneration, biologic strategies aimed at reversing or retarding the degenerative process are appealing.
Intervertebral Disc Degeneration
Intervertebral Disc Biomechanics
Mechanical Treatments
Facet Joint Biomechanics
Biologic Factors
Premise for Biologic Treatment
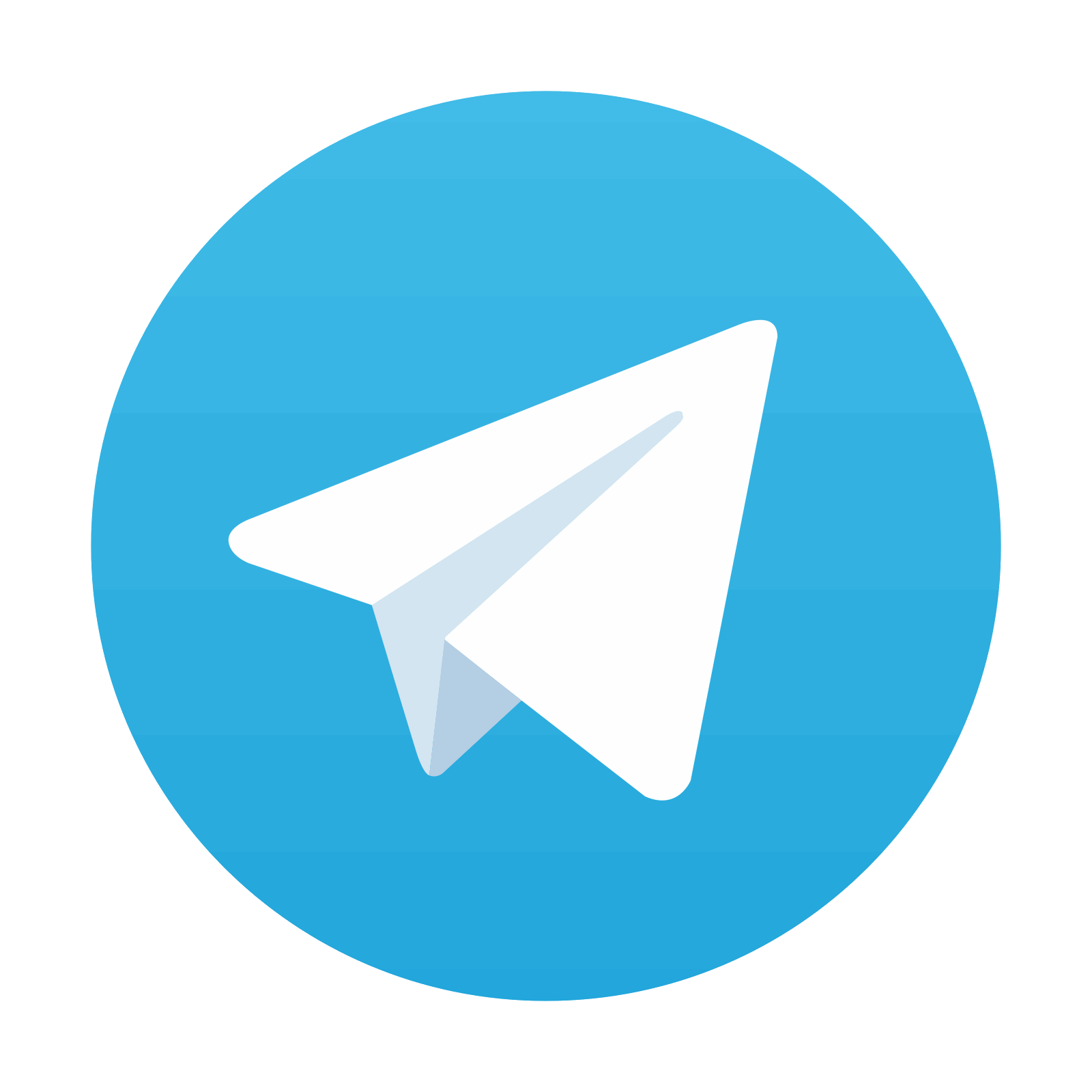
Stay updated, free articles. Join our Telegram channel

Full access? Get Clinical Tree
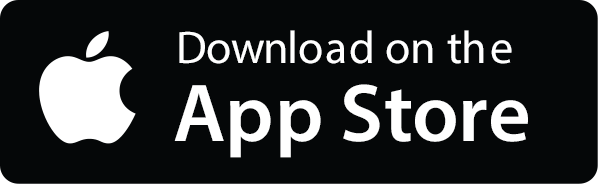
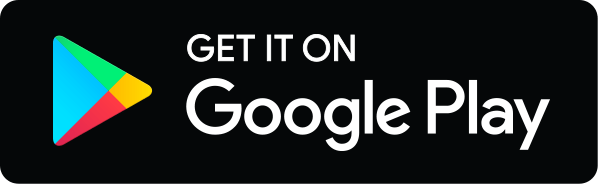