The Control of Posture
Movement begins and ends in posture: for most of the time, the motor system is not concerned with moving the body at all, but rather with keeping it still. This is especially true in Man, his precarious twin supports needing constant motor commands to keep him upright against the force of gravity.
One might perhaps think that this was merely a matter of keeping sufficiently rigid, once a stable balancing position had been found. But our centre of gravity is so high off the ground that this kind of passive stability is not enough: one need only compare the ease with which one can push over a tailor’s dummy with the near impossi bility of doing the same thing to a living person to realize that stability must involve active processes as well, which use proprioceptive feedback information.
The importance of support
In physical terms, whether someone – or something – falls over or not is entirely a matter of the vertical projection of his centre of gravity relative to their supports. If this line of projection (X) lies within the area defined by the points of contact with the ground (the support area), then all is well – small disturbances will result in a turning couple tending to restore the status quo. If X lies outside this critical area, then the system is unstable, and any further tilting will cause an ever-increasing rotational force that will make them fall over. Because the support area is much smaller in Man than in four-footed animals, maintaining an upright posture is correspondingly more difficult: a tilt of only a few degrees is sufficient to cause instability. Thus proper standing is not, as is often implied, just a matter of keeping upright: this man clearly has an excellent upright posture, but is equally clearly about to experience a postural disaster, because the vertical projection of his centre of gravity lies outside his region of contact with his support. Support is in every sense fundamental to posture, which must be controlled either by moving the centre of gravity relative to the feet, or moving the feet relative to the centre of gravity.
![]() |
![]() |
Two sources of information enable us to do this. The first is the existence of pressure receptors in the feet themselves that provide information about the distribution of support. Knowledge of differences of pressure at different points of support (the red arrows in the first figure) tells us precisely what we need to know to determine our postural state, namely the position of the vertical projection of the centre of gravity relative to the body’s supports. The second source of information is in the head: those special senses that can tell us about the
position and motion of the head relative to the outside world, the vestibular and visual systems. It is convenient to consider the use of information from the feet first, since this is a more direct process than the contribution of the special senses.
position and motion of the head relative to the outside world, the vestibular and visual systems. It is convenient to consider the use of information from the feet first, since this is a more direct process than the contribution of the special senses.
Responses to pressure distribution
Imagine that we had to design an automatic system for keeping a lunar module standing upright on uneven ground. If the craft leans in the direction of one particular leg, then that leg will experience more force. So a good strategy would be to have a rule that any leg that experienced more pressure than the others was automatically extended, while any that experienced less was shortened. The result would be a system that would always bring the vertical projection of the centre of gravity to the middle of the critical area defined by the points of support. It is not difficult to demonstrate a precisely analogous mechanism in four-footed animals: if we suspend a decerebrate animal in the air with its legs hanging down, and push up on the sole of one of its feet, the animal responds by extending the corresponding limb. This tonic response is called the positive supporting reaction; there is also a transient component of the response called extensor thrust, and it is often accompanied by stiffening of the limb and arching of the back. A variation is the buttress reaction, often observed on trying to pull a reluctant dog forward, increasing the differential pressure at the front: the extension of the front feet is obvious. The same mechanism may be seen at work in the postural sway reaction: if an animal’s body is pushed from the side, the shift in pressure on the feet results in marked extension of the limbs on the opposite side, and retraction of the others, so that the animal in effect leans against the experimenter.
![]() |
![]() |
It is easy to see how this mechanism would act to increase postural stability, as for example when the animal is standing on sloping ground. On the level, there is a roughly even distribution of pressure amongst the four feet, and hence no tendency for one leg to lengthen more than another. But if the animal is facing up a slope the pressure on the back feet is greater than on the front, and consequently the positive supporting reaction will result in rear limb extension and front limb flexion: the final result will be that the body adopts a more horizontal posture, and the projection of the centre of gravity is brought more nearly to the middle of the points of support.
It seems likely that analogous mechanisms may be used when sitting, kneeling, lying down and so forth, involving information about differences of pressure on different parts of the body other than the feet. A blindfolded animal, whose vestibular system has been destroyed (leaving cutaneous receptors as the only remaining source of postural information) will nevertheless right itself when laid on its side on the ground. If a plank is laid on top of it that reduces the ratio of the pressures experienced by the two sides of the body, this body righting reaction is inhibited, a phenomenon said to be used sometimes by veterinary surgeons to help restrain an animal for operation.

![]() |
If for some reason the projection of the centre of gravity has moved outside the critical area, then automatic stepping reactions are elicited that in effect move the feet in such a way as to track the centre of gravity: if one happens to be standing on one leg, the result is a hopping reaction. One can demonstrate these responses quite easily to oneself by first standing upright and then trying to fall over deliberately by leaning over: at some point, however hard one tries not to, reflex stepping or hopping comes into play and actual falling is prevented. Incidentally, if you try to fall over backwards in this way, you will also observe an involuntary upward flexion of the feet, as expected from the positive supporting reaction – though in these circum stances it is of no use whatever! It is also interesting to note that whereas in walking one is for most of the time in postural equilibrium – in the sense that one may ‘freeze’ at nearly any point of the walking cycle without falling over – this is not the case in running, when the centre of gravity is normally ahead of the support area. One can think of running as being a series of regular and almost unconscious stepping reactions in response to the bent-forward posture that the runner maintains.
The mechanisms described so far assume that some
sort of support is already present: there are other types of response that may be used to find postural support. Responses called placing reactions are used for acquiring postural support when none is present. If a blindfolded animal is suspended in the air and brought up to a table until the edge touches the backs of its paws, it will bring them smartly up to rest on the top of the table, in turn evoking a positive supporting reaction: a similar response may be triggered if the animal’s whiskers are brought to touch the table. These responses are, however, rather more complex then those described above, and probably involve the cerebral cortex: unlike the stepping and supporting reactions, they cannot be shown in decerebrate animals.
sort of support is already present: there are other types of response that may be used to find postural support. Responses called placing reactions are used for acquiring postural support when none is present. If a blindfolded animal is suspended in the air and brought up to a table until the edge touches the backs of its paws, it will bring them smartly up to rest on the top of the table, in turn evoking a positive supporting reaction: a similar response may be triggered if the animal’s whiskers are brought to touch the table. These responses are, however, rather more complex then those described above, and probably involve the cerebral cortex: unlike the stepping and supporting reactions, they cannot be shown in decerebrate animals.
Vestibular contribution to posture
The vestibular apparatus was described in Chapter 5. We saw that it provided two separate types of information about the head: angular velocity from the semicircular canals, and attitude relative to the effective direction of gravity, from the otolith organs. From the point of view of the control of posture it is of course the effective direction of gravity rather than its ‘real’ direction that matters. What determines, for instance, whether one falls over when standing in a bus that starts to accelerate is not the vertical projection of the centre of gravity vertically relative to the critical area, but rather its projection in the direction of the vector formed by gravity (g) and the horizontal linear acceleration (a) acting together, and this is what the utricle and saccule tell us.
![]() |
Each of these two divisions of the vestibular system gives rise to its own kinds of postural reactions, and so it is useful to distinguish between the static or tonic vestibular responses due to the otolith organs and the dynamic or phasic ones driven by the canals.
Static vestibular reactions
On the whole the otolith organs produce rather less powerful postural responses than do the canals, particularly in higher animals. But in the long run they are the only source of information about the absolute position of the head in space, since the canals essentially signal only changes of position. One of their main functions is in fact to keep the head upright despite changes in the position of the body, through appropriate changes in the tone of the neck muscles; these are the head righting reflexes. If the head is forcibly tilted in different directions, so that the head righting reflexes cannot operate, one can also observe compensatory static vestibulo-ocular reflexes that similarly help to maintain the normal attitude of the eyes with respect to the outside world. In Man these eye reflexes cannot easily be demonstrated, and if the head is tilted to one side the resultant counter-rolling of the eyes is seldom of more than a few degrees and so cannot maintain the correct orientation of the retinal image. But in animals such as the rabbit whose eyes essentially point sideways, tilting the head results in almost exact compensation over a wide range of angles of tilt: they can continue to check the horizon while nibbling the grass at their feet. While both these types of response obviously aid the sensory organs of the head by providing a stable ‘platform’ from which to operate, they are not of course strictly postural in the sense of contributing to the maintenance of equilibrium of the body as a whole.
![]() |
Reactions which are truly postural in this sense may be quite easily demonstrated in conscious animals, and are called tonic postural vestibular reflexes. If an animal is suspended in the air, and its head and body tilted nose downwards, one observes extension of the front legs and retraction of the rear. Corresponding limb movements are found if the animal is tilted in other direc tions, for example to the side: in each case, there is extension of the limbs in the direction of downward tilt and retraction of the others. The function of this response is clear: if
the animal is facing down a slope, this tonic vestibular response will assist the positive supporting reaction in shifting the centre of gravity backwards in relation to the feet, as may be seen in the human subject below.
the animal is facing down a slope, this tonic vestibular response will assist the positive supporting reaction in shifting the centre of gravity backwards in relation to the feet, as may be seen in the human subject below.

![]() |
![]() |
![]() |
Dynamic vestibular reactions
Because the canals are velocity sensitive, they effectively give advance warning that one is about to fall over, possibly before the otolith organs have sensed that there is yet an actual error in head position. Perhaps for this reason, their responses are particularly fast, and generally bigger and more dramatic than the static vestibular reactions. The types of response they generate fall essentially into the same categories as the tonic ones: thus there is a dynamic component to the head righting reflex, that may be elicited by selective stimulation of the canals alone, and one may also demonstrate clear effects of canal stimulation on the eyes and limbs. If we seat someone on a rotating chair and record their eye movements (in the dark, so that there is no visual input to the oculomotor system), we find that the eyes move in the opposite direction to that of the head with a velocity that compensates almost exactly for the rotation, keeping the eye stationary with respect to the outside world. Clearly this dynamic vestibulo-ocular reflex (VOR) cannot go on for ever, since sooner or later the eyes are going to reach the limit of their rotation in the orbit: what in fact is observed is that the smooth counter-rotation in one direc tion is interrupted at more or less regular intervals by a quick flick in the other direction, giving rise to a sawtooth-like eye movement called vestibular nystagmus.
The smooth, compensatory, movement is called the slow phase of the nystagmus, and the quick flick – which is essentially the same as an ordinary voluntary saccade – is called the quick phase (rather confusingly, it is the latter which is used in clinical practice to describe the direction of nystagmus, so that a subject turning to the right produces what would be called a nystagmus to the right, even though the more important, compensatory, component of the response is to the left). This allows the observer to maintain a stable visual field during the slow phase, with vision being only briefly compromised during the quick phase.

![]() |
During rotation of this kind at constant angular velocity the response declines over a period of some 20 seconds or more, on account of the natural adaptation of the canals (see Chapter 5), and so does the velocity of the slow phase. If the chair is suddenly stopped, and with it the semicircular canals, the fluid tends to continue. As a result the cupula is deflected in the opposite direction, and a corresponding reversed nystagmus is seen, which in turn declines with a time-course of some 20 seconds as the fluid ceases its motion; these two types of nystagmus are respectively known as per-rotatory and post-rotatory nystagmus. Vestibular nystagmus provides a convenient means for clinical investigation of the functioning of the semicircular canals, since the eye movements produced by caloric stimulation (Chapter 5) of each of the two labyrinths may be examined separately to reveal imbalance of function on the two sides.
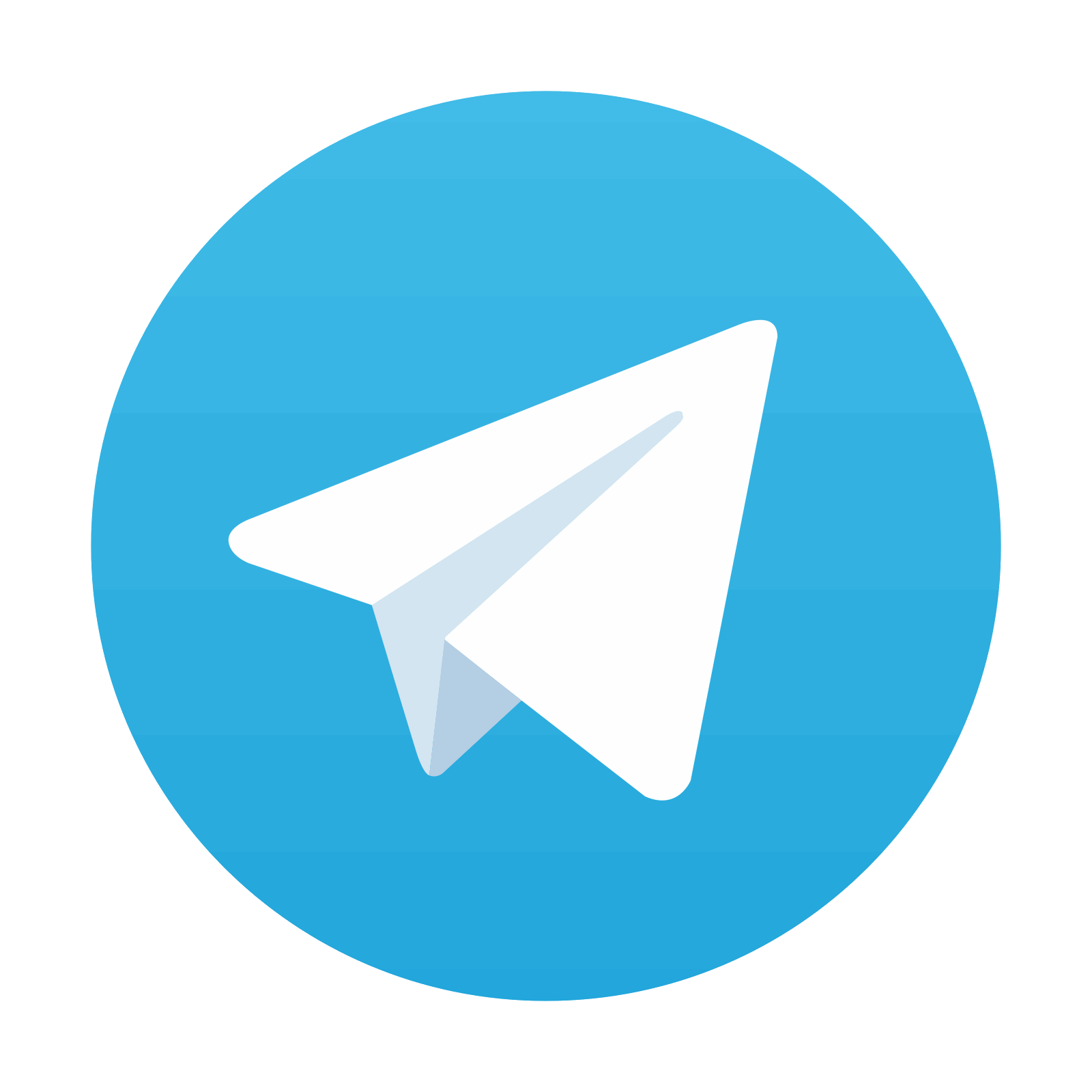
Stay updated, free articles. Join our Telegram channel

Full access? Get Clinical Tree
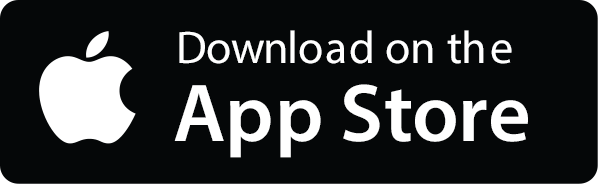
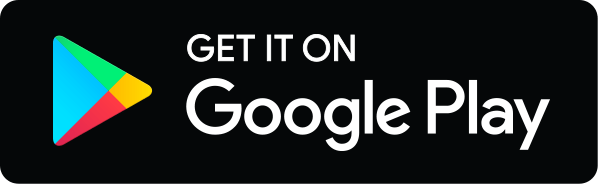