Fig. 15.1
AT1 receptors are involved in the altered neuronal activation induced by amphetamine exposure in response to different challenges. Angiotensin II (400 pmol) was administered intracerebrally and amphetamine (0.5 mg/kg) intraperitoneally. The neuronal activation was measured as Fos expression in the two brain areas from animals exposed to amphetamine 21 days before, pretreated with AT1 receptor antagonist (CV) or vehicle. The values were calculated as percentage respect control group (vehicle-saline animals exposed to saline challenge) and expressed as mean ± SEM. Two-way ANOVA analysis, *p < 0.05, n = 7–10
Repeated Amphetamine Exposure Modifies Brain Angiotensin II AT1 Receptor Functionality
Sodium depletion, which activates RAS, develops cross-sensitization effects leading to enhanced locomotor activity responses to amphetamine [97]. These experiments indicate that treatments implying RAS activation show reciprocal behavioral cross-sensitization with psychostimulants. In relation to these findings, our group, using a protocol of repeated amphetamine, found long-lasting changes affecting brain response to angiotensin II [98]. These alterations were revealed by exogenously intracerebrally injected angiotensin II in conscious rats, known to produce a marked increase in water and sodium intake, as well as an increased natriuresis [98]. All these effects have been previously and exhaustively described [99–103]; however, the results obtained in our study showed that previous exposure to repeated amphetamine administration modified the described effects of angiotensin II i.c.v. on these parameters in a long-term manner (1 week after amphetamine withdrawal) [104]. In this respect, it was found that repeated amphetamine exposure markedly decreased the sodium intake induced by angiotensin II; meanwhile, water intake was unaffected. Sodium intake behavior is likely to reflect the differential regulation of intracellular signaling pathways. In this regard, it has been hypothesized that differential AT1 receptors signaling pathways play separable roles in water and saline intake stimulated by angiotensin II [105, 106]. There are results that support this hypothesis, demonstrating that G protein-dependent pathways appear to be more important for water intake stimulated by angiotensin II, whereas G protein-independent pathways may be more relevant for angiotensin II-stimulated sodium intake [107]. In accordance with this last fact are the results showing that repeated i.c.v. angiotensin II administration reduced the dipsogenic effect without affecting sodium intake [108]. Therefore, a possible explanation for amphetamine exposure effects is the alteration of intracellular signaling pathway involved in the effects of angiotensin II on sodium intake. This altered response obtained in amphetamine-exposed animals may involve the desensitization of AT1 receptors through internalization of these receptors [109]. This last is supported by the evidence showing that angiotensin II i.c.v. induces internalization of AT1 receptors [110]. Accordingly, a decrease in the response to angiotensin II after a persistent or repetitive stimulation of AT1 receptors has been described [111]. Moreover, it has been shown that the early inducible genes, c-fos, c-jun, and delta-fos are involved in the control of transcription factors expression that ultimately mediate the desensitization to the angiotensin II signal [112]. In our laboratory, we found that angiotensin II i.c.v. induced a threefold increase in NAc and CPu neuronal activation; this effect was blunted by repeated amphetamine exposure. This decreased response could demonstrate an AT1 receptor desensitization induced by repeated psychostimulant administration. In this regard, AT1 receptor desensitization-reduced Fos expression has been described as a consequence of repetitive angiotensin II i.c.v. administration in different brain areas that co-expressed AT1 receptors [112]. Interestingly, these results are in agreement with those obtained in regard to the decreased response in sodium intake to angiotensin II i.c.v.
It is known that exogenous i.c.v. angiotensin II administration stimulates oxytocin release from the pituitary gland [113, 114]. It has been found that the increase of sodium intake through sodium deprivation or adrenalectomy decreases basal oxytocin levels; meanwhile, treatments that stimulate oxytocin secretion (e.g., hypertonic saline, lithium chloride, and copper sulfate) inhibit sodium intake in sodium-deprived rats [115–117]. Moreover, blockade of central oxytocin receptors before i.c.v. angiotensin II administration resulted in a potentiation of angiotensin II-induced sodium intake, although in the absence of exogenously administered angiotensin II, blockade of oxytocin receptors does not interfere with the dipsogenic properties of angiotensin II, nor does it stimulate sodium intake [115]. In rats, the oxytocin receptor antagonist administration-induced sodium intake is blunted by AT1 receptor antagonist administration [118]. This evidence supports the idea of an inhibitory oxytocinergic tone involved in the activation or disinhibition of AT1 receptors [118].
The results obtained in our study using repeated amphetamine administration reveal a long-lasting effect of amphetamine exposure.
Moreover, it is possible to suggest that the decreased response in sodium intake induced by angiotensin II i.c.v. in amphetamine-exposed animals could be attributed to an increased oxytocin response to angiotensin II as a consequence of AT1 receptors altered functionality. This explanation is supported by our results, showing that amphetamine exposure increased the number of Fos-oxytocin positive neurons in response to angiotensin II. The mechanisms by which angiotensin II i.c.v. induces natriuretic effects could involve brain oxytocin release [119]. It has been shown that angiotensin II i.c.v. activates oxytocin neurons in paraventricular and supraoptic nucleus [113–115]. In our study, the repeated amphetamine administration potentiated the activation of oxytocin neurons induced by angiotensin II i.c.v. in different oxytocinergic subnuclei of paraventricular and supraoptic nucleus, possibly showing an increased oxytocin response to angiotensin II because of the reduced AT1 receptor functionality mentioned above. Therefore, the repeated amphetamine exposure could reduce AT1 receptor functionality (desensitization-like) shown as a potentiated oxytocinergic response to i.c.v. angiotensin II that elicits a decrease in sodium intake, an increase in natriuresis, and decreases in plasma renin activity. These results are also supported by the increased number of Fos-oxytocin positive neurons in paraventricular and supraoptic nucleus in response to i.c.v. angiotensin II found in the amphetamine-exposed animals [104].
In conclusion, the results presented here support the view that long-lasting changes in brain RAS could be considered among the psychostimulant-induced neuroadaptations.
References
1.
Angrist B, Corwin J, Bartlik B, Cooper T. Early pharmacokinetics and clinical effects of oral D-amphetamine in normal subjects. Biol Psychiatry. 1987;22(11):1357–68.PubMed
2.
Sherer MA. Intravenous cocaine: psychiatric effects, biological mechanisms. Biol Psychiatry. 1988;24(8):865–85.PubMed
3.
Lieberman JA, Kinon BJ, Loebel AD. Dopaminergic mechanisms in idiopathic and drug-induced psychoses. Schizophr Bull. 1990;16(1):97–110.PubMed
4.
Klawans HL, Margolin DI. Amphetamine-induced dopaminergic hypersensitivity in guinea pigs. Implications in psychosis and human movement disorders. Arch Gen Psychiatry. 1975;32(6):725–32.PubMed
5.
Dackis C, Gold MS. Neurotransmitter and neuroendocrine abnormalities associated with cocaine use. Psychiatr Med. 1985;3(4):461–83.PubMed
6.
Antelman SM, Chiodo LA. Dopamine autoreceptor subsensitivity: a mechanism common to the treatment of depression and the induction of amphetamine psychosis. Biol Psychiatry. 1981;16(8):717–27.PubMed
7.
Kalivas PW, Pierce RC, Cornish J, Sorg BA. A role for sensitization in craving and relapse in cocaine addiction. J Psychopharmacol. 1998;12(1):49–53.PubMed
8.
Stewart J, Badiani A. Tolerance and sensitization to the behavioral effects of drugs. Behav Pharmacol. 1993;4(4):289–312.PubMed
9.
Pierce RC, Kalivas PW. A circuitry model of the expression of behavioral sensitization to amphetamine-like psychostimulants. Brain Res Brain Res Rev. 1997;25(2):192–216.PubMed
10.
Vanderschuren LJ, Schmidt ED, De Vries TJ, Van Moorsel CA, Tilders FJ, Schoffelmeer AN. A single exposure to amphetamine is sufficient to induce long-term behavioral, neuroendocrine, and neurochemical sensitization in rats. J Neurosci. 1999;19(21):9579–86.PubMed
11.
Valjent E, Bertran-Gonzalez J, Aubier B, Greengard P, Herve D, Girault JA. Mechanisms of locomotor sensitization to drugs of abuse in a two-injection protocol. Neuropsychopharmacology Off Publ Am Col Neuropsychopharmacology. 2010;35(2):401–15.
12.
Vanderschuren LJ, Kalivas PW. Alterations in dopaminergic and glutamatergic transmission in the induction and expression of behavioral sensitization: a critical review of preclinical studies. Psychopharmacology (Berl). 2000;151(2–3):99–120.
13.
Vezina P. D1 dopamine receptor activation is necessary for the induction of sensitization by amphetamine in the ventral tegmental area. J Neurosci. 1996;16(7):2411–20.PubMed
14.
Prasad BM, Hochstatter T, Sorg BA. Expression of cocaine sensitization: regulation by the medial prefrontal cortex. Neuroscience. 1999;88(3):765–74.PubMed
15.
Sorg BA, Kalivas PW. Effects of cocaine and footshock stress on extracellular dopamine levels in the medial prefrontal cortex. Neuroscience. 1993;53(3):695–703.PubMed
16.
Sorg BA, Davidson DL, Kalivas PW, Prasad BM. Repeated daily cocaine alters subsequent cocaine-induced increase of extracellular dopamine in the medial prefrontal cortex. J Pharmacol Exp Ther. 1997;281(1):54–61.PubMed
17.
Pierce RC, Reeder DC, Hicks J, Morgan ZR, Kalivas PW. Ibotenic acid lesions of the dorsal prefrontal cortex disrupt the expression of behavioral sensitization to cocaine. Neuroscience. 1998;82(4):1103–14.PubMed
18.
Tzschentke TM, Schmidt WJ. The development of cocaine-induced behavioral sensitization is affected by discrete quinolinic acid lesions of the prelimbic medial prefrontal cortex. Brain Res. 1998;795(1–2):71–6.PubMed
19.
Hedou G, Feldon J, Heidbreder CA. Effects of cocaine on dopamine in subregions of the rat prefrontal cortex and their efferents to subterritories of the nucleus accumbens. Eur J Pharmacol. 1999;372(2):143–55.PubMed
20.
Richard ML, Liskow BI, Perry PJ. Recent psychostimulant use in hospitalized schizophrenics. J Clin Psychiatry. 1985;46(3):79–83.PubMed
21.
Lippoldt A, Paul M, Fuxe K, Ganten D. The brain renin–angiotensin system: molecular mechanisms of cell to cell interactions. Clin Exp Hypertens. 1995;17(1–2):251–66.PubMed
22.
Steckelings UM, Bottari SP, Unger T. Angiotensin receptor subtypes in the brain. Trends Pharmacol Sci. 1992;13(9):365–8.PubMed
23.
Steele MK, Stephenson KN, Meredith JM, Levine JE. Effects of angiotensin II on LHRH release, as measured by in vivo microdialysis of the anterior pituitary gland of conscious female rats. Neuroendocrinology. 1992;55(3):276–81.PubMed
24.
Barnes NM, Costall B, Kelly ME, Murphy DA, Naylor RJ. Anxiolytic-like action of DuP753, a non-peptide angiotensin II receptor antagonist. Neuroreport. 1990;1(1):20–1.PubMed
25.
Kaiser FC, Palmer GC, Wallace AV, Carr RD, Fraser-Rae L, Hallam C. Antianxiety properties of the angiotensin II antagonist, DUP 753, in the rat using the elevated plus-maze. Neuroreport. 1992;3(10):922–4.PubMed
26.
Georgiev V, Tanaka M, Tsuda A, Koga C, Takeda S, Yokoo H, et al. Effects of angiotensin II on regional brain noradrenaline metabolism in non-stressed and stressed rats. Kurume Med J. 1992;39(4):235–44.PubMed
27.
Barnes NM, Costall B, Kelly ME, Murphy DA, Naylor RJ. Cognitive enhancing actions of PD123177 detected in a mouse habituation paradigm. Neuroreport. 1991;2(6):351–3.PubMed
28.
Barnes JM, Barnes NM, Costall B, Coughlan J, Kelly ME, Naylor RJ, et al. Angiotensin-converting enzyme inhibition, angiotensin, and cognition. J Cardiovasc Pharmacol. 1992;19(Suppl 6):S63–71.PubMed
29.
Dennes RP, Barnes JC. Attenuation of scopolamine-induced spatial memory deficits in the rat by cholinomimetic and non-cholinomimetic drugs using a novel task in the 12-arm radial maze. Psychopharmacology (Berl). 1993;111(4):435–41.
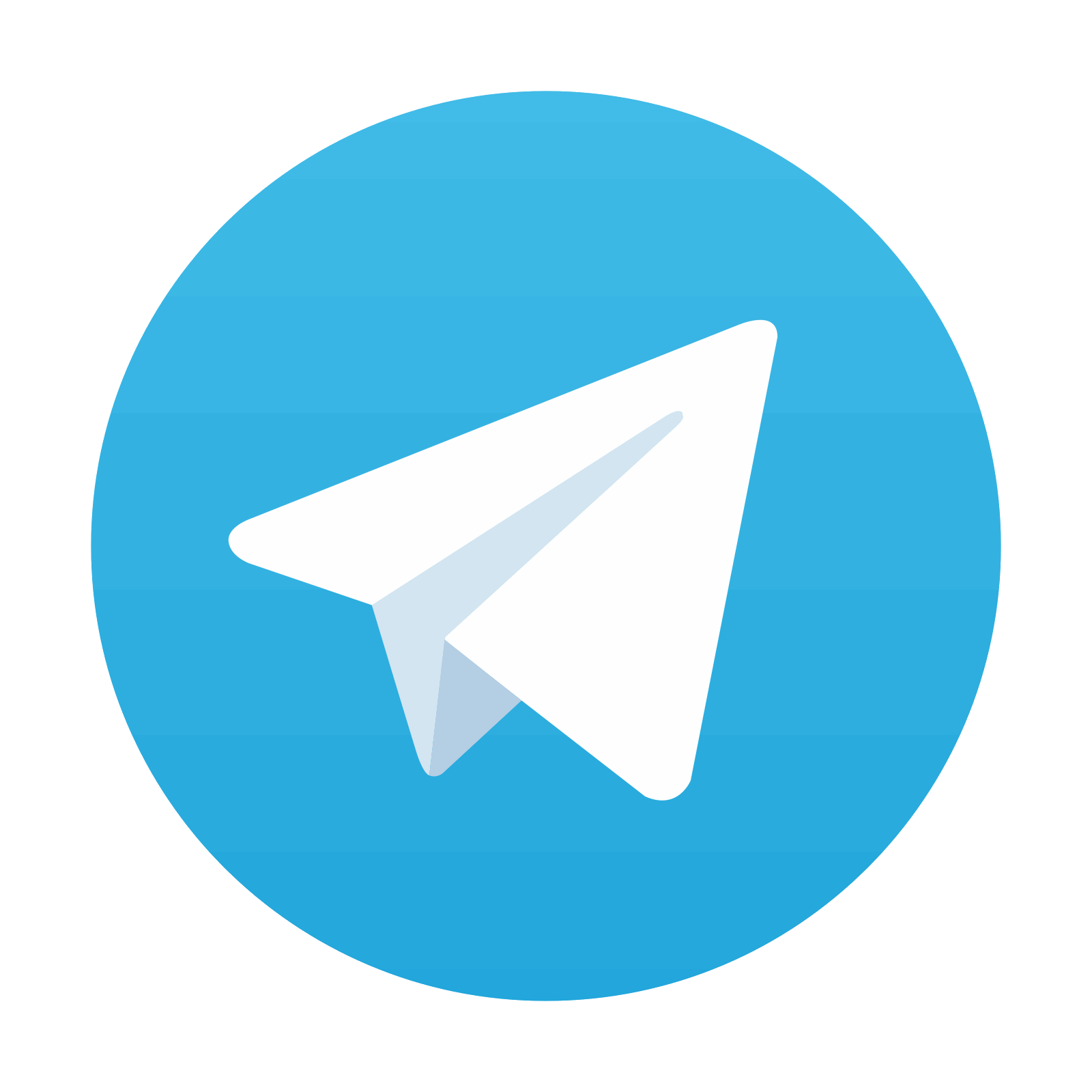
Stay updated, free articles. Join our Telegram channel

Full access? Get Clinical Tree
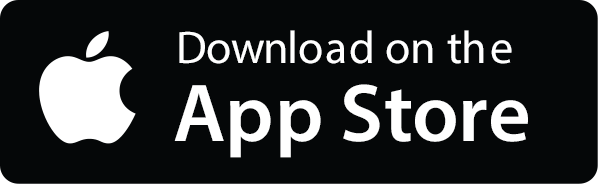
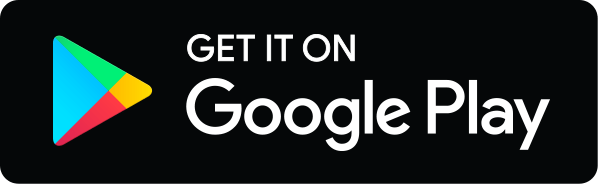