Figure 25.1. Dorsolateral FLE with seizures arising from the motor strip: a 21-year-old woman with simple partial seizures since a viral illness at age 9. Seizures began to cluster at age 19 after 10 years of response to medical therapy. The clinical pattern was an aura of nonspecific aura of dizziness, followed by right leg greater than arm tonic stiffening with preserved awareness. Stage I evaluation (A) shows CZ beta, which was not clearly determined to be ictal, and abundant muscle artifact. Stage II evaluation with left dorsolateral and interhemispheric subdural electrodes (map, B, MRI in C) showed ictal onset in primary motor cortex and gamma at contacts 8, 9, 12, and 13 in the left posterior grid (D). Subpial transections over the ictal onset zone were performed.
Anterior Cingulate.
The anterior cingulate gyrus has extensive connections throughout the frontal lobe as well as the limbic system, brainstem, and thalamus. It is involved in emotions, autonomic functions, perception of pain, and motor planning (13). Whereas the amygdala is implicated in the generation of fear in auras of TLE, the cingulate gyrus in the medial frontal lobe is thought to be the generator of fear auras in patients with FLE. This may relate to propagation of ictal discharges to the amygdala. Patients with proven lesional cingulate epilepsy have complex, hypermotor behaviors such as running, kicking, grasping, or thrashing. Behavioral changes including postictal or interictal agitation are seen, which can resolve after lesionectomy (14).
Primary Motor Area
Primary motor cortex in the medial frontal lobe (interhemispheric region) is responsible for movement of the lower extremity and sphincter control. Seizures arising from the leg motor cortex produce clonic movement of the thigh, leg, or foot and may produce bladder or bowel incontinence.
Dorsolateral Frontal Seizures
The dorsolateral frontal lobe includes the lateral primary motor strip, perirolandic region, and premotor areas. Seizures arising from premotor dorsolateral frontal cortex can cause tonic posturing of the arm or face, sensory phenomenon, or psychosensory auras including forced thinking (as seen with frontopolar seizures). Early spread to motor cortex will cause clonic activity while still conscious, whereas temporal lobe seizures are more likely to produce experiential or epigastric auras with motor activity once consciousness is impaired (15). Seizure propagation to Broca’s area may cause speech arrest, and contralateral head and eye version commonly occur with spread to the frontal eye fields. The EEG more often shows localized findings than with mesial or orbitofrontal seizure origin because of the close proximity of the cortex to the scalp. The scalp ictal EEG often shows fast activity in the beta frequency range, in contrast to temporal lobe seizures, which more often begin with rhythmic theta discharges. Ictal beta at seizure onset is often well localized in dorsolateral frontal lobe seizures and predicts a good postsurgical outcome, but the same is not necessarily true for all FLE patients (16,17).
Seizures that arise from the primary motor strip differ clinically from those of the SMA or premotor area in that they usually begin with contralateral facial or hand clonic activity followed by spread to the rest of that side of the body (i.e., a jacksonian pattern of spread), with speech arrest and then head and eye version. Patients might remain awake, or these seizures might generalize. Progression to asymmetric tonic limb posturing occurs as the seizure spreads to the SMA (9). These seizures usually have ictal EEG localized to the perirolandic area. Surgical resection of the motor strip will produce permanent weakness and clumsiness unlike the transient deficit associated with SMA resection. Figure 25.2 provides an example of a patient with epilepsy localized to the motor cortex.
Figure 25.2. Supplementary motor area seizures. A 20-year-old man has refractory GTC seizures and also SPS with left arm tonic extension occurring at night. The ictal surface EEG was nonlocalizing and demonstrated only diffuse attenuation during SPS. A and B: Cortical map showing seizure onset seen in the interhemispheric strip electrodes, primary and supplementary areas. C: Ictal IEEG with fast activity seen in the right interhemispheric electrodes.
Frontal Opercular and Insular Seizures
The operculum is the region of cortex overlying the insula and is subdivided into frontal, temporal, and parietal opercular cortices. The insula is considered by some as an anatomic part of the temporal lobe and a distinct lobar entity by others. This section will discuss seizures that arise from the frontal operculum and insula where they are confluent, as a subdivision of frontal lobe seizures.
Seizures arising from the frontal operculum often present with gustatory auras, apraxias of swallowing or mastication, and dysarthria. Patients may chew, may salivate, and may complain of abnormal laryngeal sensations, paresthesias in the arm and face, choking sensation, and trouble breathing. Early spread in a posterior and superior direction often causes facial clonic activity. An acquired epileptiform opercular syndrome has been described with ictal manifestations of above symptoms in addition to other seizure types. This has clinical similarities to the structural disease in Foix–Chavany–Marie syndrome and is analogous to Landau–Kleffner syndrome, but with symptoms localizing to the anterior rather than posterior operculum (18). Stereotactic EEG studies show that seizures with frontal opercular and insular onset produce the aforementioned symptoms at onset with hypermotor or asymmetric tonic posturing during sleep (19). This probably reflects variable spread patterns to mesial frontal structures (i.e., anterior cingulate or SMA cortex).
Localization of Frontal Lobe Seizures by EEG
The interictal EEG is often not helpful in the localization of frontal lobe seizures. Only a small proportion of patients have spikes restricted to the frontal lobe focus. Some patients have bifrontal or generalized spike-and-wave discharges, while others may have only temporal lobe interictal spikes. Other individuals have multifocal spikes, and some patients have a normal interictal EEG (3).
The ictal EEG is often unrevealing in frontal lobe seizures, sometimes obscured by movement and muscle artifact, and at other times either negative or poorly localized. Focal seizure onset occasionally is noted in the scalp EEG, usually with high-frequency beta frequency onset, but more often, the ictal EEG does not provide adequate localizing information. This may be due to the fact that much frontal cortex is buried, either within sulci or in medial or basal regions that are poorly sampled by the scalp EEG and by rapid contralateral spread of ictal discharges via the corpus callosum. Seizures beginning in mesial frontal lobe are particularly difficult to record with scalp EEG. Half of patients with mesial FLE will have generalized epileptiform patterns, nonlateralized midline discharges, or no EEG findings when seizures begin. FLE arising in dorsolateral cortex more often appear lateralized than mesial FLE in the ictal EEG, though remain less well localized than temporal lobe seizures. Incorrect seizure lateralization is more often observed in lateral FLE patients than in mesial FLE patients (20).
When the scalp ictal EEG is nonlocalized—as is common in the extratemporal lobe epilepsies—noninvasive neuroimaging techniques other than magnetic resonance imaging (MRI) including PET-FDG, magnetoencephalography, ictal single photon emission tomography, and functional MRI (including with simultaneous EEG) may help to localize the source of seizures and guide intracranial electrode placement that is required.
A recent series of FLE patients localized by FNIRS (functional near-infrared spectroscopy) showed the promise of this novel technique as an adjunct to video–EEG monitoring (21).
Differential Diagnosis
The clinical patterns of frontal lobe seizures include bizarre behaviors, which may be confused for nonepileptic events. Patients may be misdiagnosed as having parasomnias or receive a diagnosis of psychogenic nonepileptic seizures (PNES) and mistakenly thought to have a primary psychiatric disease. The challenge in diagnosing FLE is partly due to odd ictal behaviors, which may be confounded by ambiguous EEG findings as noted above. It is important to recognize stereotypy of behavior and nocturnal clustering, which suggest frontal lobe seizures rather than PNES or parasomnias. Patients with FLE may have preserved awareness during bilateral body movement, which may include bicycling and large thrashing movements. Ictal eye closure, pelvic thrusting, and prolonged seizure duration (i.e., >5 minutes) suggest PNES rather than FLE.
Surgical Considerations
After failure of medical therapy, surgical planning for FLE should take into account the possibility of eloquent cortex (e.g., areas important for language, movement, personality) in the epileptogenic zone. Intracranial EEG may be performed with subdural grids to over Broca regions and the motor strip, which can be mapped either intraoperatively or extraoperatively at the bedside with electrical stimulation of the cortex. If the seizure focus overlies eloquent cortex, performing multiple subpial transections to preserve columnar organization can minimize postoperative functional deficits or responsive neurostimulation (RNS) can be performed (22). The addition of an anterior corpus callosotomy (ACC) may be considered for FLE patients with frequent secondary generalization, since that procedure alone often offers benefit. For patients who have multifocal or bilateral onset zones, RNS implantation may also be considered. Deep brain stimulation of the centromedian nucleus was performed experimentally in patients with FLE, but might be more suitable for patients with generalized epilepsy syndromes (23). Vagus nerve stimulation also may be considered for refractory cases that are not candidates for focal resection or fail resective therapy.
Outcome of Surgery for Frontal Lobe Epilepsy
Frontal lobe resections have the worst seizure-free outcomes for epilepsy surgery, with 27% of patients found to be seizure free after surgery in a large metaanalysis (24), though some individual series demonstrate a seizure-free rate as high as 60% (17). In children, frontal lobe resections more often resulted in unsatisfactory outcomes than posterior resections in a meta-analysis of nonlesional cases. The authors found that complex partial seizures rather than generalized tonic–clonic seizures and focal cortical dysplasia identified on pathology were associated with better outcomes (25).
The class I outcome in the series of 16 patients reported by Laskowitz et al. (3) in 1995 was 67%. Fourteen of them had frontal lobe resections, of which half were accompanied by ACC. The remaining two patients received ACC alone. Follow-up was at least 5 years with a mean follow-up period of 46 months. Electrocorticography was performed intraoperatively for most patients, and five patients underwent chronic intracranial monitoring. A lower preoperative seizure frequency was associated with seizure-free outcome as was a later age at onset (3). Another series reported 55% seizure freedom at 1 year, which dropped to 27% at 5 years (26). The presence of generalized interictal spikes, extrafrontal MRI findings including hippocampal sclerosis, a normal MRI with either normal pathology or malformation of cortical development, acute postoperative seizures, and postoperative interictal spikes on EEG correlated with a recurrence of seizures postoperatively (26).
A more recent series at the same center of a larger cohort found 66% seizure freedom at 1-year, 52% at 2-year, and 44% at 5-year follow-up. Longer seizure duration (>5 years) was predictive of poor outcome especially in children and those with MCD and tumors (27). Another recent study of 58 FLE patients who underwent focal resection with long-term follow-up demonstrated a favorable outcome in 60% of patients. They did not find any difference in outcome based on the location of resection (28). An intracranial EEG study noted that an ictal onset zone of <2 cm and delayed spread of the ictal discharges to other lobes were associated with postoperative seizure freedom. Dominant resections were more often followed by poor outcome, probably because the presence of adjacent language cortex limited resection size (17).
OCCIPITAL LOBE EPILEPSY
Occipital lobe seizures may arise from either primary visual or association cortex. Elementary visual auras such as seeing lights and shapes arise from primary cortex, while more complex hallucinations arise from adjacent association cortex in occipital, posterior temporal, or parietal lobes. Eye blinking, eye flutter, and amaurosis are other common clinical phenomena. Gaze deviation, typically ipsilateral, without impairment of consciousness also has been reported (29).
In children occipital lobe epilepsy may be caused by a variety of conditions, such as trauma, tumors, and stroke, but specific causes include celiac disease, childhood occipital epilepsy syndromes including Panayiotopoulos and Gastaut types, mitochondrial disease, and posterior reversible encephalopathy syndrome. Lafora body disease is a form of progressive myoclonic epilepsy in which patients may experience occipital seizures early in the course of illness. Celiac disease is associated with occipitoparietal lobe calcifications and epilepsy (30). The typical calcifications occur unilaterally and are sinuous and double contoured with associated microgyria. These findings may be confused with Sturge–Weber syndrome but have a different pathophysiology. Children often do not have intestinal symptoms when diagnosed with epilepsy, although some may have had an intestinal syndrome in infancy. Folate levels are characteristically low. Antigliadin antibodies and intestinal biopsy help diagnose the condition, and seizures can be successfully treated with a gluten-free diet when initiated early (31).
Occipital lobe epilepsy can also be difficult to diagnose. The occipital lobe has three surfaces, the mesial, lateral, and inferior surfaces. Mesial and inferior generators are often difficult to identify and lateralize with scalp EEG. Incorrect localization and lateralization are seen commonly, and generalized seizure patterns can be seen in over one-quarter of patients (20). Patients with occipital epilepsy often have coexisting temporal lobe abnormalities in the EEG and may have dual pathology affecting both occipital and temporal lobes (32).
Surgical Considerations
After surgery, 46% of patients are seizure-free based on a meta-analysis with long-term follow-up (24). Satisfactory (meaning Engel I and II) outcomes have been reported between 45% and 70% in studies done since the year 2000, and more recently published series have shown better outcomes than older series with as many as 68% achieving class I or II outcome (33). Although only small series have been reported, it has been noted that resections that include the temporal lobe and hippocampus result in favorable outcomes, which may be due to close connections to mesial temporal structures (32). Also, ample coverage of all three surfaces of the occipital lobe with intracranial electrodes (the lateral, mesial, and tentorial surfaces) is associated with better outcome (32). Cortical mapping to determine the extent of primary visual cortex and utilizing intracranial visual evoked potentials may help minimize postoperative hemianopsias. The desire to spare the visual fields is the main factor limiting the extent of surgical resection, and perhaps seizure-free rates. Figure 25.3 demonstrates an example of occipital lobe epilepsy.
Figure 25.3. Occipital lobe epilepsy in a 15-year-old boy who had medically refractory complex partial seizures with an aura of flashing lights in the right visual field, progressing to oral automatisms and loss of awareness. A and B: Development of the left occipital discharge on surface EEG. C: 3D MRI rendering and skull x-rays showing the coverage of all three occipital surfaces with subdural strip electrodes. D and E: Cortical map with seizure onset at visual evoked responses shown. F: Intracranial recording demonstrating left inferior occipital onset of gamma.
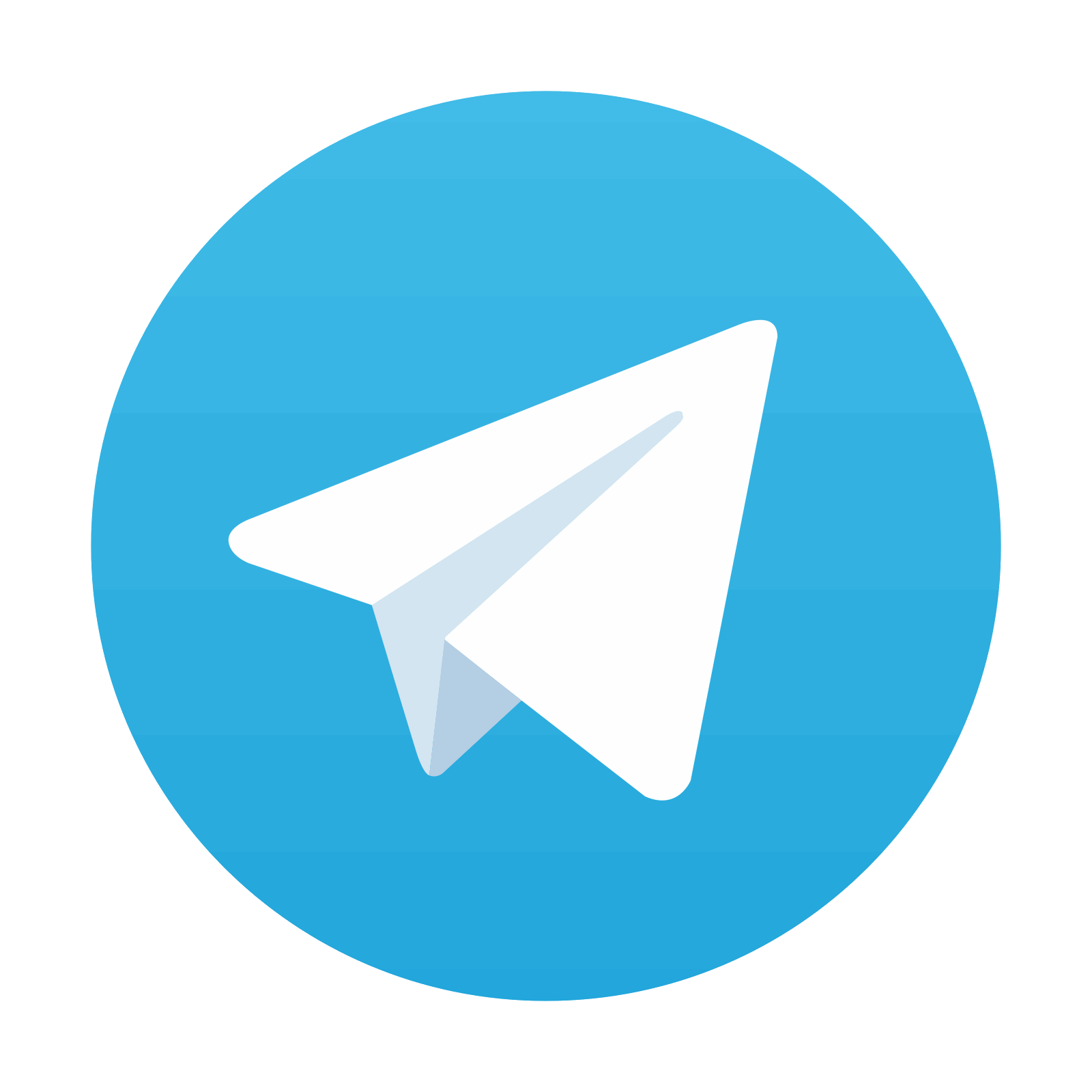
Stay updated, free articles. Join our Telegram channel

Full access? Get Clinical Tree
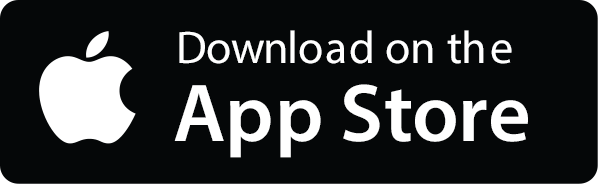
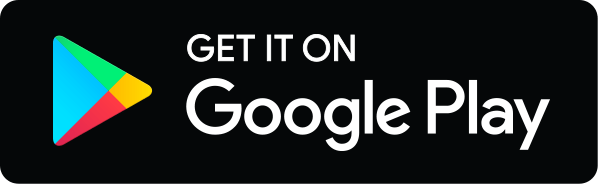