Fig. 6.1
Proposed syrinx filling mechanisms involving fluid flow from the fourth ventricle. Theory proposed by Gardner et al. (1957). (a) During systole, CSF is forced into the central canal. (b) During diastole the canal is closed and fluid cannot return to the fourth ventricle . Theory proposed by Williams (1970). (c) During Valsalva manoeuvres, CSF is forced from the spine into the cisterna magna. (d) After relaxing, the cerebellar tonsils act as a valve, preventing fluid returning to the spinal subarachnoid space. Fluid is therefore forced into the fourth ventricle and then into the syrinx. There is now abundant evidence that these theories do not explain the vast majority of syrinxes
Much of Gardner’s hypothesis appears to hold for syringomyelia in association with Chiari II malformation. In these cases, there is continuity between the expanded central canal and the fourth ventricle, and there is hydrocephalus (Milhorat et al. 1995a, b). The hydrocephalus and syringomyelia both resolve with ventricular shunting.
For other types of syringomyelia, which form the majority, there has been an accumulation of strong evidence against Gardner’s hypothesis: the subarachnoid space forms prior to the opening of the fourth ventricle outlets during development; there is usually no continuity between the fourth ventricle and the syrinx; the outlets of the fourth ventricle are not always obstructed in cases of Chiari I malformation; and Chiari malformation is not the only condition associated with syringomyelia.
Williams developed an alternative explanation for a force driving fluid from the fourth ventricle into the central canal (Williams 1970, 1972). He proposed that the outlets of the fourth ventricle are not obstructed and that fluid enters the cranial subarachnoid space when the spinal subarachnoid pressure increases with coughing and sneezing (Fig. 6.1). The cerebellar tonsils would then act like a valve to prevent fluid flowing back into the spinal subarachnoid space, resulting in a pressure differential between the cranial and spinal cavities. The only available pathway for fluid in the cranial subarachnoid space to reach the spine to restore pressure equilibrium would then be for it to flow into the fourth ventricle and then to the central canal, causing it to expand.
Evidence against this proposed mechanism includes the fact that syrinx cavities are not usually in continuity with the fourth ventricle and that syringomyelia occurs in association with other posterior fossa abnormalities and tumours that would not be expected to have the same valve mechanism as was proposed for the cerebellar tonsils in Chiari malformation.
The evidence against a direct flow of fluid from the fourth ventricle into the central canal or syrinx is compelling. Although posterior fossa decompression remains the mainstay of treatment for syringomyelia associated with Chiari malformation, this does not appear to be due to correction of the abnormalities proposed by Gardner or Williams. Plugging of the opening of the central canal is no longer recommended as part of this procedure (Vanaclocha et al. 1997; Ball and Dayan 1972).
6.4.2 Trans-parenchymal Flow
If the fluid in syrinx cavities is CSF and it has not reached the cavity directly from the fourth ventricle, it must flow across the cord tissue from the subarachnoid space. There has been much speculation about the possible route of such a fluid flow and the forces driving it.
In contrast to the proposal by Williams, Ball and Dayan suggested that a Chiari malformation would act to prevent spinal CSF from entering the cranial compartment during Valsalva manoeuvres (Fig. 6.2). They then speculated that the resulting increase in spinal CSF pressure could force CSF into perivascular spaces in the cord and that this fluid could coalesce to form a syrinx (Ball and Dayan 1972). Subarachnoid space obstruction from other causes such as post-traumatic arachnoiditis was said to produce a similar mechanism for fluid entry into the cord (Ball and Dayan 1972). The authors pointed to the pathological finding of enlarged perivascular spaces in syrinx cases as evidence for this theory.
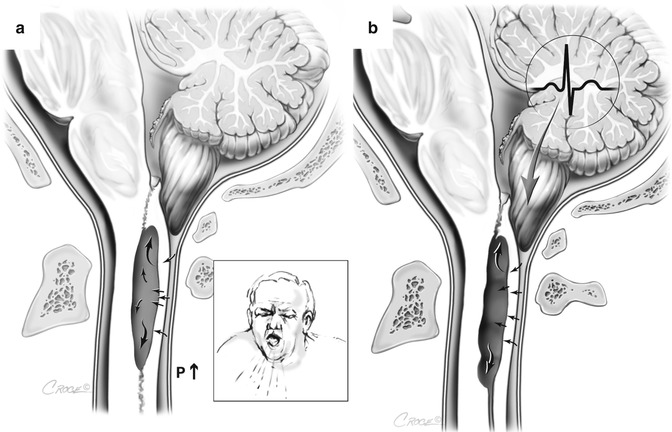
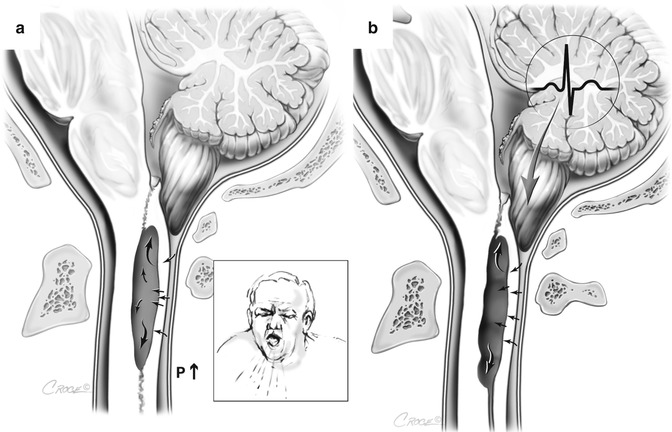
Fig. 6.2
Proposed trans-medullary filling mechanisms. Theory proposed by Ball and Dayan (1972). (a) The Chiari malformation acts to isolate the spinal subarachnoid space and that Valsalva manoeuvres increase the subarachnoid space pressure, forcing fluid into the cord. Theory proposed by Oldfield et al. (1994). (b) The cerebellar tonsils act as a ‘piston’ with each systole to increase spinal subarachnoid pressure and force fluid into the cord. These theories cannot explain expansion of a syrinx cavity, because the pressure in the cavity must exceed subarachnoid pressure for it to do so
Oldfield and colleagues proposed a similar mechanism, whereby the Chiari malformation imparts a piston-like effect on the spinal subarachnoid space, forcing fluid through either the perivascular spaces or interstitial spaces (Fig. 6.2) (Oldfield et al. 1994; Heiss et al. 1999). These authors provided cine-MRI and intra-operative ultrasound evidence of cerebellar tonsil movement in support of their theory, but had no direct evidence for fluid flow into the cord.
Support for a perivascular flow of fluid has arisen from the experimental work of Stoodley and colleagues, who used tracers of CSF bulk flow to demonstrate perivascular flow from the subarachnoid space to the central canal in normal animals (Stoodley et al. 1996, 1997), and from Klekamp et al., who showed oedema and enlarged perivascular spaces in a model of arachnoiditis (Klekamp et al. 2001). Further work showed that perivascular flow of CSF from the subarachnoid space occurs in models of canalicular and extracanalicular syringomyelia (Fig. 6.3) (Stoodley et al. 1999; Brodbelt et al. 2003b) and that flow is dependent on arterial pulsations (Stoodley et al. 1997).
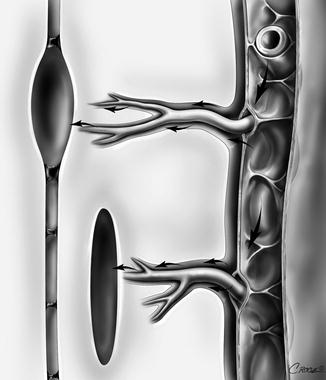
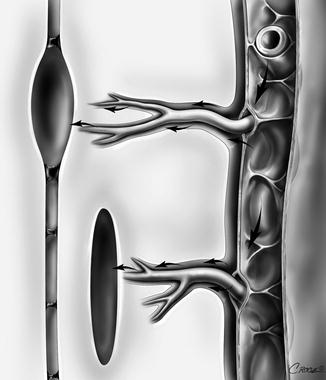
Fig. 6.3
Perivascular spaces as a proposed pathway for CSF flow in both canalicular and extracanalicular syringomyelia. Flow from the subarachnoid space enters the perivascular spaces , which narrow as they penetrate deeper into the cord. Possible mechanisms for such a flow to create a higher mean syrinx pressure than mean subarachnoid pressure include a partial valve effect of the perivascular space and a mismatch in the timing of the arterial and CSF pulse waves (Bilston et al. 2003, 2010)
A major problem with any proposed explanation for syrinx formation from a trans-parenchymal flow of CSF from the subarachnoid space is the simple physical fact that increasing pressure on the outside of the cord cannot create an expanding cyst within the cord. For a cavity to enlarge, the pressure within it must exceed the surrounding pressure, and this cannot occur with flow that is driven by an increase in pressure in the subarachnoid space. Several investigators have attempted to address this. Bilston and colleagues have examined the pulsatile properties of fluid flow in perivascular spaces and the subarachnoid space (Bilston et al. 2003, 2010). Using computational modelling, they demonstrated that the anatomical characteristics of the perivascular space could act as a ‘leaky’ one-way valve for pulsatile CSF flow. In addition, a timing mismatch between the arterial wave and CSF pressure wave arriving at the interface between the subarachnoid space and the perivascular space could act to increase flow (Fig. 6.4). They indicate this occurs when the CSF peak pressure occurs at a different time to the arterial pulse peak pressure, resulting in lower resistance to CSF inflow than outflow (Bilston et al. 2010). It was suggested that Chiari malformation and other obstructions in the subarachnoid space could act to create the timing mismatch.
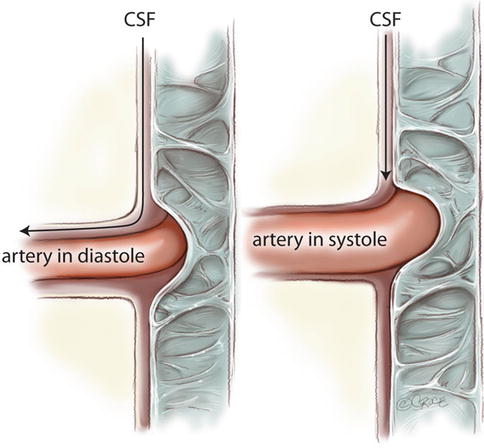
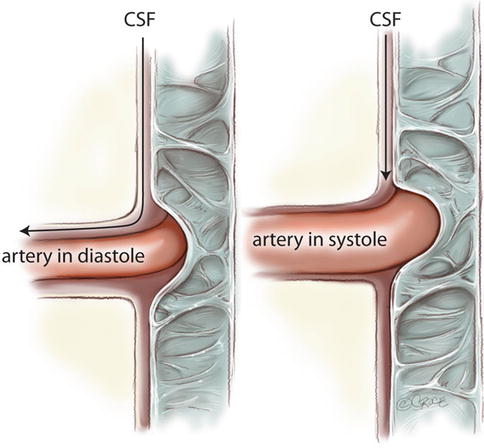
Fig. 6.4
A phase difference in pulsations may explain a valvelike effect of perivascular flow. Obstructions of the subarachnoid space could be responsible for slowing the pulse transmission to create a phase mismatch. If the CSF systolic wave arrives at the cord surface during arterial diastole, the perivascular space will be open, and flow will be greater than if the CSF pulse arrives during arterial systole, when the perivascular space will be smaller (Bilston et al. 2010)
An alternative explanation is that stenosis of the subarachnoid space could lead to a focal increase in pressure in the spinal cord with Valsalva manoeuvres, the so-called ‘elastic jump ’ (Carpenter et al. 2003). Although computational modelling has shown this to be a theoretical possibility, the magnitude of the effect appears to be too small to be significant (Elliott et al. 2009).
6.4.3 Obstruction of Outflow
It appears likely that there is a continual flow of fluid into the spinal cord and also into syrinx cavities. Unless a syrinx is enlarging, the outflow must equal the inflow. The physiology of fluid outflow is not known, but one possible explanation for syrinx formation and enlargement is the obstruction of outflow. This concept was initiated by Ellertsson and Greitz, who measured syrinx and subarachnoid space pressures in patients, showing higher pressure in syrinx cavities, although this was not significant. They suggested an impairment of outflow, although did not speculate as to the mechanism of this (Ellertsson and Greitz 1970).
Aboulker suggested that the pressure on the spinal cord at the cervicomedullary junction prevents CSF draining rostrally along the central canal to the fourth ventricle (Aboulker 1979). Although such a rostral flow has been demonstrated in experimental animals, it is not known whether this occurs in humans (Milhorat et al. 1991). Theoretically, fluid could also drain through spinal cord parenchyma, and cord compression might compress the extracellular space or reduce permeability, thus restricting outflow.
Recently, Koyanagi and Houkin have suggested that an increase in spinal venous pressure might impair absorption of extracellular fluid, resulting in accumulation of fluid in the cord and syrinx formation (Koyanagi and Houkin 2010).
There is little clinical or experimental evidence to support or refute these theories. The physiology of fluid outflow from the spinal cord and syrinx cavities is a largely unexplored area that may be important in syringomyelia physiology and is deserving of more research attention.
6.4.4 Transmitted Pressure Effects on Existing Cavities
Rather than fluid being forced into the cord by pressure changes in the subarachnoid space or physiological changes in perivascular flow, an alternative view is that pressure exerted on the cord surface causes rostral-caudal dissection of an existing cavity, leading to enlargement of the cavity. This concept began with the description by Williams of a ‘suck and slosh ’ mechanism for expansion of post-traumatic syrinx cavities. He suggested that increases in pressure in the subarachnoid space cause pressure on a cavity, which then dissects into the surrounding cord tissue, enlarging the potential space for the cavity, which then fills with extracellular fluid (Williams 1992). This mechanism has also been supported by the work of Oldfield and colleagues (Oldfield et al. 1994), who used intra-operative ultrasound to show that the cord and syrinx became compressed with each arterial pulsation and in synchrony with the descent of the cerebellar tonsils of a Chiari malformation. They suggested that this compression would cause extension of the syrinx cavity and that the fluid filling the cavity came from the subarachnoid space.
These theories rely on the presence of an initial cavity that can subsequently be enlarged by the putative dissection process. It is possible that this may apply to post-traumatic syringomyelia, where initial cavities form from haemorrhage and ischaemia. However, there is no evidence for a similar process in those cases associated with Chiari malformation. In addition, this process could at best explain the enlargement of a syrinx but not an increase in pressure relative to the subarachnoid space.
6.4.5 Summary of Hydrodynamic Theories
The available evidence does not support a fourth ventricular origin for syrinx fluid. There is good evidence that at least some syrinx fluid originates from the subarachnoid space and that the route of this fluid flow is via the perivascular spaces. However, theories that invoke increases in subarachnoid space pressure as the driving force are not sufficient to explain syrinx formation and enlargement. It is possible that complex relationships between CSF and arterial pulsations or the anatomical characteristics of the perivascular spaces explain the accumulation of fluid inside the cord. Pulsations exerted on the surface of the cord may contribute to cord tissue damage but seem insufficient to explain the development of high-pressure cavities. The possible role of perturbations in fluid outflow remains largely unexplored.
6.5 Other Sources of Fluid
None of the hydrodynamic theories have adequately explained syrinx formation and enlargement. Alternative theories have been put forward to suggest that syrinx fluid is not of CSF origin and that hydrodynamic factors driving CSF flow are not responsible. These proposed mechanisms have been used to explain the development of cavities in association with intramedullary tumours , or have suggested that syrinx fluid is derived from interstitial fluid , or originates through abnormalities of the blood-spinal cord barrier or even cellular fluid transport mechanisms.
6.5.1 Tumours
Syringomyelia occurs in association with posterior fossa tumours and with spinal extramedullary and intramedullary tumours. In general, it is reasonable to propose that the filling mechanism in posterior fossa tumour cases may be similar to the process that occurs with Chiari malformation and may be hydrodynamic in origin. Spinal extramedullary tumours may act by causing partial blockage of the subarachnoid space and could be thought of as having a similar underlying aetiology to cases associated with spinal arachnoiditis.
The particular tumour type that is likely to have a unique pathophysiology is the intramedullary tumour . Although expansion of the cord could theoretically result in obstruction of the subarachnoid space, it is our experience that many tumours are small and the subarachnoid space does not appear to be affected in the majority of cases. Intramedullary tumours with particularly high rates of syrinx development include haemangioblastomas and ependymomas (Samii and Klekamp 1994).
A possible explanation for syrinx development in association with intramedullary tumours is that the cystic cavity is part of the tumour itself (Barnett 1973), but for some tumour types at least, the syrinx wall is gliotic tissue (Lohle et al. 1994). There is limited information regarding the composition of tumour-associated syrinx fluid, but there is some evidence that it is high in protein (Lohle et al. 1994), suggesting that the fluid comes directly from the tumour or its vasculature.
It has been suggested that, in haemangioblastomas, the vasculature is leaky and the interstitial pressure is high, leading to extravasation of plasma (Lonser et al. 2006). In support of this hypothesis, Lonser et al. have reported a case demonstrating progressive leakage of contrast medium from a haemangioblastoma into the surrounding cord tissue (Lonser et al. 2006).
Samii and Klekamp argue that the pathophysiology is likely to include some abnormality of CSF flow in addition to secretion of fluid and protein by the tumour. They cite the predominant rostral location of syrinx cavities relative to the tumour and the higher rates of syrinx formation in tumours in the cervical cord to support this view (Samii and Klekamp 1994).
We are not aware of any experimental models of tumour-associated syringomyelia, and the clinical evidence is limited. However, it does seem likely that specific tumour-related factors are more important than CSF dynamics in this type of syrinx.
6.5.2 Interstitial Fluid
Interstitial fluid has been proposed by numerous authors as a source of syrinx fluid. One line of reasoning is that tethering of the cord by arachnoiditis results in tensile radial stress, which lowers the pressure in the cord and leads to inflow of extracellular fluid (Bertram et al. 2008).
Josephson and colleagues provided experimental evidence for a theory that pulse transmission through the cord, past a region of subarachnoid block, would cause expansion of the cord below the block (Josephson et al. 2001). The proposal is that, with each arterial pulsation, the pulse wave in the subarachnoid space is blocked, but the wave in the cord continues, creating a higher pressure in the cord than in the surrounding subarachnoid space, thus expanding the cord. The expanded cord would then fill with extracellular fluid. A similar explanation was proposed and tested using an electrical circuit model of CSF dynamics (Chang and Nakagawa 2003, 2004). In this model, the pressure was transmitted down the central canal, forcing fluid out of this channel, below the level of the subarachnoid block where the surrounding subarachnoid pressure was lower.
Greitz subsequently proposed a somewhat different explanation (Greitz 2006). He suggested that narrowing of the subarachnoid space causes an increase in CSF velocity at the region of narrowing. The increased fluid velocity has a Venturi effect , lowering the pressure in the subarachnoid space and causing a suction effect on the cord, expanding it during each systole. According to Greitz, extracellular fluid would accumulate in the expanded cord, forming a syrinx (Greitz 2006).
Klekamp suggested that interstitial fluid could be important in canalicular and extracanalicular cavities, by exceeding the normal fluid capacity of the extracellular space. This might occur due to blockage of perivascular spaces, cord tethering , changes in vascular flow, or obstruction of CSF flow (Klekamp et al. 2002).
In common with the hydrodynamic theories, many of the theories proposing an interstitial fluid origin cannot explain a higher pressure in the syrinx than the spinal cord tissue and subarachnoid space. Instead, they imply passive filling of syrinx cavities, which does not fit with our clinical observation that the pressure in many syrinx cavities appears much higher than the surrounding subarachnoid space.
6.5.3 Blood-Spinal Cord Barrier Disruption
Several authors have suggested that fluid crossing a deficient blood-spinal cord barrier may contribute to syrinx fluid (Fig. 6.5). Clinical case reports have supported a role for barrier disruption, by demonstrating contrast enhancement around syrinx cavities on MR scans (Lonser et al. 2006; Ravaglia et al. 2007).
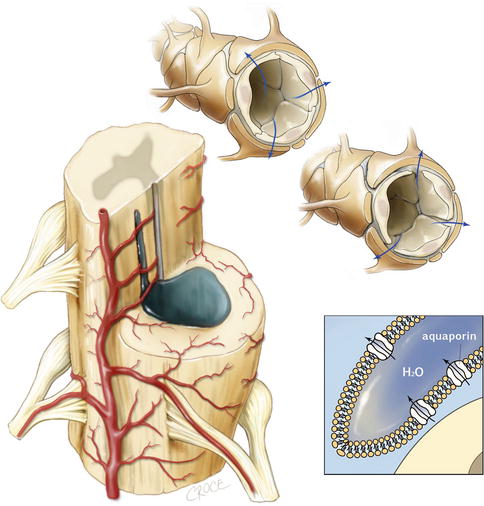
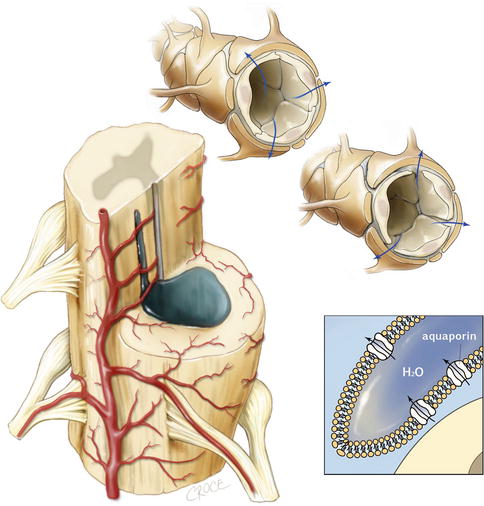
Fig. 6.5
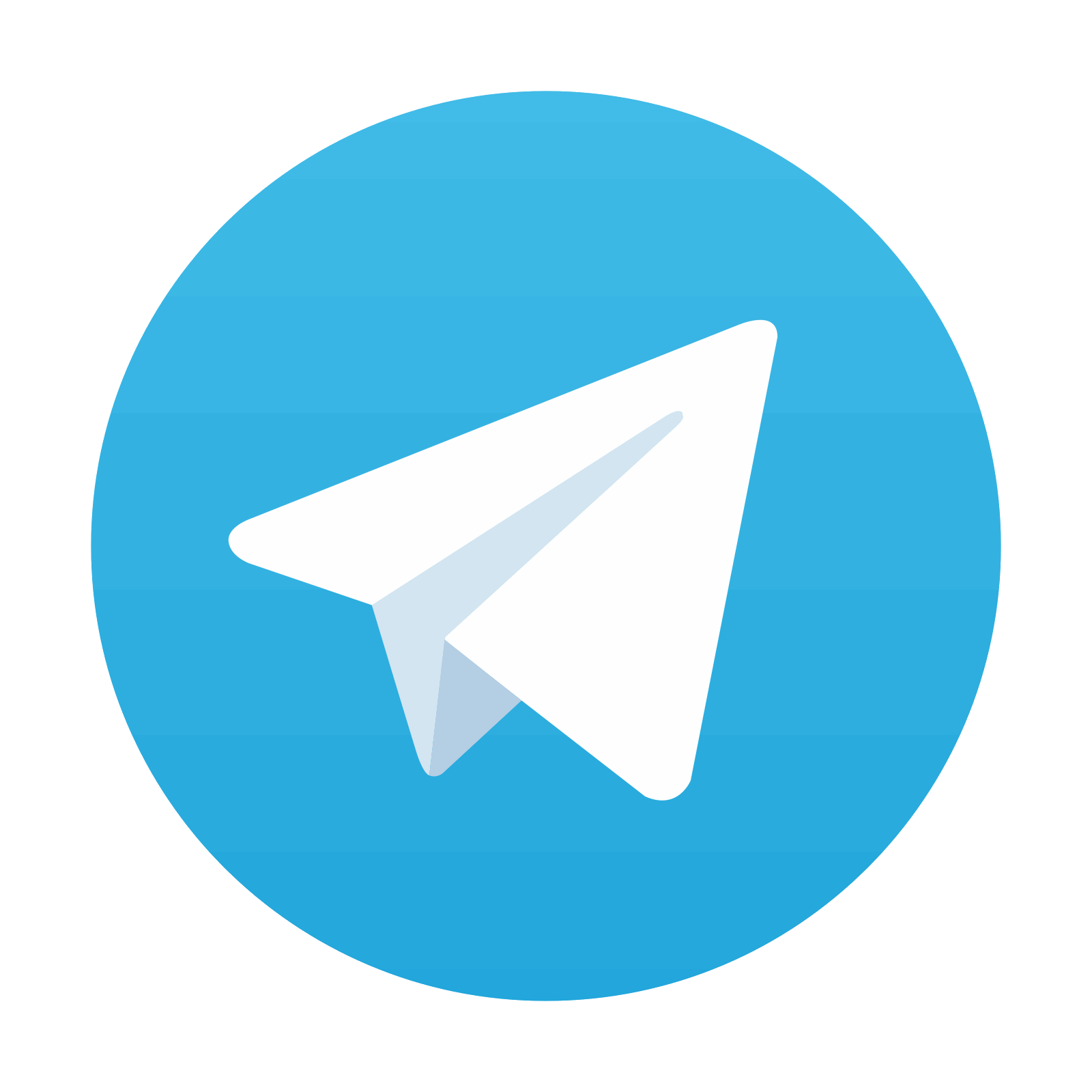
Vascular and tissue sources of fluid or impaired absorption may result in a syrinx pressure higher than the subarachnoid space. Left: the syrinx cavity is often in the highly vascular grey matter and surrounded by vessels. Top and top-right: an impaired blood-spinal cord barrier allows fluid to cross the vessel wall and add to the syrinx volume. Bottom right: abnormalities of aquaporin expression may also allow fluid to leak across vessels or may impair absorption of syrinx fluid into the vasculature
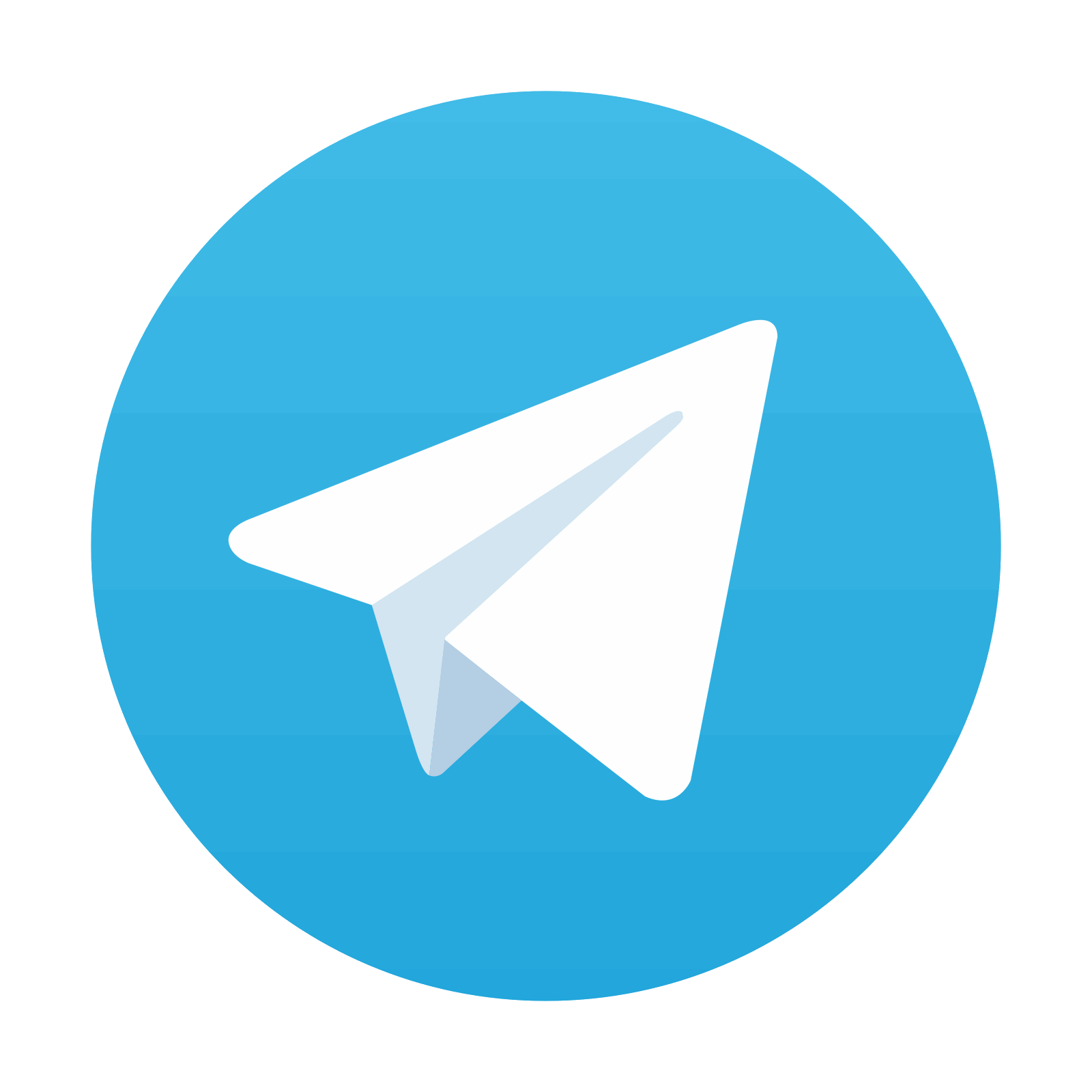
Stay updated, free articles. Join our Telegram channel

Full access? Get Clinical Tree
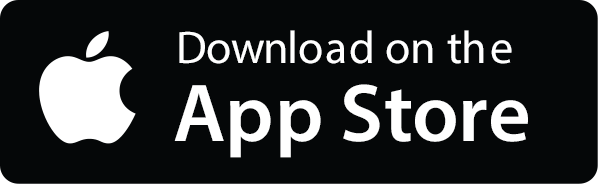
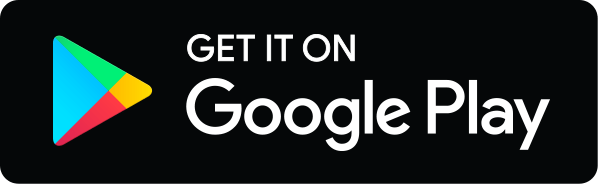
