The Ketogenic Diet
Douglas R. Nordli Jr.
Darryl C. De Vivo
INTRODUCTION
The ketogenic diet is a high-fat, low-carbohydrate, low-protein regimen that has been used for more than 70 years in thousands of patients. It is an effective and safe medical treatment for epilepsy, but it must be judiciously applied and carefully monitored.
Historical Highlights
There are biblical references to the salutary effects of starvation upon seizure control, but the earliest scientific observations were made by Geyelin at the Presbyterian Hospital in 1921 (1). Shortly thereafter, Wilder proposed a high-fat diet to mimic the effects of starvation (2). Since this high-fat diet increased the production of ketone bodies, the regimen became known as a “keto,” or ketogenic diet. It was known that ketone bodies could be found in the urine of patients with diabetes and that they were produced when fatty acids were oxidized. This led to the notion that ketone bodies were potentially toxic metabolites of fatty acid degradation and that their anticonvulsant effect was caused by a sedative property, similar to the mechanisms of action of the available anticonvulsants of that era—bromides and phenobarbital.
This notion was challenged when Krebs suggested that ketone bodies were fuel for respiration in 1961 (3). In 1967, Owen and colleagues proved that ketone bodies were the major fuel for brain metabolism during starvation (4). Appleton and De Vivo (1974) developed an animal model, whereby they demonstrated that utilization of ketone bodies during starvation alters brain metabolites and increases cerebral energy reserves (5). In 1976, Huttenlocher showed that the level of ketosis correlated with efficacy (6). Livingston and associates reported extensive (41-year) experience with the ketogenic diet for the treatment of myoclonic seizures of childhood, stating that it completely controlled seizures in 54% of his patients and markedly improved control in another 26% (7). Subsequently, valproate (VPA) and other antiepileptic drugs (AEDs) effective for the control of myoclonic seizures were introduced in the United States. Yet, despite the availability of these agents, the ketogenic diet continues to be used in many centers across the country.
PRODUCTION AND UTILIZATION OF KETONE BODIES
The major precursors of ketone bodies are nonesterified fatty acids. During the fasting state, the decrease in blood glucose reduces plasma insulin production, stimulates lipolysis in fatty tissues, and increases the flux of nonesterified fatty acids to the liver. Nonesterified fatty acids can be esterified or metabolized to ketone bodies. The fate of fatty acids in the liver is determined, at least in part, by the carbohydrate status of the host (8). A critical component of this regulation is malonyl-coenzyme A (CoA), an intermediate in the pathway of lipogenesis (9,10). Malonyl-CoA inhibits carnitine acyltransferase I, which is needed to shuttle long-chain fatty acyl-CoA into the mitochondria for oxidation. The production of glucose from glycogen provides the carbon source for lipogenesis and, in particular, malonyl-CoA. If glucose is reduced, so is malonyl-CoA. The reduction in malonyl-CoA decreases the inhibition of (or increases the net activity of) carnitine acyltransferase. This allows more movement of fatty acids into the mitochondria, where fatty acyl-CoA is converted to acetyl-CoA and later to acetoacetate (AcAc). AcAc is in equilibrium with β-hydroxybutyrate, the major ketone body used by the brain.
Passage of ketone bodies into the brain may be the critical factor limiting the rate of brain utilization of these chemicals. Movement of ketone bodies into the brain relies on the monocarboxylic transporter-1 system. This is upregulated during fasting in adults and during milk feeding in
neonates (11,12). Fasting studies in humans demonstrated that the brain’s ability to extract ketone bodies is inversely related to the age of the subject (13). In contrast to glucose, ketone bodies can pass directly into mitochondria without being processed in the cytosol. Also in contrast to glucose, ketone bodies may be used directly by neurons for metabolism (14).
neonates (11,12). Fasting studies in humans demonstrated that the brain’s ability to extract ketone bodies is inversely related to the age of the subject (13). In contrast to glucose, ketone bodies can pass directly into mitochondria without being processed in the cytosol. Also in contrast to glucose, ketone bodies may be used directly by neurons for metabolism (14).
Once inside the mitochondria, β-hydroxybutyrate is converted to AcAc and then to AcAc-CoA. The enzyme that facilitates this is 3-oxoacid-CoA-transferase, or succinyl-CoA-acetoacetate-CoA-transferase. As the name implies, this conversion requires commensurate conversion of succinyl-CoA to succinate. It is possible that reduced blood glucose and increased blood ketones may be needed to induce the activity of this enzyme (15).
SCIENTIFIC BASIS OF THE DIET
Scientific studies of the ketogenic diet have revealed important biochemical and metabolic observations. The animal model designed by Appleton and De Vivo permitted study of the effect of the ketogenic diet on cerebral metabolism (5). Adult male albino rats were placed on either (a) a high-fat diet containing (by weight) 38% corn oil, 38% lard, 11% vitamin-free casein, 6.8% glucose, 4% United States Pharmacopeia salt mixture (USP), and 2.2% vitamin diet fortification mixture; or (b) a high-carbohydrate diet containing (by weight) 50% glucose, 28.8% vitamin-free casein, 7.5% corn oil, 7.5% lard, 4% USP salt mixture, and 2.2% vitamin diet fortification mixture. Parallel studies were conducted to evaluate electroconvulsive shock responses and biochemical alterations. These studies revealed that the mean voltage necessary to produce a minimal convulsion remained constant for 12 days before the high-fat diet was started and approximately 10 days after beginning the feedings (69.75 ± 1.88 volts). After 10 to 12 days on the high-fat diet, the intensity of the convulsive response to the established voltage decreased, necessitating an increase in voltage in order to reestablish a minimal convulsive response. Approximately 20 days after beginning the high-fat diet, a new convulsive threshold was achieved (81.25 ± 2.39 volts; p <0.01). When the high-fat diet was replaced by the high-carbohydrate diet, a rapid change in response to the voltage was observed. Within 48 hours, the animal exhibited a maximal convulsion to the electrical stimulus that previously had produced only a minimal convulsion, and the mean voltage to produce a minimal convulsion returned to the prestudy value (70.75 ± 1.37 volts).
Blood concentrations of β-hydroxybutyrate, AcAc, chloride, esterified fatty acids, triglycerides, cholesterol, and total lipids increased in the rats fed on the high-fat diet. Brain levels of β-hydroxybutyrate and sodium were also significantly increased in the fat-fed rats.
Hori and associates studied the efficacy of the ketogenic diet in kindled animals—an appropriate model for partial seizures—and found the diet to have transient anticonvulsant properties (16). The investigators studied 32 male Sprague-Dawley rats, 20 of which were kindled and underwent behavioral testing; the 12 others underwent behavioral testing alone. Rats were kindled from P56 to 60 and then randomized (10 in each group) to treatment with either a ketogenic diet or regular rat chow. After-discharge threshold and seizure thresholds were tested at 1, 2, 4, and 5 weeks. Behavioral testing using both a water maze test and an open-field test was performed at week 3. During the period of administration of the ketogenic diet, statistically significant elevations of β-hydroxybutyrate were reported. Both the after-discharge thresholds and seizure thresholds were raised for the first 2 weeks of the diet; however, this effect disappeared by weeks 4 and 5. There was no difference in behavioral performance between the ketogenic diet rats and the controls (16).
Stafstrom and coworkers reported on electrophysiologic observations using hippocampal slices from rats treated with the ketogenic diet (17). They found that the ketogenic diet did not alter baseline electrophysiologic parameters in normal rats (excitatory postsynaptic potential [EPSP] slope, input and output relationship, responses to evoked stimulation, and Mg(++)-free burst frequency), but that it was associated with fewer spontaneous seizures and reduced CA1 excitability in rats made chronically epileptic by administration of kainic acid. The researchers concluded that at least part of the ketogenic diet mechanism of action might involve long-term changes in network excitability. In another experiment, rats fed the ketogenic diet after kainic acid-induced status epilepticus had significantly fewer and briefer spontaneous seizures, and less supragranular mossy fiber sprouting, compared with animals on a normal diet (18). These results provide evidence that the ketogenic diet has an antiepileptogenic effect in an experimental model.
Bough and Eagles demonstrated that the ketogenic diet increases the resistance to pentylenetetrazole-induced seizures in the rat (19). In their experiment, seizures were induced by tail-vein infusion of pentylenetetrazole in rats fed either a ketogenic diet or a normal diet for 35 days. The rats fed a ketogenic diet had a significantly increased threshold for seizure induction (p <0.01) compared with controls. These observations are particularly relevant because this model may mimic the condition of myoclonic seizure disorders in humans (19). In subsequent experiments, Bough and other collaborators performed recordings in the dentate gyrus of rats fed ketogenic caloriere-stricted (KCR), normal calorie-restricted (NCR), or normal ad libitum (NAL) diets. In vivo extracellular field responses to angular bundle stimulation were recorded. Input and output curves and paired-pulse relations were used to assess network excitability, and a maximal dentate activation (MDA) protocol was used to measure electrographic seizure threshold and duration. The animals fed the KCR diet showed greater paired-pulse inhibition,
elevated MDA threshold, and an absence of spreading depression-like events. Perhaps even more importantly, in the MDA model, the rate of increase in seizure duration after repeated stimuli was markedly reduced in the rats fed the KCR diet. These results agree with clinical observations made in the early 20th century that calorie restriction may be anticonvulsant, but they also show that the KCR diet has special properties and may, in fact be antiepileptogenic (20a).
elevated MDA threshold, and an absence of spreading depression-like events. Perhaps even more importantly, in the MDA model, the rate of increase in seizure duration after repeated stimuli was markedly reduced in the rats fed the KCR diet. These results agree with clinical observations made in the early 20th century that calorie restriction may be anticonvulsant, but they also show that the KCR diet has special properties and may, in fact be antiepileptogenic (20a).
De Vivo and colleagues reported on the change in cerebral metabolites in chronically ketotic rats (21), and found no changes in brain water content, electrolytes, and pH. As expected, fat-fed rats had significantly lower blood glucose concentrations and higher blood β-hydroxybutyrate and AcAc concentrations. More importantly, brain concentrations of adenosine triphosphate (ATP), glycogen, glucose-6-phosphate, pyruvate, lactate, β-hydroxybutyrate, citrate, α-ketoglutarate, and alanine were higher, and brain concentrations of fructose 1,6-diphosphate, aspartate, adenosine diphosphate (ADP), creatine, cyclic nucleotides, acidinsoluble CoA, and total CoA were lower in the fat-fed group. Cerebral energy reserves were significantly higher in the fat-fed rats (26.4 ± 0.6) compared with controls (23.6 ± 0.2; p <0.005). Many of these changes in metabolites could be explained by the higher energy state of the brain cells in the fat-fed group, specifically by the ratio of ATP to ADP. In addition, the normal oxaloacetate, elevated α-ketoglutarate and decreased succinyl-CoA imply maximal tricarboxylic acid (TCA) cycle activity—quite contrary to the metabolite profile observed with anesthetic-sedative agents. Pan and associates used 31P spectroscopic imaging at 4.1T to demonstrate an elevated ratio of phosphocreatine to inorganic phosphorus in patients on the ketogenic diet and concluded that there was improvement in energy metabolism with use of the diet (22).
Another possible mechanism of action may be suggested from these biochemical alterations. Elevated α-ketoglutarate may indicate increased flux through the γ-aminobutyric acid (GABA) shunt, which may, in turn, be expected to increase cerebral GABA levels. One study of adult male rats fed a ketogenic diet, however, failed to demonstrate elevated cerebral GABA levels (23).
Finally, it is worth speculating about possible GABA mimetic effects of ketosis, given the chemical structural similarities of GABA, β-hydroxybutyrate, and AcAc. Indeed, this notion was advanced in the 1930s by Helmholz and Keith, but does not appear to have been studied further to any great extent (24,25). More recent work by Likhodii and colleagues has suggested that there may be a direct anticonvulsant action of acetone. Rats were administered acetone intraperitoneally and tested in four models: maximal electroshock, subcutaneous pentylenetetrazole, amygdala kindling, and the AY-9944 test—a model of chronic atypical absence seizures. Acetone suppressed seizures in all models (26).
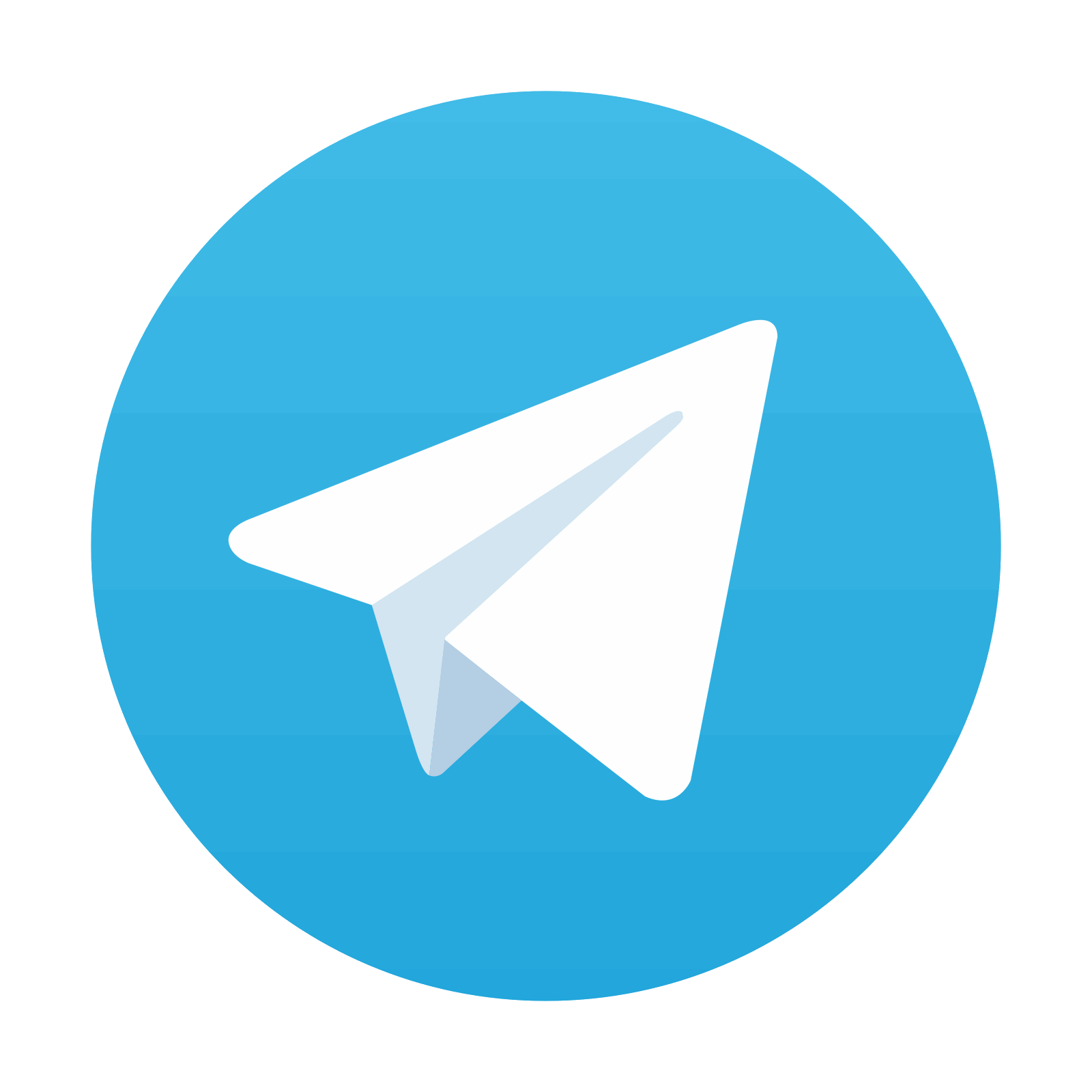
Stay updated, free articles. Join our Telegram channel

Full access? Get Clinical Tree
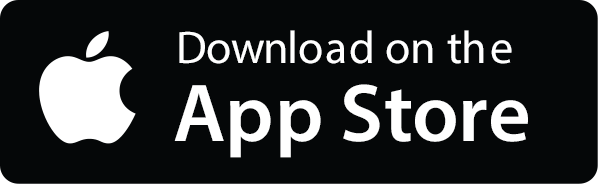
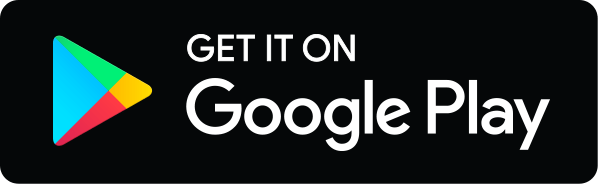