FIGURE 14.1 The extraocular muscles and the third nerve in the orbit.
FIGURE 14.2 Actions of the extraocular muscles on the left eye. Arrows denote the main directions of action for each muscle, resulting from a combination of movements of the globe in the three dimensions.
To achieve conjugate downgaze to one side, the superior oblique of the adducting eye is yoked to the inferior rectus of the abducting eye (Figure 14.3). In fact, even in adduction most of ocular elevation and depression is accomplished by the superior and inferior rectus, and the major action of both obliques is torsional (cyclotorsional, rotational). The primary action—some contend the only action—of the superior oblique is to intort (incyclotort) and of the inferior oblique to extort (excyclotort). Because of its angle of insertion along the anatomic axis, when the eye is in primary gaze the inferior rectus acts not only to depress the eye but to extort it. The yoked superior oblique, through its intorsion action, counteracts the extorsion effect of the inferior rectus so that downgaze is smooth and linear. Likewise the superior rectus and the inferior oblique.
FIGURE 14.3 The yoke muscles control extraocular movement in the six cardinal directions of gaze.
Because of the anatomical arrangement of the obliques and vertically acting recti, examination of extraocular movement should include gaze to the right and left plus upgaze and downgaze in eccentric position to both sides: the six cardinal directions of gaze (Figure 14.3). The levator palpebrae superioris supplies the striated muscles of the eyelid and elevates the lid.
THE OCULOMOTOR NERVE
The oculomotor, or third cranial nerve (CN III), arises from the oculomotor nuclear complex in the midbrain and conveys motor fibers to extraocular muscles, plus parasympathetic fibers to the pupil and ciliary body. These nuclear centers are situated in the periaqueductal gray matter just anterior to the aqueduct of sylvius, at the level of the superior colliculi (anterior quadrigeminal bodies). The median longitudinal fasciculus (MLF) abuts the nucleus laterally and ventrally (Figure 14.4). Each oculomotor nucleus consists of multiple adjacent subnuclei that innervate specific ocular muscles. The neurons are somatic motor (general somatic efferent). The paired lateral nuclei are the largest and are situated anterior and lateral to the others; their medial portions are fused into an unpaired mass. CN III has a superior and an inferior division. The superior division supplies the levator palpebrae superioris and superior rectus muscles. The inferior division supplies the medial and inferior recti, the inferior oblique, and the pupil. The lateral subnucleus innervates the ipsilateral inferior oblique and medial and inferior recti; its axons make up the inferior division of CN III. The superior rectus muscle is innervated by the contralateral medial subnucleus. Because of its crossed innervation, a major clue to the presence of a nuclear third nerve palsy is superior rectus weakness in the opposite eye.
FIGURE 14.4 Section through the mesencephalon at the level of the superior colliculus and oculomotor nucleus.
A single midline structure, the central caudal nucleus, supplies the levator palpebrae muscles on both sides. The periaqueductal gray matter is also involved with eyelid function; destructive lesions there may cause ptosis. A supraoculomotor area in the ventral periaqueductal gray matter controlling levator function has been proposed. The Edinger-Westphal (EW) nuclei are part of the craniosacral, or parasympathetic, division of the autonomic nervous system. The EW subnucleus is a single structure that provides parasympathetic innervation to both sides. It is spread throughout the length of the oculomotor complex with a paired rostral portion and an unpaired medial and caudal portion. Preganglionic fibers from the EW nuclei go to the ciliary ganglion (Figure 13.9). Postganglionic fibers derived from cells in the rostral part of the subnucleus supply the pupillary sphincter; those derived from the anteromedial nucleus supply the ciliary muscle and function in accommodation. Fibers from the medial, EW, and central caudal subnuclei form the superior division of CN III.
Fibers from the ipsilateral lateral subnucleus, from the contralateral medial subnucleus (superior rectus), and from both central caudal (levator palpebrae) and EW (parasympathetics) nuclei join to form the filaments of CN III, which course anteriorly through the mesencephalon, traversing the medial portion of the red nucleus and the substantia nigra. The nerve exits from the interpeduncular fossa on the anterior surface of the midbrain just above the pons (Figure 11.3). It travels anteriorly and passes between the superior cerebellar and posterior cerebral arteries (Figure 14.5). It runs forward parallel to the posterior communicating artery. Third nerve palsy is a classic and important sign of posterior communicating aneurysm. In its course toward the cavernous sinus, it lies on the free edge of the tentorium cerebelli, medial to the temporal lobe. Here it is at risk of compression due to uncal herniation. Through the nerve’s subarachnoid course, the parasympathetic fibers lie superficially on the dorsomedial surface. The location of these fibers influences whether a third nerve palsy will or will not involve the pupil, an important differential diagnostic point. CN III penetrates the dura just lateral and anterior to the posterior clinoid processes and enters the cavernous sinus, where it lies in the upper aspect, close to the lateral wall (Figure 14.6). In the cavernous sinus, CN III has important relationships with the carotid artery, ascending pericarotid sympathetics, and CNs IV, V, and VI. CN III separates into its superior and inferior divisions in the anterior cavernous sinus, then enters the orbit through the superior orbital fissure and passes through the annulus of Zinn. It sends a short root to the ciliary ganglion, from which postganglionic fibers go as the short ciliary nerves to supply the ciliary muscle and the sphincter pupillae (Figure 14.1). The sphincter pupillae causes constriction of the pupil. Contraction of the ciliary muscle causes relaxation of the ciliary zonule, decreasing the tension on the lens capsule and allowing it to become more convex to accommodate for near vision. Pupillary constriction, convergence, and accommodation are all part of the near reflex.
FIGURE 14.5 Anatomy of the oculomotor nerve in relation to the major arteries at the base of the brain. An aneurysm arising from the posterior communicating artery is compressing and distorting the nerve.
FIGURE 14.6 Oblique section through the cavernous sinus.
THE TROCHLEAR NERVE
The trochlear, or fourth cranial nerve (CN IV), is the smallest CN. It arises from the trochlear nucleus located just anterior to the aqueduct in the gray matter of the lower mesencephalon at the level of the inferior colliculus, immediately above the pons (Figure 14.7) and caudal to the lateral nucleus of CN III but separated by a short distance. The nucleus contains somatic motor neurons. The nerve filaments curve posteriorly around the aqueduct, decussate in the anterior medullary velum, and exit through the tectum. It is the only CN to exit from the brainstem posteriorly, and because of this extra distance, CN IV has the longest intracranial course of any of the CNs. The nerve circles the brainstem, then turns and runs forward, passing between the posterior cerebral and superior cerebellar arteries, then courses along the tentorium. It penetrates the dura just behind and lateral to the posterior clinoid processes and enters the cavernous sinus in proximity to CN III. In the sinus, it is located superolaterally, below CN III but above the trigeminal branches. Leaving the cavernous sinus, it traverses the superior orbital fissure, enters the orbit, and crosses over CN III to supply the superior oblique. It does not pass through the annulus of Zinn.
FIGURE 14.7 Section through the mesencephalon at the border of the pons, showing the trochlear nerve.
CN IV terminates on the superior oblique muscle on the side opposite the nucleus of origin. In a nuclear lesion of the fourth nerve, the contralateral superior oblique muscle is weakened; in an extramedullary lesion along the course of the nerve, the ipsilateral muscle is involved.
THE ABDUCENS NERVE
The nucleus of the abducens, or sixth cranial nerve (CN VI), lies in the mid to lower pons, in the gray matter of the dorsal pontine tegmentum in the floor of the fourth ventricle, encircled by the looping fibers of the facial nerve (Figure 14.8). The nucleus is made up of somatic motor neurons. The nerve exits anteriorly at the pontomedullary junction, crosses the internal auditory artery, and then ascends the clivus in the prepontine cistern. It passes near the Gasserian ganglion, makes a sharp turn over the petrous apex, pierces the dura at the dorsum sellae, and traverses the Dorello’s canal between the posterior clinoid process and the petrous apex. The petroclinoid ligament forms the roof of the canal. The nerve enters the cavernous sinus in company with CNs III and IV, where it lies below and medial to CN III and just lateral to the internal carotid artery. CN VI is the only nerve that lies free in the lumen of the sinus; the others are in the wall (Figure 14.6). It enters the orbit through the superior orbital fissure and the annulus of Zinn to innervate the lateral rectus.
FIGURE 14.8 Section through the pons showing fibers of the abducens and facial nerves.
SUPRANUCLEAR CONTROL OF GAZE
The supranuclear mechanisms that control gaze are designed to ensure that the fovea maintains fixation on the object of interest despite movements of the object, the eyes, or the head. A saccade (Fr. “jerk”) is a quick, small-amplitude eye movement used to acquire a target. Smooth pursuit mechanisms use slower eye movements to track a target once acquired. Saccades are designed to rapidly shift gaze to the target; pursuit movements are designed to maintain foveation of a moving target. The CN VI nucleus is the final common pathway controlling horizontal eye movements. The vertical gaze centers lie in the midbrain. There are six currently recognized eye movement control systems: saccadic, smooth pursuit, vergence, fixation, optokinetic, and vestibulo-ocular reflex (VOR).
Four interconnected cortical areas are involved in the generation of saccades: the frontal eye field (FEF), which lies anterior to the motor strip in the premotor cortex in the second frontal gyrus; the supplementary eye field, which lies in the supplementary motor area; the dorsolateral prefrontal cortex, which lies anterior to the FEF in the second frontal gyrus; and the posterior eye field, which lies in the parietal lobe.
The FEF controls horizontal conjugate saccades to the opposite side. Fibers descend and decussate en route to the pontine paramedian reticular formation (PPRF) (Figure 14.9). Other fibers descend to the superior colliculus, and are then relayed to the contralateral PPRF. The FEF to the superior colliculus pathway and the posterior eye fields are likely involved in reflex saccades. The PPRF (horizontal gaze center, lateral gaze center, pontine gaze center) is a premotor area that consists of cells lying ventrolateral to the MLF from the level of the abducens nucleus extending rostrally to near the trochlear nucleus. Signals from the PPRF activate both motor neurons and interneurons in the adjacent CN VI nucleus. The CN VI motor neurons activate the ipsilateral lateral rectus, while the interneurons simultaneously send impulses up the MLF, which decussates just rostral to the PPRF and runs to the medial rectus subnucleus of the contralateral oculomotor nuclear complex in the midbrain to activate the medial rectus. The ipsilateral lateral rectus and contralateral medial rectus then contract synchronously to produce conjugate horizontal gaze. A left FEF-initiated command to look right is thus transmitted down to the right PPRF, which simultaneously influences the right sixth nerve to contract the lateral rectus and the left third nerve to contract the yoked medial rectus—both contract, according to Hering’s law, exactly the same amount.
FIGURE 14.9 Diagram of the major supranuclear gaze pathways. The saccadic pathway arises in the frontal lobe and descends to the contralateral pons. The pursuit pathway arises in the region of the parietotemporal-occipital junction (PTOJ) and descends to the ipsilateral pons. Vertical dashed line represents the midline. DLPN, dorsolateral pontine nuclei; III, nucleus of CN III; VI, nucleus of cranial nerve (CN) VI; MLF, medial longitudinal fasciculus; NPH, nucleus prepositus hypoglossi; PPRF, pontine paramedian reticular formation; PTOJ, parietotemporal-occipital junction; VN, vestibular nuclei.
The PPRF contains burst and pause neurons. The burst neurons fire a high-frequency pulse of discharges to initiate an ipsilateral saccade. The burst neurons determine saccadic velocity. The pause neurons lie in the nucleus raphe interpositus. Their tonic discharges prevent the burst cells from initiating extraneous saccades, and the tonic firing pauses just before and during saccades. Smooth pursuit signals to the PPRF come from the vestibular nuclei and the nucleus prepositus hypoglossi, which lies in the perihypoglossal nuclear complex. Step cells in the perihypoglossal nuclei control the impulses that maintain the eyes in an eccentric position following a saccade. To acquire and maintain an eccentric stationary target, the CN VI motor neurons would have a pulse of activity generated by the burst cells to create the saccade, followed by a step-up of firing generated by the step cells to maintain the new position. All movements would be conjugate and synchronous because of coordination with the fellow eye by the MLF.
The dorsolateral prefrontal cortical area may be involved in mechanisms responsible for inhibiting unwanted saccades. Antisaccades are voluntary saccades away from a target. Patients with frontal lobe disease, progressive supranuclear palsy, Parkinson’s disease, Alzheimer’s disease, and schizophrenia, when asked to look away from a visual stimulus, may be unable to inhibit a saccade toward the target (prosaccade) and are therefore unable to make an antisaccade or make it only after a prosaccade. Another system involved in saccades works through the basal ganglia. Collaterals from the FEF go to the head of the caudate and putamen, which send fibers to the ipsilateral substantia nigra. Neurons in the pars reticulata project to the superior colliculus, which then projects to the PPRF. Disturbances in this system may explain some of the abnormalities of ocular motor control that occur in basal ganglia disorders, particularly Parkinson’s disease.
The FEF to the contralateral PPRF system controls saccadic eye movements. The smooth pursuit system originates ipsilaterally in the region of the parietotemporal-occipital junction (PTOJ), and functions to maintain foveation of a moving target. The visual cortex sends information about the target to the extrastriate cortex at the PTOJ. From there, fibers descend in the internal sagittal stratum adjacent to the atrium of the lateral ventricle down to the ipsilateral dorsolateral pontine nuclei. The system then double decussates. The dorsolateral pontine nuclei project to the contralateral cerebellum. Signals from the cerebellum then activate the medial vestibular nucleus and the nucleus propositus hypoglossi, which in turn project to the contralateral PPRF. The PPRF then coordinates conjugate horizontal pursuit movements. The PTOJ also sends corticocortical fibers to the ipsilateral frontal lobe. Smooth pursuit to the right is controlled by the right occipital region. Quick refixation saccades back to the left are mediated by the right FEF, so the process of following a series of moving objects (as in optokinetic nystagmus [OKN], or railroad nystagmus) is all accomplished in the same cerebral hemisphere.
The vergence system controls the degree of convergence or divergence of the eyes, maintaining macular fixation no matter what the distance to the target. The vestibular system has a large input into the oculomotor system in order to maintain proper eye orientation in relation to head and body position. The VOR produces conjugate eye movements of equal magnitude but in the opposite direction to compensate for head movements in order to maintain foveation during motion of the head. The VOR is discussed in more detail in Chapter 17.
The pathways controlling upgaze and downgaze course in the region of the rostral midbrain, pretectum, and posterior commissure. The vertical gaze equivalent of the PPRF is the rostral interstitial nucleus of the MLF (riMLF), which lies in the midbrain near the red nucleus. The lateral portion of the riMLF is concerned with upgaze, the medial portion with downgaze. The riMLF send impulses to the nuclei of CNs III and IV. Connections via the posterior commissure coordinate the activity on the two sides. The interstitial nucleus of Cajal (INC) lies caudal to the riMLF. Its neurons connect to the riMLF and are involved in vertical pursuit and gaze holding. Upgaze and downgaze pathways occupy different positions, and abnormalities may affect one without the other. The upgaze centers lie more dorsally. Lesions in the region of the posterior commissure may disturb vertical gaze, especially upgaze (Parinaud’s syndrome). The downgaze centers lie more ventrally, and lesions there may primarily affect downgaze.
Bhidayasiri et al. developed a hypothetical scheme to account for clinical disorders of vertical gaze based on recent insights gained from experimental studies. Vertical saccades are generated by burst neurons in the riMLF, with unilateral innervation of depressor muscles but bilateral innervation of elevator muscles. The riMLF is also a torsional saccade generator. Torsional deviation during an attempted vertical saccade, together with a vertical gaze palsy, occurs with lesions involving the riMLF. The INC acts similar to the step neurons in the PPRF, holding the eye in the new position after a vertical saccade. The INC projects to ocular motoneurons via the posterior commissure. Bilateral INC or posterior commissure lesions cause defects of vertical gaze.
Reflex upgaze occurs with forceful eyelid closure (Bell’s phenomenon), and in some conditions, reflex upgaze may be preserved when upgaze is otherwise paralyzed. Levator palpebrae and superior rectus muscle tone is normally matched. In extreme downgaze, both are maximally inhibited, but in reflex upgaze, the normal parallel innervation becomes reversed.
THE MEDIAL LONGITUDINAL FASCICULUS
The oculomotor, trochlear, and abducens nuclei make up one more or less continuous cell column. They are united for coordinated and conjugate action by the MLF, an extensive and prominent fiber tract that runs in the midline in the dorsal tegmentum of the brainstem. The MLF extends from the midbrain down to the upper thoracic spinal cord. It has many connections. Its primary function is to coordinate lateral gaze by connecting the sixth nerve nucleus on one side with the third and fourth nerve nuclei on the opposite side in order to allow the two eyes to move synchronously. Signals from the PPRF activate interneurons in the adjacent sixth nerve nucleus, which send axons up the MLF. The MLF crosses in the pons, soon after beginning its ascent to the contralateral third nerve complex. Lesions of the MLF disrupt communication between the two nuclei, causing internuclear ophthalmoplegia (INO). The MLF also has extensive connections with CNs V, VII, VIII, XI, and XII, and with the motor nuclei of the upper cervical nerves. Nuclear groups in the rostral midbrain are involved in MLF function, including the nucleus of the posterior commissure (nucleus of Darkshevich), INC (the nucleus of the MLF), and the riMLF. These connections coordinate movement of the two eyes, as well as head and eye, and even body movements. The MLF mediates reflex head and eye movements in response to various stimuli and is important in auditory-ocular, vestibular-ocular, and righting reflexes.
Sympathetic Innervation
The sympathetic pathway to the eye begins in the hypothalamus. Fibers of the first order neuron descend through the brainstem and upper cervical spinal cord. The second order neuron lies in the intermediolateral gray column at C8–T2 of the upper thoracic spinal cord (ciliospinal center of Budge). Axons exit through the anterior roots and transverse the gray rami communicantes, and then arch over the apex of the lung and beneath the subclavian artery to enter the cervical sympathetic chain, where they ascend to synapse on the third order neuron in the superior cervical ganglion at the level of the carotid bifurcation.
Postganglionic fibers of the third order neuron lie on the wall of the common carotid artery, forming the pericarotid sympathetic plexus. Sympathetic fibers innervating facial structures follow the external carotid at the bifurcation. Sympathetic fibers destined for the eye follow the internal carotid artery. A lesion proximal to the carotid bifurcation causes Horner’s syndrome (ptosis, miosis, and anhidrosis); and a lesion distal to the bifurcation causes oculosympathetic paresis (Horner’s syndrome minus facial anhidrosis). The effects on the eye of oculosympathetic paresis and Horner’s syndrome are the same and the terms are often used interchangeably; subsequent discussion refers to Horner’s syndrome. The pericarotid sympathetic plexus continues along the internal carotid artery in its course through the cavernous sinus. Sympathetic fibers migrate to CN VI for a short distance, then join the nasociliary branch of CN V1 and enter the orbit through the superior orbital fissure. They continue as long ciliary nerves to the pupillodilator muscle (Figure 14.10).
FIGURE 14.10 The cervical portion of the sympathetic division of the autonomic nervous system.
Sympathetically innervated smooth muscle is present in both the upper and lower lids to serve as accessory retractors. The upper lid muscle is better organized and identified, and it is referred to as the accessory levator palpebrae superioris, superior tarsal muscle, or Müller’s muscle. The inferior tarsal muscle in the lower lid is less distinct.
CLINICAL EXAMINATION AND DISORDERS OF FUNCTION OF THE OCULAR MOTOR NERVES AND THE CERVICAL SYMPATHETIC SYSTEM
Examination of the eyes begins with inspection—looking for any obvious ocular malalignment, abnormal lid position, or abnormalities of the position of the globe within the orbit. Abnormalities of the external eye may occasionally be of diagnostic significance in neurologic patients. Tortuous (“corkscrew”) blood vessels in the conjunctiva occur with carotid cavernous fistula, or there may be jaundice, evidence of iritis, Kayser-Fleischer rings, chemosis, dysmorphic changes (e.g., epicanthal folds), xanthelasma due to hypercholesterolemia, keratoconjunctivitis sicca, premature cataract, or ocular complications of upper facial paralysis. Basal skull fractures often cause bilateral periorbital ecchymosis (raccoon eyes). Examples of disorders with external eye and neurologic abnormalities include Gaucher’s disease, Wilson’s disease, Sjögren’s syndrome, head trauma, sarcoidosis, thyroid disease, orbital mass lesions, cavernous sinus disease, congenital syphilis, Cogan’s syndrome, Down’s syndrome, and fetal alcohol syndrome.
Exophthalmos and Enophthalmos
The globe may be abnormally positioned within the orbit so that it protrudes (exophthalmos, proptosis) or recedes (enophthalmos). Subtle proptosis can often be better appreciated by looking down at both eyes from above the vertex of the head, or by comparing side views. Exophthalmos is usually bilateral and most commonly due to thyroid eye disease (TED, Graves’ ophthalmopathy, Graves’ orbitopathy). Other conditions associated with neurologic complications and exophthalmos include the craniosynostosis syndromes and Hand-Schuller-Christian disease. While TED can occasionally cause unilateral exophthalmos, the likelihood of other conditions increases in this situation. Orbital pseudotumor is an idiopathic inflammatory condition that affects the tissues of the orbit. It is common, and second only to TED as a cause of unilateral proptosis. Some of the neurologically significant causes of unilateral proptosis include orbital mass lesion, carotid cavernous fistula, cavernous sinus thrombosis, sphenoid wing meningioma, meningocele, and mucormycosis.
Other causes of exophthalmos include orbital neoplasm and vascular malformation of the orbit. Intraorbital varicosities may cause intermittent, positional exophthalmos due to decreased venous return in certain head postures. Pulsatile proptosis may occur with an orbital vascular malformation or when there is a defect in the orbital roof that transmits cerebral pulsations, which can occur in neurofibromatosis. Diplopia can occur in conditions where the movement of the eyeball is restricted. Pseudo-proptosis is the appearance of proptosis in the absence of any orbital disease; it may occur with lid retraction or high myopia.
Few conditions of neurologic interest cause enophthalmos. Horner’s syndrome causes apparent enophthalmos because the upper lid is ptotic and the lower lid elevated due to hypotonicity of the accessory eyelid retractors. This may cause the eye to appear sunken in the orbit, but it is an optical illusion and the actual globe position is normal.
The Eyelids
Patients may couch the complaint of ptosis (blepharoptosis) in ways other than droopy eyelid (e.g., eye has shrunk). Fluctuating ptosis may suggest myasthenia gravis (MG), although many varieties of ptosis, as in Horner’s syndrome, may get worse when the patient is fatigued. Ptosis may have been present for a very long time before coming to the patient’s attention. Looking at old photographs is often helpful. The eyes on a driver’s license photo can be seen quite well by using the ophthalmoscope on magnification.
The position of the eyelids is important and can reveal significant information. Note the position of the eyelids and the width of the palpebral fissures bilaterally. The width of the palpebral fissures should be equal on both sides, although a slight difference occurs in many normal individuals. Any asymmetry of lid position should be noted, such as ptosis or lid retraction. Note the amount of iris or pupil covered by the lid. Patients may compensate for ptosis by contracting the frontalis muscle. If the examiner fixes the frontalis muscle with her finger, the patient may be unable to raise the eyelid. Ptosis may cause an artefactual superior altitudinal visual field defect that disappears when the eyelid is raised.
The normal upper eyelid in primary position crosses the iris between the limbus (junction of the iris and sclera) and the pupil, usually 1 to 2 mm below the limbus; the lower lid touches or crosses slightly above the limbus. Normally there is no sclera showing above the iris. The palpebral fissures are normally 9 to 12 mm from upper to lower lid margin. Measurement can also be made from the lid margin to the corneal light reflex. The upper lid margin is normally 3 to 4 mm above the light reflex. Levator function can be assessed by measuring the upper lid excursion from full downgaze to full upgaze just to the point where the frontalis begins to contract. This excursion is typically 10 to 12 mm. Upper lid excursion of 4 mm or less indicates poor levator function; 8 mm or more indicates good function.
With ptosis, the lid droops down and may cross at the upper margin of the pupil, or cover the pupil partially or totally. With complete ptosis, the eyelid is down and the eye appears closed (Figure 14.11). Patients with ptosis often display telltale wrinkling of the ipsilateral forehead as they attempt to hold the eye open using the frontalis muscle. Ptosis may be unilateral or bilateral, partial or complete, and occurs in many neurologic conditions (Figure 14.12). With eyelid retraction, the upper lid pulls back and frequently exposes a thin crescent of sclera between the upper limbus and the lower lid margin. Lid retraction is a classic sign of thyroid disease, but occurs in neurologic disorders as well.
FIGURE 14.11 Paralysis of the left oculomotor nerve in a patient with an aneurysm of the left internal carotid artery. A. Only ptosis can be seen. B. On elevating the eyelid, it is seen that the pupil is dilated and the eyeball is deviated laterally.
FIGURE 14.12 Characteristics of different causes of abnormal lid position. A. Right third CN palsy with complete ptosis. B. Left Horner’s syndrome with drooping of upper lid and slight elevation of lower lid. C. Bilateral, asymmetric ptosis in myasthenia gravis (MG). D. Right lid retraction in thyroid eye disease. E. Bilateral lid retraction with a lesion in the region of the posterior commissure (Collier’s sign).
The width of the palpebral fissures is normally equal on the two sides. Sometimes inequality results from subtle lid retraction or a widened palpebral fissure on one side, not to be confused with ptosis on the other side. When in doubt, measure the width of the palpebral fissures with a ruler, in both primary position and in upgaze. In addition to observing the lid position at rest, notice the relationships of the lid to the globe during eye movement. CN VII, via contraction of the orbicularis oculi, closes the eye. Facial weakness never causes ptosis. In fact, the palpebral fissure on the weak side is often wider than normal, and unilateral widening of one palpebral fissure may be an early sign of facial palsy.
Total unilateral ptosis only occurs with complete third nerve palsy. Mild to moderate unilateral ptosis occurs as part of Horner’s syndrome, or with partial third nerve palsy. Ptosis may rarely be the only manifestation of an oculomotor nerve palsy. Mild to moderate bilateral ptosis occurs in some neuromuscular disorders, such as MG, muscular dystrophy, or ocular myopathy. The ptosis in MG is frequently asymmetric and may be unilateral, though it will tend to shift from side to side (Figure 14.13). It characteristically fluctuates from moment to moment and is worsened by prolonged upgaze (fatigable ptosis). Cogan’s lid twitch sign, characteristic of myasthenia, consists of a brief overshoot twitch of lid retraction following sudden return of the eyes to primary position after a period of downgaze. A similar upward twitch may occur on glancing quickly to the side from primary position (eyelid hopping). When the ptosis is asymmetric, the driving discharges attempting to keep the more ptotic eyelid open are also transmitted, per Hering’s law, to the less ptotic eyelid. Manually raising the more ptotic lid causes relaxation and the eye with less ptosis, sometimes even no ptosis, may suddenly crash (curtain sign, seesaw ptosis). Because of the law of equal innervation, compensation for mild ptosis on one side may cause the involved eye to appear normal and the other eye to have lid retraction. Ptosis in MG may be dramatically responsive to edrophonium (Figure 14.14).
FIGURE 14.13 Bilateral ptosis in a patient with MG.
FIGURE 14.14 Patient with MG. A,B. Ptosis and paresis of upward gaze. C. Absence of ptosis and normal upward gaze after administration of neostigmine.
Cerebral ptosis is due to supranuclear lesions. Unilateral cerebral ptosis occurs with lesions, usually ischemic, of the opposite hemisphere, and is more common with right hemisphere lesions. Bilateral supranuclear ptosis may occur with unilateral or bilateral hemispheric lesions. Ptosis has been reported in as many as 37.5% of patients with hemispheric strokes. Because of the anatomy of the central caudal nucleus, bilateral ptosis can occur as the only ocular motility abnormality with some midbrain lesions.
Senile or involutional ptosis is very common. Asymmetric lids and redundant lid tissue are more the rule than the exception in the elderly. The levator aponeurosis attaches the levator muscle to the tarsal plate, which forms the eyelid. Aging may cause levator dehiscence-disinsertion (LDD)—with stretching, thinning, or detachment of the aponeurosis. Normally, with the eyelids gently closed, the upper lid margin lies 5 to 7 mm below the upper lid fold (the skin fold at the upper part of the lid). An increase in this distance suggests LDD (Figure 14.15). The lid excursion is normal, usually 9 mm or more. Trauma to the eyelid, as from contact lenses, may cause LDD in younger patients. Blepharochalasis (dermatochalasis) refers to age-related lax, baggy skin around the eyelids; it can also simulate ptosis but levator function is normal. Other non-neurologic conditions that may be confused with ptosis include blepharitis, lid edema, lid infiltration, and eyelid tumor. Patients with neurofibromatosis may have diffuse neurofibroma in the eyelid, causing a characteristic S-shaped lid. Chronic use of steroid eye drops can cause ptosis that has been attributed to a focal steroid myopathy.
FIGURE 14.15 Levator dehiscence disinsertion on the left. The distance between the upper lid margin and the upper lid crease is increased compared to the normal right side.
Congenital ptosis is common; because of levator fibrosis it may be associated with lid lag in down-gaze that is unusual in acquired ptosis. Jaw winking (Marcus Gunn phenomenon, for R. Marcus Gunn, Scottish ophthalmologist) occurs when there is congenital ptosis with abnormal communication between CN V and the levator palpebrae. The ptotic lid opens with jaw movement (see Chapter 15). A number of videos have been posted on the Internet of jaw-winking; an especially dramatic example is http://www.youtube.com/watch?v=jjvcNDimBc4. Synkinesia between CN V and the levator more rarely causes ptosis on mouth opening (inverse Marcus Gunn phenomenon). Marin Amat syndrome is a facial nerve aberrant innervation syndrome with levator inhibition with mouth opening. Orbicularis oculi contraction causing eye closure on smiling is another common synkinesia. Eyelid myotonia may cause transient difficulty opening the eyes after a forceful contraction or transient lid retraction after looking up. Ptosis in Lambert-Eaton syndrome may temporarily improve after a brief period of upgaze. Blepharospasm is a focal dystonia causing involuntary eye closure; levator function is normal. In apraxia of lid opening, the patient has difficulty in voluntarily initiating lid elevation although there is no levator impairment or blepharospasm. A fine tremor of the lid (Rosenbach’s sign) may occur in hyperthyroidism.
Pseudoptosis is the appearance of ptosis in the absence of levator abnormality. A narrow palpebral fissure can occur because of mechanical limitation of levator excursion or enophthalmos. In vertical strabismus with the hypertropic eye fixing, the lid of the hypotropic eye may seem to be ptotic, but is not. In Duane’s syndrome, the palpebral fissure narrows on ocular adduction because of globe retraction causing dynamic enophthalmos. The eyelids may also be intrinsically abnormal because of inflammation, trauma, or other factors, and these lid disorders may be mistaken for ptosis. Nonorganic ptosis is rare; it can occur because of voluntary unilateral blepharospasm. A telltale clue is that there is contraction of the orbicularis oculi or relaxation of the eyebrow elevators causing brow ptosis in addition to the appearance of lid ptosis.
Lid Retraction
Lid position is abnormal if there is a rim of sclera showing above the limbus, indicating either lid retraction or lid lag. TED is a common cause of lid abnormalities, including lid retraction in primary gaze (Dalrymple’s sign), infrequent blinking (Stellwag’s sign), and lid lag in downgaze (von Graefe’s sign). Lid retraction in primary gaze also occurs with lesions involving the posterior commissure (Collier’s sign, posterior fossa stare; see discussion of Parinaud’s syndrome). Lid retraction with posterior commissure lesions is bilateral, but may be asymmetric. With Collier’s sign, the levators relax appropriately and the lids usually descend normally on downgaze without lagging behind as they do in TED. In addition, the lid retraction may worsen with attempted upgaze (Figure 14.16). Circumscribed midbrain lesions may cause eyelid retraction with minimal impairment of vertical gaze. In Parkinson’s disease, there is infrequent blinking and there may be some lid retraction.
FIGURE 14.16 Paresis of upward gaze in a patient with a neoplasm of the posterior third ventricle.
Topical instillation of sympathomimetic agents may cause lid retraction. Very weak sympathomimetics (e.g., tetrahydrozoline), may cause the lid with denervation supersensitivity, as in Horner’s syndrome, to elevate. Apraclonidine may also improve the ptosis (see below). In vertical strabismus, the lid of the hypertropic eye may appear to be retracted when the hypotropic eye is fixing. Aberrant regeneration of CN III may cause lid retraction on adduction (the opposite of Duane’s syndrome). Lid retraction may also be mechanical, due to trauma or surgery. The plus minus lid syndrome is ptosis on one side and lid retraction on the other due to a unilateral lesion of the third nerve nucleus extending rostrally to involve the region of the posterior commissure. Lid retraction may be confused with ipsilateral proptosis or contralateral ptosis.
The Pupils
The function of the pupil is to control the amount of light entering the eye, ensuring optimal vision for the lighting conditions. The pupils should be equal in size, round, regular, centered in the iris, and should exhibit specific reflex responses. Large, small, and abnormally reactive pupils are discussed in this section. Normal pupils may display constant, small amplitude fluctuations in size under constant illumination (hippus, pupillary play, pupillary unrest, pupillary athetosis). Hippus is of no clinical significance, even when pronounced, but can cause confusion in the evaluation of an afferent pupillary defect (APD) (see discussion of afferent pupillary defect).
Size
Pupillary size depends primarily on the balance between sympathetic and parasympathetic innervation and the level of ambient illumination. The most important determinants are the level of illumination and the point at which the eyes are focused. Accurate measurements are important. Measurements should be made with a pupil gauge or a millimeter ruler; estimates are surprisingly inaccurate. Special cameras can measure the pupil to within 0.1 mm. The size of the pupils should be determined at distance in ambient and dim light and at near. The normal pupil is 2 to 6 mm in diameter. In ordinary ambient light, the pupils are usually 3 to 4 mm in diameter. The pupils are small and poorly reactive at birth and in early infancy, becoming normal size around ages 7 to 8. They are normally larger in adolescents and young adults, about 4 mm in diameter and perfectly round. In middle age, they are typically 3.5 mm in diameter and regular, and in old age 3 mm or less and often slightly irregular.
Pupils less than 2 mm in diameter are miotic. Common causes of acquired miosis include old age, hyperopia, alcohol abuse, and drug effects. Neurologically significant causes of miosis include neurosyphilis, diabetes, levodopa therapy, and Horner’s syndrome. Acute, severe brainstem lesions, such as pontine hematoma, may cause bilaterally tiny, “pinpoint” pupils that still react. Primary ophthalmologic conditions commonly cause miosis when there is external eye disease. Spastic or irritative miosis is due to spasm of the pupillary sphincter, often in association with corneal or intraocular foreign bodies or eye trauma. Other ophthalmologic disorders causing miosis include iridocyclitis, miotic drops, spasm of the near reflex, chronic anterior segment ischemia, and an old Adie’s pupil. Synechia are adhesions that may develop in the eye, usually after inflammation such as iritis. The scarring may bind the pupil down to the cornea (anterior synechia) or lens (posterior synechia) and cause miosis and pupillary irregularity. Paralytic miosis is due to paralysis of the pupillary dilator muscle (oculosympathetic paresis).
Pupils more than 6 mm in diameter are dilated. Common causes of bilateral mydriasis include anxiety, fear, pain, myopia, and drug effects—especially anticholinergics. Large pupils were once considered a sign of youth and beauty, and the anticholinergic belladonna (Ital. “fair lady”) alkaloids were named for their ability to produce this effect. Persons with light irises have larger pupils than those with dark irises. Only severe, bilateral lesions of the retina or anterior visual pathways, enough to cause near blindness, will affect the resting pupil size. Neurologically significant bilateral mydriasis occurs in midbrain lesions, in comatose patients following cardiac arrest, in cerebral anoxia, and as a terminal condition.
Shape
The normal pupil is round, with a smooth, regular outline. Gross abnormalities in shape are usually the result of ocular disease such as iritis or eye surgery. Synechia, a congenital coloboma (a gap in the iris), prior trauma, or iridectomy may all cause pupil irregularity. A slight change in shape, however, such as an oval pupil, slight irregularity in outline, serration of the border, or slight notching, may be significant in the diagnosis of neurologic disease.
Equality
The pupils are generally of equal size. A difference of 0.25 mm in pupil size is noticeable, and a difference of 2 mm is considered significant. Physiologic anisocoria (aniso, “unequal”; cor, “pupil”), mild degrees of inequality with less than 1 mm of difference between the two sides, occurs in 15% to 20% of normal individuals. With such physiologic anisocoria, the degree of inequality remains about the same in light and dark, and the pupils react normally to all stimuli and to instilled drugs (Figures 14.17 and 14.18). Normally, the pupil of the abducting eye may dilate and the adducting eye constrict with extreme lateral gaze (Tournay’s pupillary phenomenon), causing transient physiologic anisocoria. Unequal pupils may be caused by primary eye disorders, such as iritis. Unilateral mydriasis is never due to isolated, unilateral visual loss. The reactivity of the normal eye and the consensual light reflex will ensure pupil size remains equal. Unilateral mydriasis can occur with local ocular trauma (traumatic iridoplegia).
FIGURE 14.17 Behavior of unequal pupils in light and dark conditions.
FIGURE 14.18 Flow diagram for the evaluation of anisocoria.
Position
The pupil is normally situated in the center of the iris. An eccentric pupil (corectopia) usually signifies local eye disease, but it can occur with neurologic disease, especially disorders of the midbrain.
The Pupillary Reflexes
The principal pupillary reflex responses assessed on examination are the light response and the near response (“accommodation”). The normal pupil constricts promptly in response to light. Pupillary constriction also occurs as part of the near response, along with convergence and rounding up of the lens for efficient near vision. Normally, the light and near responses are of the same magnitude.
The Light Reflex
The pupillary light reaction is mediated by the macula, optic nerve, chiasm, and optic tract. Before reaching the lateral geniculate body, pupil afferents leave the optic tract to synapse in the pretectum. In addition to the decussation of nasal hemiretinal pupillary afferents, extensive crossing occurs through the posterior commissure with pupillary afferents synapsing both ipsilaterally and contralaterally. Because of the decussation in the chiasm and the decussation in the posterior commissure, pupillary fibers are extensively commingled and the reflex is bilateral, both direct and consensual (crossed) (Figure 13.9). Fibers project from the pretectum to the EW subnucleus of the oculomotor nuclear complex in the midbrain. Parasympathetic pupillary efferents from the EW subnucleus enter the third nerve and travel through the cavernous sinus and along the inferior branch of III in the orbit to innervate the pupilloconstrictor muscle of the iris. The pupillomotor fibers travel to the ciliary ganglion, then through the short posterior ciliary nerves between the sclera and the choroid to the pupillary sphincter. The sphincter is concentrically arranged; the pupillodilator muscle is radially arranged. Balancing parasympathetic input from the EW subnucleus is sympathetic input ascending from the superior cervical ganglion.
The light reflex should be tested in each eye individually. The examining light should be shone into the eye obliquely with the patient fixing at distance to avoid eliciting a confounding near response. A common error in pupil examination is to have the patient fixing at near, as by instructing her to look at the examiner’s nose. This technique provides both a light stimulus and a near stimulus simultaneously, and the pupils may well constrict to the near target of the examiner’s nose even when the reaction to light is impaired or absent. Using this technique, the examiner would invariably miss light-near dissociation. Always have the patient fix at a distance when checking the pupillary light reaction. Another technique is to use ambient light by covering the eyes, then withdrawing the cover alternately and looking for pupil constriction. The normal pupillary light reflex is brisk constriction followed by slight dilatation back to an intermediate state (pupillary escape). Escape may occur because of adaptation of the visual system to the level of illumination. The responses may be noted as prompt, sluggish, or absent, graded from 0 to 4+, or measured and recorded numerically (e.g., 4 mm → 2 mm.) In comatose patients, it is often important, but difficult, to see if the pupillary light reaction is preserved, especially if there is a question of brain death. A useful technique is to use the ophthalmoscope: focus on the pupil with high positive magnification, dim the ophthalmoscope, and then rapidly reilluminate. Even a small residual reaction may be seen.
The Accommodation Reflex
The accommodation reflex (near response, near reflex, accommodation-convergence synkinesis, near synkinetic triad) is elicited by having the patient relax accommodation by gazing into the distance, then shifting gaze to some near object. The best near object is the patient’s own finger or thumb. The response consists of thickening of the lens (accommodation), convergence of the eyes, and miosis. The primary stimulus for accommodation is blurring. Without the near response, attempting to focus on a close object would result in blurred vision or frank diplopia. With special techniques, each component of the response can be tested separately. Routine bedside testing elicits all three components. Accommodation occurs because contraction of the ciliary muscle relaxes the zonular fibers, permitting the lens to become more convex because of its inherent elasticity. Accommodation is measured in diopters (Box 14.1).
Myopia and Hyperopia
The near point of accommodation (NPA) is the closest point at which an object can be seen clearly. The NPA lengthens distressingly with advancing presbyopia. The far point of accommodation (FPA) is the distance at which a distant image is focused on the retina with no accommodative effort. An emmetrope (person with a perfectly normal eye) has an FPA of infinity, and distant objects are properly focused on the fovea. In hyperopia (farsightedness, hypermetropia) the eyeball is too short, the FPA is behind the eye, accommodation can bring far objects into focus but may fail with near objects, and the correction is with positive lenses to add refractive power. In myopia (nearsightedness), the eyeball is too long or refractive power is excessive, the FPA falls in front of the fovea, relaxation of accommodation can bring near objects into focus but fails for distant objects, and the correction is with negative lenses to reduce refractive power. To quickly tell if a patient who uses correction has hyperopia or myopia, use the patient’s glasses to look at some object about arm’s length away. Hyperopic glasses magnify, myopic lenses minify.
The convergence movement of the near reflex is mediated by the vergence subcomponent of the supranuclear ocular motor control system. It consists of a long latency, slow dysconjugate eye movement to foveate the near object by contracting both medial rectus muscles. Convergence spasm causes excessive convergence for the distance of the object, and the patient cannot focus at distance. Convergence insufficiency causes inadequate convergence, and the patient cannot focus at near.
The pupils constrict at near to increase the depth of focus. Miosis accompanies accommodation even when convergence is prevented by prisms, and it accompanies convergence even when accommodation is prevented by atropine. The pathways are less certain than for the pupillary light reflex, but they involve the visual cortex with impulses probably descending through the corticotectal tract to near response cells in the pretectum. The midbrain mechanisms for pupillary constriction to near are separate from those for the light reflex; one response may be abnormal while the other is preserved.
Other Pupillary Reflexes
The ciliospinal reflex consists of dilation of the pupil on painful stimulation of the skin of the ipsilateral neck. Local cutaneous stimulation (e.g., scratching the neck), activates sympathetics through connections with the ciliospinal center at C8–T2 that cause the ipsilateral pupil to dilate. An intact ciliospinal reflex is evidence of brainstem integrity when evaluating a comatose patient. Efferent impulses are relayed through the cervical ciliospinal center and the sympathetic nervous system. The response is minimal and may be difficult to see even when normal. A similar response may follow painful pressure on the cheek. The oculosensory or oculopupillary reflex consists of either constriction of the pupil or dilation followed by constriction in response to painful stimulation of the eye or its adnexa. The pupils normally constrict on attempted lid closure (Piltz-Westphal reaction). Dilation or constriction followed by dilation occurs in response to a loud noise (cochleopupillary reflex) or stimulation of the labyrinthine system (vestibulopupillary reflex). The pupils may dilate in response to fear, anxiety, mental concentration, and sexual arousal because of sympathetic nervous system activity (psychic reflex).
Effects of Drugs on the Pupil
Many systemically acting as well as locally acting drugs may influence pupil size and reactivity. An abnormal pupil may fail to respond appropriately, or respond excessively because of denervation super-sensitivity. Pupil pharmacology is complex. In brief, sympathomimetics and anticholinergics cause pupillary dilation, and parasympathomimetics or sympathetic blockers cause pupillary constriction. Agents that cause mydriasis include the anticholinergics atropine, homatropine, and scopolamine and the sympathomimetics epinephrine, norepinephrine, phenylephrine, hydroxyamphetamine, and cocaine. Agents that cause miosis include the cholinomimetics pilocarpine, methacholine, muscarine, and opiates, and the cholinesterase inhibitors physostigmine and neostigmine. Ergot derivatives block postganglionic adrenergic nerves and can cause pupil constriction. Pupillary pharmacology can be applied in the neurologic examination, primarily in the evaluation of Horner’s syndrome and Adie’s pupil (see Table 14.1).
TABLE 14.1 Summary of Pharmacologic Pupillary Testing for Horner’s Syndrome
DISORDERS OF THE PUPIL
Pupils can be abnormal for numerous reasons. Common problems include pupils that are too large or too small, unilaterally or bilaterally, or pupils that fail to demonstrate normal reflex responses.
Large Pupils
The two conditions most commonly causing a unilaterally large pupil are third CN palsy and Adie tonic pupil. In CN III palsy, the large pupil has impaired reactions to light and to near; abnormalities of extraocular movement and eyelid position generally betray the origin of the abnormal pupil. With total CN III palsy there is complete ptosis; lifting the eyelid reveals the eye resting in a down and out position (Figure 14.11). Although CN III palsies often affect the pupil more than other functions, some ptosis and ophthalmoparesis is usually present. Since the pupillary parasympathetics occupy a position on the dorsomedial periphery of the nerve as it exits the brainstem, compressive lesions such as aneurysms generally affect the pupil prominently. Ischemic lesions tend to affect the interior of the nerve and spare the pupil, as in diabetic third nerve palsies, because the periphery of the nerve has a better vascular supply. This rule is not absolute: pupil-sparing third nerve palsies have been reported with aneurysms (in up to 10% of cases), as have diabetic palsies involving the pupil. In Keane’s series of 1,400 patients, 53% of diabetic CN III palsies involved the pupil, but only 2% of aneurysms spared the pupil. In diabetics, pupil abnormalities were often bilateral, suggesting autonomic neuropathy. However, only rarely does a complete aneurysmal third nerve palsy spare the pupil. When the pupil is spared, some other functions are usually spared as well. Barton summarized the correct “pupil rule” as follows: complete pupil sparing with otherwise complete and isolated palsy of CN III is never due to an aneurysm. The pupil is usually involved early and prominently with third nerve compression due to uncal herniation (Hutchinson’s pupil).
When the ocular sympathetics are involved along with CN III, the pupil may be midposition because the sympathetic denervation prevents the pupil from dilating fully. This occurs most often in cavernous sinus lesions when there is compression of both CN III and the pericarotid sympathetics, leaving the pupil mid-size but unreactive. This should not be mistaken for pupil sparing. CN III palsies are sometimes complicated by aberrant reinnervation (see p. 182). This misdirection syndrome may cause abnormal pupil constriction in relation to eye movements that may mimic pupil sparing.
The patient presenting with Adie (Holmes-Adie) tonic pupil is typically a young woman who suddenly notes a unilaterally enlarged pupil, with no other symptoms. The pupillary reaction to light may appear absent, although prolonged illumination may provoke a slow constriction. The reaction to near, although slow, is better preserved. Once constricted, the tonic pupil redilates very slowly when illumination is removed or the patient looks back at distance, often causing a transient reversal of the anisocoria. The pathology in Adie pupil lies in the ciliary ganglion or short ciliary nerves, or both; its precise nature remains unknown. The parasympathetic denervation eventually leads to denervation supersensitivity; the pupil may then constrict to solutions of pilocarpine or methacholine that are too dilute to affect a normal eye. About 20% of patients develop a tonic pupil in the other eye. Adie syndrome is the association of the pupil abnormality with depressed or absent deep tendon reflexes, particularly in the lower extremities. With the passage of time, the pupil may become smaller. An old Adie pupil can be a cause of unilateral miosis. The light reaction never recovers.
The term “tectal pupils” refers to the large pupils with light near dissociation sometimes seen when lesions affect the upper midbrain. Such pupils may accompany the impaired upgaze and convergence/retraction nystagmus of Parinaud’s syndrome. The variably dilated, fixed pupils reflecting midbrain dysfunction in a comatose patient carry a bleak prognosis. Glutethimide intoxication, infamous for causing fixed pupils in drug-induced coma, has fortunately become rare. Acute angle closure glaucoma can cause severe frontotemporal headache and a dilated, poorly reactive pupil. A cloudy cornea may provide the clue that the patient does not harbor an aneurysm and needs to quickly see an ophthalmologist rather than a neurologist or a neurosurgeon. Deliberately or accidentally instilled mydriatics will produce a dilated, fixed pupil. Such pharmacologic blockade can be distinguished by the failure to respond to full strength pilocarpine, which promptly constricts a large pupil of any other etiology.
Small Pupils
The pupils in the elderly are normally smaller. Many older patients use pilocarpine eye drops to manage chronic open angle glaucoma. Many systemic drugs, such as opiates, may symmetrically shrink the pupils. Important neurologic conditions causing an abnormally small pupil include Horner’s syndrome and neurosyphilis.
HORNER’S SYNDROME
In Horner’s syndrome, sympathetic dysfunction produces ptosis, miosis, and anhidrosis. The syndrome was first described in humans by J. F. Horner (Swiss ophthalmologist), but the abnormality had been described previously in animals by Claude Bernard (and others) and it is sometimes referred to as Bernard-Horner syndrome. Lack of sympathetic input to the accessory lid retractors results in ptosis and apparent enophthalmos. The ptosis of the upper lid due to denervation of Müller muscle is only 1 to 3 mm, never as severe as with a complete CN III palsy, although it may simulate partial third nerve palsy. The ptosis can be subtle and is often missed. The lower lid is frequently elevated 1 to 2 mm because of loss of the action of the lower lid accessory retractor that holds the lid down (inverse ptosis). The resulting narrowing of the palpebral fissure causes apparent enophthalmos. Since the fibers mediating facial sweating travel up the external carotid, lesions distal to the carotid bifurcation produce no facial anhidrosis except for perhaps a small area of medial forehead that is innervated by sympathetic fibers traveling with the internal carotid.
The small pupil dilates poorly in the dark. Pupillary asymmetry greater in the dark than in the light generally means Horner’s syndrome. Recall that physiologic anisocoria produces about the same degree of pupillary asymmetry in the light and dark. In contrast, third nerve palsy and Adie pupil cause greater asymmetry in the light because of the involved pupil’s inability to constrict. Examining the eyes under light and dark conditions can help greatly in sorting out asymmetric pupils (Figures 14.17 and 14.18). Should the examiner err by having the patient fixate at near during testing, the pupillary constriction in the good eye may lessen the asymmetry and cause the abnormal pupil to be missed. The pupil in Horner’s syndrome not only dilates less fully, it dilates less rapidly. In the first few seconds after dimming the lights, the slowness of dilation of the affected pupil may cause the anisocoria to be even more pronounced (dilation lag). There is more anisocoria at 4 to 5 seconds after lights out than at 10 to 12 seconds.
The causes of Horner’s syndrome are legion and include the following: brainstem lesions (especially of the lateral medulla), cluster headache, internal carotid artery thrombosis or dissection, cavernous sinus disease, apical lung tumors, neck trauma, and other conditions (Figure 14.19). Horner’s syndrome may be an isolated manifestation of syringomyelia. The tiny and minimally reactive pupils seen commonly in pontine hemorrhage may represent acute, severe, bilateral oculosympathetic paresis. The rare condition of reverse Horner’s syndrome (Pourfour du Petit syndrome) is unilateral mydriasis, sometimes with facial flushing and hyperhidrosis, due to transient sympathetic overactivity in the early stages of a lesion involving the sympathetic pathways to one eye.
FIGURE 14.19 Left Horner’s syndrome in a patient with a pulmonary sulcus tumor.
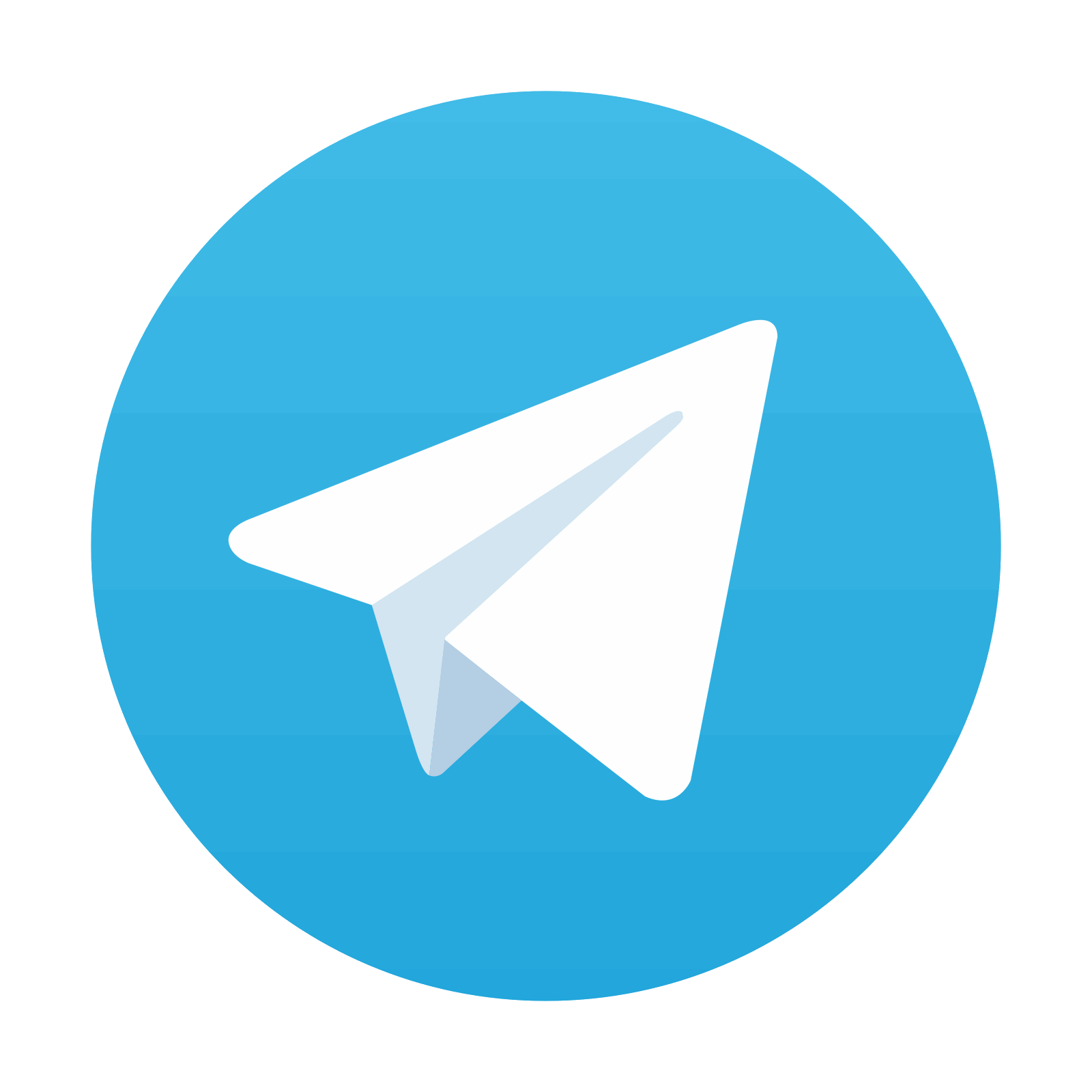