Trauma-Related Complications— Atlantooccipital Dislocation
Jason S. Weinstein
Andrew N. Nemecek
The occipitoatlantal and atlantoaxial joints comprise the bony articulations that make up the craniocervical junction. Complete disruption of the ligamentous attachments between one or both of these joints, known as traumatic atlantooccipital, atlantoaxial, or more generally craniocervical dislocation (AOD/AAD/CCD) is a frequently fatal injury following high-energy-mechanism accidents. However, improvements in prehospital management have led to an increased proportion of survivors, necessitating awareness among providers for rapid recognition and management of this highly unstable injury.
The most frequent complication associated with these injuries is delay in diagnosis. Bellabarba et al. (1) reported 17 cases of AOD/CCD from a large level 1 trauma center, of which only 4 (23%) were recognized during the initial trauma evaluation. Multiple imaging modalities are often necessary to identify these injuries. Conventional cervical spine x-rays, computed tomography (CT), magnetic resonance imaging (MRI), and dynamic studies such as the traction test may be utilized. However, given the rarity of this condition and the difficulty visualizing relevant structures, especially on plain radiographs, CCD remains a challenging diagnosis to make. Failure to diagnose CCD can lead to serious neurologic deterioration or death due to the severe instability generated and the vulnerability of the spinal cord and vascular structures. As a result, a high level of suspicion is required, as well as multiple layers of redundancy in the evaluation of patients at risk for this condition (1).
Once identified, CCD requires emergent stabilization with instrumentation and arthrodesis at the earliest safe opportunity. Modern spinal instrumentation systems utilize independent, contoured plate/screw or rod/screw implants to conform to this complex anatomic region. Serious complications of surgery are generally infrequent, but can include improper alignment, inadequate decompression of neural elements, venous sinus injury, parenchymal or spinal cord injury, vertebral artery or other vascular injury, hardware loosening or failure, cerebrospinal fluid (CSF) leak, wound infection, and graft site complications, such as iliac fracture and pain. A thorough understanding of the relevant anatomy and surgical goals can minimize these complications and result in high rates of bony fusion.
ANATOMY
The craniovertebral junction is a complex transition point between the occipital bone of the skull and the upper cervical spine. Up to 50% of all cervical flexion/extension and axial rotation occur here among the articulations between the occiput and the first two cervical vertebrae (2). Traversing the craniovertebral junction are the brainstem, spinal cord, and vertebral arteries. The critical balance between mobility and stability is reflected by the often devastating spinal cord injuries that may occur as a result of disruption of these articulations.
SKELETAL ANATOMY
The suboccipital region refers to the dorsal caudal portion of the occipital bone. The superior nuchal line marks its superior extent and approximates the level of the transverse sinuses. Though there is significant variability, the sinus is located an average of 0.9 to 2.2 mm above the superior nuchal line on the left and right, respectively; the inion corresponds to the torcular herophili, where the sagittal and transverse sinuses converge (3). In the midline, along the internal occipital crest, the suboccipital bone thickness averages 8.3 mm at the level of the inferior nuchal line and increases to an average of 13.8 mm at the inion (3). Knowledge of this thick midline keel of bone is important when considering occipital instrumentation. Likewise, an appreciation of the approximate location of the major venous sinuses will reduce potentially devastating complications related to occipital screw placement. Laterally and cranially, the bone thins out radially ranging from 3.7 inferiorly to 8.3 mm at the level of the superior nuchal line (3).
The occiput rests on the atlas via bilateral, semilunarshaped, occipital condyles. The condylar masses are wider medially than laterally when viewed from ventrally, arising from the ventrolateral occipital bone. The condyles have a
mean length, width, and height of 23.4, 10.6, and 9.2 mm, respectively. They articulate with the dorsal, concave lateral masses of C1. In children, the recess formed by this articulation is shallower; this may contribute to the higher reported incidence of traumatic atlantooccipital dislocation in pediatric patients (2). Running within the ventrolateral portion of the occipital condyle is the hypoglossal canal. The hypoglossal nerve passes through this canal at a mean distance of 12.2 mm from the dorsal margin of the condyle, along with a branch of the posterior meningeal artery (3,4).
mean length, width, and height of 23.4, 10.6, and 9.2 mm, respectively. They articulate with the dorsal, concave lateral masses of C1. In children, the recess formed by this articulation is shallower; this may contribute to the higher reported incidence of traumatic atlantooccipital dislocation in pediatric patients (2). Running within the ventrolateral portion of the occipital condyle is the hypoglossal canal. The hypoglossal nerve passes through this canal at a mean distance of 12.2 mm from the dorsal margin of the condyle, along with a branch of the posterior meningeal artery (3,4).
The atlas, or C1, is unique among vertebrae in that it does not have a vertebral body and is not associated with intervertebral disks on either its superior or inferior surfaces. The atlas is the widest of the cervical vertebrae, with a mean width of 78.6 mm (3). The two lateral masses, situated ventrolaterally in the ring of C1, are connected by a short ventral arch and a long dorsal arch. The odontoid process or dens of C2 lies just dorsal to the ventral arch, occupying what would otherwise be the expected position of the vertebral body of C1. On the inferior surface of C1 lies grooves for the vertebral artery and first cervical nerve root. The ventral surfaces of the lateral masses are slightly convex and directed medially where they articulate with the superior articular facets of the axis. The axis, or C2, is also uniquely shaped among vertebrae. The odontoid process serves as the axial pivot point for the entire craniocervical junction. Lying just dorsal to the ventral arch of C1, the dens has a ventral oval-shaped facet, which articulates with the ventral ring of C1, and a dorsal transverse groove occupied by the cruciate ligament. While osseous structures play a large role in determining the mechanical properties of the occiput-C1 segment, the mechanical properties of the C1-C2 articulations are primarily determined by ligamentous elements (Figs. 104.1 and 104.2) (3).
![]() Figure 104.1. Osseous anatomy of the suboccipital skull, C1 vertebra, C2 vertebra, and craniovertebral junction. |
LIGAMENTS OF THE CRANIOCERVICAL JUNCTION
The orientation of internal and external ligaments form the basis for complex movements, balanced by remarkable stability, at this transition point between the comparatively large skull and the diminutive cervical vertebrae. The internal ligaments provide the majority of stability and largely bypass the atlas. The atlas therefore acts as a bearing upon which the head rotates with respect to the axis.
External ligaments include the anterior and posterior atlantooccipital membranes as well as the laterally positioned atlantooccipital ligaments. The rostral extension of the anterior longitudinal ligament becomes the anterior atlantooccipital and atlantoaxial membranes. These ligaments and joint capsules are redundant and sufficiently lax to allow rotation and extension (5). Of the external ligamentous structures, only the anterior atlantooccipital membrane contributes significantly to craniocervical stability, limiting hyperextension (2). Dorsally, the ligamentum flavum is absent between the occiput and atlas as well as the atlas and axis. Superficially, the ligamentum nuchae runs from the external occipital protuberance to the spinous processes (Fig. 104.2).
The internal craniocervical ligaments lie within the spinal canal and provide the majority of stability to the complex (4). Three layers of ligaments, all lying ventral to the dura mater, run from the dorsal surface of the axis to the foramen magnum. The tectorial membrane is the rostral extension of the posterior longitudinal ligament and is well visualized on sagittal MR images (5). It lies dorsal to the remainder of the internal ligaments as well as a strong band of longitudinally oriented fibers attached to the dorsal surface of C3 as well as the bodies of the axis and dens (4). Ventral to the tectorial membrane lies the cruciate ligament. The transverse component, or transverse atlantal ligament, is 6 to 7 mm in height and secures the dens to the ventral ring of C1 (4). Triangular-shaped rostral and caudal extensions attach to the ventral surface of the foramen magnum and axis, respectively. Further ventrally lie the odontoid ligaments, referred to as the apical and alar ligaments. The paired alar ligaments are thick, funnelshaped structures that run from the dorsolateral aspect of the dens to the medial surface of the occipital condyles. The apical ligament, which runs from the tip of the dens to the foramen magnum, is a rudimentary structure, not typically visible on MR images and of unclear importance in overall stability (Figs. 104.2 and 104.3) (6).
BIOMECHANICS AND KINEMATICS
The modern perspective on craniovertebral junction biomechanics was presented by Werne in a comprehensive series of cadaver studies published in 1957 as Studies in Spontaneous Atlas Dislocation (7). In this review, he credits the initial description of occipitocervical joint mechanics to
Weber & Weber’s (1836) Die Mechanik der menschlichen Gehwerkzeuge (8). A summary of Werne’s findings demonstrates that the internal and external occipitocervical ligaments limit movements of the relatively lax joint capsules at these levels. The atlantooccipital and atlantoaxial joints each allow for approximately 20 to 30 degrees of flexion/extension in the anterior-posterior plane. Contact of the dens with the ventral foramen magnum and the transverse ligament limits flexion, while the tectorial membrane limits hyperextension (2, 3 and 4,7). Lateral flexion is limited to 5 to 10 degrees and 20 to 30 degrees at the atlantooccipital and atlantoaxial joints, respectively, with the alar ligaments primarily limiting these movements (7,9). Axial rotation of 80 to 90 degrees occurs primarily at the atlantodental and atlantoaxial joints and is constrained by the ipsilateral transverse ligament, contralateral alar ligament, and the atlantoaxial capsular ligaments (3). Vertical distraction, the most relevant mechanism to trauma-related craniovertebral distraction injuries, is resisted by the tectorial membrane, the anterior atlantooccipital membrane, the superior and inferior portions of the cruciate ligament, the apical dental ligament, and the alar ligaments; of these, the tectorial membrane and alar ligaments are the most important (3,10). Intact occipitocervical ligaments restrict both distraction and translation to less than 2 mm in any plane (3,9).
Weber & Weber’s (1836) Die Mechanik der menschlichen Gehwerkzeuge (8). A summary of Werne’s findings demonstrates that the internal and external occipitocervical ligaments limit movements of the relatively lax joint capsules at these levels. The atlantooccipital and atlantoaxial joints each allow for approximately 20 to 30 degrees of flexion/extension in the anterior-posterior plane. Contact of the dens with the ventral foramen magnum and the transverse ligament limits flexion, while the tectorial membrane limits hyperextension (2, 3 and 4,7). Lateral flexion is limited to 5 to 10 degrees and 20 to 30 degrees at the atlantooccipital and atlantoaxial joints, respectively, with the alar ligaments primarily limiting these movements (7,9). Axial rotation of 80 to 90 degrees occurs primarily at the atlantodental and atlantoaxial joints and is constrained by the ipsilateral transverse ligament, contralateral alar ligament, and the atlantoaxial capsular ligaments (3). Vertical distraction, the most relevant mechanism to trauma-related craniovertebral distraction injuries, is resisted by the tectorial membrane, the anterior atlantooccipital membrane, the superior and inferior portions of the cruciate ligament, the apical dental ligament, and the alar ligaments; of these, the tectorial membrane and alar ligaments are the most important (3,10). Intact occipitocervical ligaments restrict both distraction and translation to less than 2 mm in any plane (3,9).
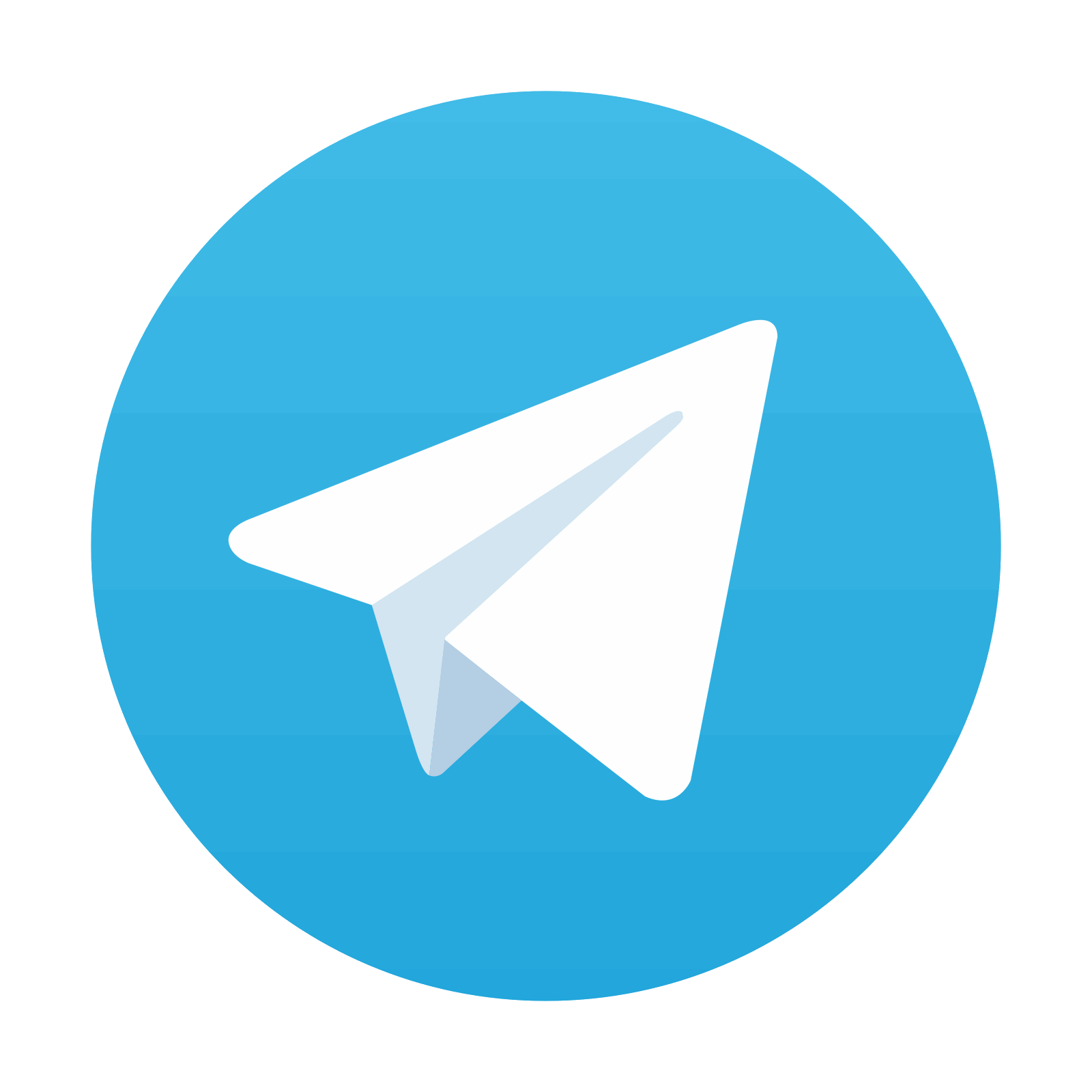
Stay updated, free articles. Join our Telegram channel

Full access? Get Clinical Tree
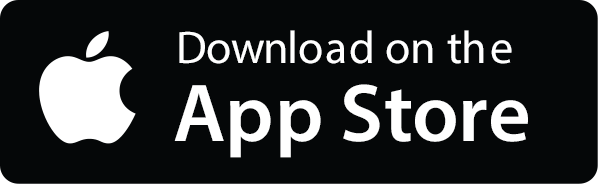
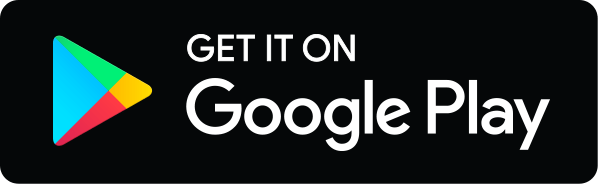