Figure 58.1. Plain (A) and contrast (B) computed tomography (CT) showing hydrocephalus and basal meningeal enhancement.
Figure 58.2. Contrast magnetic resonance imaging (MRI) showing basal meningeal enhancement.
Figure 58.3. Plain CT showing mid hydrocephalus and multiple infarcts (dots).
Figure 58.4. Contrast MRI showing basal meningeal enhancement and intra-meningeal and adjacent parenchymal tuberculomas.
58.6 Treatment of Tuberculous Meningitis
58.6.1 Antituberculous Drug Treatment
There is a scarcity of controlled trials of antituberculous drugs in CNS tuberculosis. Most of the guidelines follow the model of short-course chemotherapy of pulmonary tuberculosis: an “intensive phase” of treatment with four drugs, followed by treatment with two drugs during a prolonged “continuation phase” [12]. The Infectious Disease Society of America, the Center for Disease Control and Prevention, and the American Thoracic Society guidelines recommend an initial 2-month induction therapy with isoniazid, rifampin, pyrazinamide, and ethambutol, followed by 7 to 10 months of additional isoniazid and rifampin for an isolate that is sensitive to these drugs (Table 58.1). Recent a systemic review suggests that a 6-month regimen might be sufficient if the likelihood of drug resistance is low [40].
Drug | Blood-brain Barrier Permeability | Mechanism | Dose Children | Adult |
Isoniazi | Yes | Bactericidal (Intra-extracellular) | 10-15 mg/kg | 300 mg |
Rifampin | Yes inflamed | Bactericidal (intra-extracellular) | 10-20 mg/kg | 600 mg |
Pyrazinami | Yes | Bactericidal (intracellular) | 15-30 mg/kg | 2000 mg |
Ethambutol | Yes inflamed | Bacteriostatic | 15-20 mg/kg | 1000 mg |
Table 58.1. First-line antituberculous drugs.
58.6.2 Multi-drug Resistant Tuberculosis
Multi-drug resistant (MDR) (resistant to both isoniazid and rifampin) tuberculosis is a concern in high-burden countries. There were an estimated 500,000 cases of MDR tuberculosis in 2007, mostly from high-burden countries: 131,000 in India, 112,000 in China, 16,000 in South Africa, and 15,000 in Bangladesh. Furthermore, 55 countries had reported cases of extensively drug resistant (EDR) tuberculosis defined as MDR tuberculosis plus resistance to a fluoroquinolone and injectable second-line drugs by the end of 2008 [2]. In high-burden countries, the proportion of tuberculosis cases that are MDR may range from 1 to 14% or more [41]. Thus the probability of a patient with tuberculous meningitis in high-burden countries having MDR tuberculosis would be 0.1 to 1.4%. It will be extremely difficult clinically to suspect MRD tuberculous meningitis. MDR tuberculous therapy should be considered if there is a history of prior exposure to antituberculous drugs, contact with a patient with MDR tuberculosis, or poor clinical response to first-line drugs [13]. Resistance to isoniazid was associated with significantly longer times to CSF bacterial clearance [42]. In a prospective study of Vietnamese adults with tuberculous meningitis, isoniazid and/or streptomycin resistance was associated with slower CSF bacterial clearance but not with any difference in clinical response or outcome. However, combined isoniazid and rifampicin resistance was strongly predictive of death (RR of death 11.63, 95% CI 5.21-26.320) [43]. MDR tuberculosis requires extended treatment with second-line drugs that are less effective and have more adverse effects than isoniazid-based and rifampin-based regimens [44]. With the emergence of EDR tuberculosis, even the second-line drugs will be ineffective. Ethinomide and cycloserine have good CNS penetration and may be used as part of an “intensive phase” treatment regimen in patients with suspected MDR tuberculous meningitis [13]. Therapeutic drug monitoring has been used to assist in the management of MDR tuberculosis [45,46].
TMC207, an investigational diarylquinoline compound, acts by specifically inhibiting mycobacterial ATP synthase. In vitro, it inhibits drug-sensitive and drug-resistant M. tuberculosis isolates and is also bactericidal against dormant tubercle bacilli [47-50]. In a two-stage, phase 2, randomized, placebo-controlled trial consisting of an exploratory stage (8 weeks) followed by a separate proof-of-efficacy stage (24 weeks) in patients with newly diagnosed, smear-positive pulmonary infection caused by MDR M. tuberculosis, the addition of TMC207 to standard therapy reduced the time to conversion to a negative sputum culture as compared with placebo and increased the proportion of patients with conversion of sputum culture. The drug was well tolerated except for significant nausea [51].
The in-hospital case-fatality rate was 57% in patients with MDR tuberculous meningitis, with significant functional impairment in most of the survivors (Patel 2004) [52]. The morality was near 90% in patients with HIV-associated MDR tuberculous meningitis [53].
58.6.3 Adjunctive Steroid Therapy
A Cochrane systematic review concluded that overall adjunctive therapy with corticosteroids reduces the risk of death (RR, 0.78, 95% CI, 0.67-0.91). Data on disabling residual neurological deficits from three trials showed that corticosteroids reduce the risk of death or disabling residual neurologic deficit (RR, 0.82, 95% CI, 0.70-0.97). The review recommends the routine use of corticosteroids in HIV-negative people with tuberculous meningitis to reduce death and disabling neurological deficits amongst survivors. Corticosteroids should be used irrespective of age and disease stage [54]. A recent Vietnam adult study of adjunctive dexamethasone therapy in tuberculous meningitis demonstrated a significant reduction in mortality but not in morbidity [55]. A further subgroup analysis revealed that this benefit occurred among all severe grades of CNS tuberculosis and that this benefit was not seen in patients with HIV co-infection. The study also found that treatment with dexamethasone was associated with less severe adverse events, particularly hepatitis. The mechanism by which dexamethasone exerts its survival benefit is uncertain. Two possible mechanisms have been proposed: 1) the effect of dexamehasone on the deleterious aspects of the immune response in the CNS; and 2) the ability of dexamehtasone to prevent hydrocephalus and/or infarction [13,38]. Dexamethasone does not seem to improve outcome by attenuating immunological mediators of inflammation in the subarachnoid space or by suppressing peripheral T-cell response to mycobacterial antigens [15]. There is some evidence to suggest that adjunctive dexamethasone may affect outcome from tuberculous meningitis by reducing hydrocephalus and preventing infarction [38].
The initial dose of dexamethasone is 8 mg/day for children weighing <25 kg and 12 mg/day for children weighing ≥25 kg and for adults for 3 weeks and then decreased gradually during the following 3 weeks [56]. In the trails by Thwaites et al. [55], the initial dose was 0.3 mg/kg/day for grade I and 0.4 mg/kg/day for grades II and III, followed by gradual tapering over 6 weeks.
58.7 Treatment of Complications
In patients with tuberculous meningitis, potential neurologic complications requiring careful attention include altered mental status, elevated intracranial pressure (eICP), hydrocephalus, vasculitis, and acute seizures. Critical care of these patients should focus not only on treatment of the underlying infection and its immediate complications but also on minimizing secondary brain injury. Aggressive and appropriate care within the intensive care unit setting can minimize these complications and improve the chance of a good outcome [19].
58.7.1 Fever
Fever is a common feature in patients with tuberculous meningitis and the reported frequency varies between 60 and 95% [12]. Fever is regarded as a fundamental component of the acute-phase response to infection. Despite considerable research, it remains unclear whether infection-related fever is globally beneficial or harmful [57]. No data exist to determine the effect of fever on the pathology and ICP in tuberculous meningitis.
Fever management has become a common practice in neurologic and neurosurgical ICUs in patients with acute brain insult [58]. Fever exacerbates the degree of resulting neuronal injury in the presence of acute brain insult [58-60] and it also raises ICP [61]. However, there are several uncertainties with regard to infection-related fever management. There are very few studies that have looked into the effect of infection-related fever in patients with acute brain insult. Retrospective data indicate that stroke patients with preceding bacterial infections have poorer neurological and behavioural outcomes than do their uninfected counterparts [62,63]. In one experiment in which rats were injected with E. coli lipopolysaccharide prior to the induction of global brain hypoxia, fever was associated with increased neural damage [64]. Thus, fever management to achieve normothermia might be warranted in patients with severe grade tuberculous meningitis. However, one has to exercise caution in patients with associated sepsis. Low or normal temperature during bacteraemia has been shown to be associated with poor outcome [65]. Standard fever management consists of antipyretic drug therapy and external/physical cooling. Newer methods with surface cooling and intravascular cooling devices are more effective in lowering fever than standard fever management protocols [58].
58.7.2 Fluid and Sodium Disturbances
Disturbances of sodium, intravascular volume, and water are common in tuberculous meningitis. The reported frequency of hyponatremia in patients with tuberculous meningitis varies between 35 and 65% [66-68]. Hypotonic hyponatremia causes the entry of water into the brain, resulting in cerebral edema [69]. Hyponatremia without eICP may be associated with altered mental status. In patients with tuberculous meningitis, both hyponatremia and altered mental status are independent predictors of death or severe disability [70].
In tuberculous meningitis it is often difficult to precisely determine the cause of hyponatremia. The differential diagnosis includes the central salt wasting syndrome (CSWS), the syndrome of inappropriate secretion of antidiuretic hormone (SIADH), and adrenal insufficiency. The CSWS is defined as renal loss of sodium during intracranial disease, leading to hyponatremia, excessive natriuresis, volume depletion and clinical response to volume and salt replacement [71]. The available evidence suggests that the cause of hyponatremia in tuberculous meningitis is due to CSWS [72-77]. The CSWS involves renal salt loss resulting in hyponatremia and hypovolaemia, whereas SIADH involves a physiologically inappropriate secretion of ADH or increased renal sensitivity to ADH, leading to renal conservation of water and euvolaemic or hypervolaemic hyponatremia [71].
At presentation, many patients with tuberculous meningitis have compromised volume status. The first step is to assess the volume status and replace the volume with normal saline and simultaneously investigate the patient for hyponatremia. The therapy in CSWS is volume and sodium replacement (0.9% sodium chloride or 3% if necessary). The rapidity of sodium replacement depends on the rate at which the hyponatremia developed. Treatment of hyponatremia developing at a rate of >0.5 mmol/l/h should be aggressive, as it is a life-threatening complication and may cause death from severe cerebral edema and herniation (Kroll 1992) [78]. Mineralocorticoid or fludrocortisone supplementation has also been shown to be effective in returning serum sodium levels to normal [79,80]. Volume restriction is the treatment of choice in SIADH and in patients with symptomatic hyponatremia; 3% sodium chloride is usually combined with frusemide to facilitate free water excretion and correct hyponatremia.
58.7.3 Acute Seizures
Acute seizures occur in about 50% of children with tuberculous meningitis and in 5% of adults [12]. New-onset acute symptomatic seizures are not uncommon in patients with HIV-associated tuberculous meningitis [81]. Rarely convulsive status epilepticus (CSE) [82] and non-convulsive (NCSE) [83] status epilepticus (SE) may complicate tuberculous meningitis. Patients with the first acute symptomatic seizure caused by CNS infection are at a higher risk for 30-day mortality [84].
In patients with CNS infections, recurrent seizures are common after the first acute seizure [85]. Probably, these patients will need AED prophylaxis to prevent seizure recurrence, at least for the period of resolution or stabilization of an acute CNS insult. The plan in such patients would be acute abortive treatment with benzodiazepines, followed by a loading dose of phenytoin/fosphenytoin and subsequent maintenance therapy. An alternative drug is levetericetam. Studies using levetericetam in SE and NCSE, both in children and adults, suggest the efficacy of the drug when administered early, and a dose of <3000 mg daily is likely to provide benefits [86-88]. AEDs may be continued if there is a high risk of recurrence for a period of 3 to 6 months [89].
While using AEDs, interactions with other co-medications, particularly anituberculous drugs, should be considered. Isoniazid inhibits phenytoin, carbamazepine and valproic acid, producing high serum levels of these AEDs and thus toxicity [90,91]. Rifampin lowers the concentration of valproic acid, phenytoin, carbamazepine, lamotrigine, making some of these drugs relatively ineffective [90]. When combined with isoniazid, rifampicin counters the former’s inhibitory effect on the metabolism of phenytoin. Isoniazid, rifampin, pyrazinamide and valproic acid are all hepatotoxic drugs. When used together, they may potentiate hepatotoxicity. Preferably, valproic acid should be avoided; if given, liver function should be monitored at regular intervals.
58.7.4 Vasculitis
Vascular pathology associated with tuberculous meningitis, arteritis, arterial spasm, intraluminal thrombus, and external compression of proximal vessels by exudates in the basal cisterns, all compromise cerebral perfusion and oxygen delivery to the brain [10,11,92]. In an autopsy study, arteritis and infarcts were seen in 70% of brains. Arteritis mostly involved the perforating branches of the major arteries at the base of the brain [10,11].
It is not clear how to treat this serious complication of tuberculous meningitis or the compromised cerebral perfusion and infarction. Recently delayed abnormalities of cerebral oxygenation, despite ICP control and full conventional therapy, have been shown in two patients with tuberculous meningitis, confirming the progressive nature of the vascular insult [93]. Corticosteroids may be beneficial. How corticosteroids exert their beneficial effect in tuberculous meningitis is not clear. One of the mechanisms may be an anti-inflammatory effect. However, this effect is difficult to prove [94]. The Vietnam adult study suggests that dexamethasone might improve survival from tuberculous meningitis by reducing the incidence of infarction and speeding the resolution of hydrocephalus [38]. Corticosteroids might antagonize vascular endothelial growth factor (VEGF) β and thereby reduce vasogenic cerebral edema [95]. Gujjar et al. [96] studied the efficacy of triple-H therapy in patients with tuberculous arteritis and suggested that it is safe and may be beneficial in the management of patients with tuberculous arteritis.
58.7.5 Intracranial Pressure
In patients with tuberculous meningitis, elevated intracranial pressure (eICP) is one of the predictors of poor outcome. The relative risk of poor outcome in children with clinical features of eICP was 1.7 (95% CI, 1.7–2.2; p 0.002). In this study, the stage of the disease at admission was found to be an independent risk factor for poor outcome (odds ratio [OR], 4.8, 95% CI, 2.7-8.7; p <0.001) [21]. The presence of hydrocephalus usually signifies eICP; in patients with hydrocephalus, the stage of the disease at admission is a predictor of poor outcome [97-100].
Pathogenesis of ICP
Understanding the pathogenesis of eICP is essential for formulating appropriate therapeutic interventions. The pathological substrate of eICP includes: 1) diffuse edema consequent to encephalitic processes; 2) micro- and macroinfarcts secondary to vasculitis of both small and large vessels and the associated edema and space-occupying effect; 3) hydrocephalus due to CSF blockage by adhesion formation of the basal subarachnoid cisterns; 4) a space-occupying effect of associated tuberculoma(s) [10,11]. Rarely, mostly in children, tuberculous encephalopathy may contribute to eICP. Tuberculous encephalopathy is an immune-mediated allergic process with no appreciable inflammatory reaction; the pathology includes demyelination and edema [24].
In addition to the pathological substrate, other players in the pathogenesis of eICP in tuberculous meningitis include fever and hyponatremia. Hyponatremia is a common finding in such patients and results in osmotic water shifts, leading to an increase in intracellular fluid (ICF) volume, especially brain cell swelling or cerebral edema [69]. An increase in brain temperature in the presence of acute cerebral damage is associated with a significant raise in ICP [61].
Management
The clinical presence of papilledema may help to diagnose eICP. The GCS is a reliable scale to assess brain injury severity. A GCS <8 suggests serious pathology and possible eICP. In addition to clinical evaluation, neuroimaging is an essential component in the management of eICP in patients with tuberculous meningitis. It provides a good idea about the possible pathological substrate of eICP. The imaging evaluation usually begins with a contrast CT scan as an emergency procedure: 1) to identify intracranial lesions, tuberculomas and space-occupying infarcts that may need surgical correction; 2) to identify CSF obstruction, hydrocephalus; and 3) to appreciate the severity of cerebral edema or the presence of brain shift.
There are no established guidelines for when to institute ICP monitoring in patients with tuberculous meningitis and eICP. In one study in children with hydrocephalus and eICP, response to therapy was assessed by means of repeated lumbar CSF pressure monitoring and CT scanning. No correlation was observed between lumbar opening pressure and the degree of hydrocephalus as measured by CT [101]. Studies in children with other CNS infections, encephalitis and bacterial meningitis have shown the usefulness of ICP monitoring in the optimal management of ICP [102,103]. It will be appropriate to monitor ICP in patients with tuberculous meningitis with features of ICP and grade II and III disease.
Management of eICP should be carried out in a stepwise fashion as in any other clinical setting. The initial steps should include optimal head positioning to allow for venous drainage and adequate analgesia and sedation. Additional basic measures should focus on optimizing hemodynamic status and oxygenation (airway and ventilation control).
Osmotherapy
The administration of osmotic agents is one of the principal strategies to lower eICP. Commonly used agents are mannitol and hypertonic saline. Osmotic agents, most often mannitol, have been used in patients with CNS infections to treat eICP [104-106]. But none of the studies systematically evaluated the efficacy of the osmotic agents. Similarly, there are few studies in which eICP was treated with an ICP-targeted approach [107]. Hypertonic saline can be used as an alternative to mannitol. It may also be used in otherwise refractory intracranial hypertension to treat eICP. The safety and efficacy of hypertonic saline in the treatment of eICP in other clinical settings has been well established. However, caution is advised with high osmolar loads because they carry an increased risk for potentially deleterious consequences of hypernatremia or may induce osmotic blood-brain barrier opening, with possibly harmful extravasation of the hypertonic solution into the brain tissue [108].
Hyponatremia is a common complication of tuberculous meningitis, and hypovolaemia is often present early in the course of the disease. Fluid therapy should aim at avoiding hypovolaemia and hypo-osmolality. When such a clinical situation is associated with eICP, hypertonic saline may be an appropriate choice [19]. Hypertonic saline is devoid of the risks of dehydration and tubular damage as occur with mannitol.
Hydrocephalus
Hydrocephalus can be treated with diuretics, osmotic agents, serial lumbar puncture, external ventricular drainage or ventriculoperitoneal shunt. The addition of acetazolamide and furosemide was significantly more effective in achieving normal ICP than antituberculous drug treatment alone [109]. In a series of 217 children with tuberculous meningitis and hydrocephalus, medical treatment obviated the need for shunt surgery in over 70% of children [110]. However, patients on medical treatment should be closely monitored to detect worsening or lack of improvement, and shunt surgery should be considered if medical management fails. Ventriculoperitoneal shunt is associated with favourable outcome. The grade of the disease at presentation is a predictor of outcome after shunt surgery [97-100]. In mild and moderate hydrocephalus, early shunt surgery (2 days after diagnosis) was associated with better outcomes compared with delayed surgery (3 weeks after diagnosis [111].
Tuberculomas
The reported frequency of tuberculoma(s) on the initial CT scan varies between 2 and 38% [19]. A growing body of evidence suggests that most often tuberculomas resolve with antituberculous treatment [19].Surgical excision is indicated in: 1) tuberculomas causing obstructive hydrocephalus and significant eICP; 2) tubuerculomas causing obstructive hydrocephalus and not resolving on medical treatment; 3) large space-occupying tuberculomas with eICP; 4) tuberculomas with associated compartmental shifts and not resolving with medical treatment [19].
Prognosis
The reported mortality associated with treated tuberculous meningitis varies between 20 and 50% [13]. In a recent, large retrospective cohort study of all the children with tuberculous meningitis in the Western Cape of South Africa, the mortality was 13% [21]. This very low mortality has been explained by directly observed treatment, active treatment of hydrocephalus, and low rate of HIV co-infection and MDR tuberculosis in the study population. However, the morbidity was quite high, and only 16% of patients had a normal outcome. The morbidity reported in other series varied between 20 and 30% [13]. A major prognostic indicator for mortality and morbidity was disease stage at presentation [21,112]. In the South African study in children, ethnicity, disease stage, headache, convulsions, motor function, brainstem dysfunction, and cerebral infarction were independently associated with poor outcome on multivariate logistic regression analysis. The area under curve (AUC) of the model was 0.84 (95% CI, 0.80-0.89) [21].
58.8 Conclusion
Tuberculous meningitis is a serious CNS infection with significant mortality and high morbidity among survivors. Most factors found to correlate with poor outcome can be directly traced to the degree of disease progression at the time of diagnosis. The only way to reduce mortality and morbidity is by early diagnosis and timely recognition of complications. Aggressive and appropriate care within the intensive care unit setting can minimize associate brain injury and improve the chance of a good outcome.
References
1. Corbett EL, Watt CJ, Walker N, et al. The growing burden of tuberculosis: global trends and interactions with the HIV epidemic. Arch Intern Med 2003; 163:1009-21
2. Global tuberculosis control: epidemiology, strategy, financing, WHO report 2009 (publication No. WHO/HMT/TB/2009.411). Geneva: World Health Organization 2009
3. Selwyn PA, Haitel D, Lewis VA, et al. A prospective study of the risk of tuberculosis among intravenous drung users with human immunodefieciency virus infection. N Engl J Med 1989; 320: 345-50
4. Dye C, Scheele S, Dolin P, et al. Consensus statement. Global burden of tuberculosis: estimated incidence, prevalence, and mortality by country. WHO Global Surveillance and Monitoring Project. JAMA 1999; 282: 677-86
5. Bishburg E, Sunderam G, Reichman LB, et al. Central nervous system tuberculosis with the acquired immunodeficiency syndrome and its related complex. Ann Intern Med 2986; 205: 32-4.
6. Donald PR, Schoeman JF. Tuberculous meningitis. N Engl J Med 2004; 351; 1719-20
7. Chakraborty AK. Estimating mortality from tuberculous meningitis in a community: use of available epidemiological parmeters in the Indian context. Ind J Tub 2000; 47: 9-12
8. Thwaites GE, Duc Bang N, Huy Dung N, et al. The influence of HIV infection on clinical presentation, response to treatment and outcome in adults with tuberculous meningitis. J Infect Dis 2005; 192; 2134-41
9. van der Weert EM, Hargers NM, Schaaf HS, et al. Comparison of diagnostic criteria of tuberculous meningitis in human immunodeficiency virus-infected and uninfected children. Pediar Infect Dis J 2006; 25: 65-9
10. Dastur DK, Manghani DK, Udani PM. Pathology and pathogenetic mechanisms in neurotuberculosis. Radiol Clin North Am 1995; 33: 733-52
11. Shankar SK, Santosh V, Mahadevan A, et al. Pahtology of cerebral vasculature in neurotuberculosis-some observations. In: Mehata VS, Misra UK (eds). Progress in Neurosciences, New Delhi, Neurological Society of India, 2003; pp. 134-41
12. Thwaites GE, Hein TT. Tuberculous meningitis: many questions, too few answers. Lancet Neurol 2005; 4: 160-70
13. Rock RB, Olin M, Baker CA, et al. Central nervous system tuberculosis: pathogenesis and clinical aspects. Clin Microb Rew 2008; 21: 243-61
14. Thwaites GE, Simmons CP, Quyen NTH, et al. Pathophysiology and prognosis in Vietnamese asults with tuberculous meningitis. J Infect Dis 2003; 188: 1106-15
15. Simmons CP, Thwaites GE, Quyen NT, et al. The clinical benefit of adjunctive dexamethasone in tuberculous meningitis is not associated with measurable attenuation of peripheral or local immune responses. J Immunol 2005; 175: 579-90
16. Mastroianni CM, Paoletti F, Lichtner M, et al. Cerebrospinal fluid cytokines in patients with tuberculous meningitis. Clin Immunol Immunopathol 1997; 84: 171-6
17. Brown HC, Chau TT, Mai NT, et al. Blood-brain barrier function in cerebral malaria and CNS infections in Vietnam. Neurology 2000; 55: 104-11
18. Matsuura E, Umehara F, Hashiguchi T, et al. Marked increase of matrix metalloproteinase 9 in cerebrospinal fluid of patients with fungal or tuberculous meningoencephalitis. J Neurol Sci 2000; 173: 45-52
19. Murthy JMK. Management of intracranial pressure in tuberculous meningitis. Neurocrit Care 2005; 2: 306-12
20. Udani PM, Parekh UC, Dastur DK. Neurological and related syndromes in CNS tuberculous meningitis: clinical features and pathogenesis. J Neurol Sci 1971; 14: 341-57
21. van Well GT, Paes BF, Terwee CB, et al. Twenty years of pediatric tuberculous meningitis: a retrospective cohort study in the western cape of South Africa. Pediatrics 2009;123: e1-8
22. Katrak SM, Shembalkar PK, Rijwe SR, Bhandarkar LD. The clinical, radiological and pathological profile of tuberculous meningitis to patients with and without human immunodeficiency virus infection. J Neurol Sci 2000; 181: 118-26
23. Whiteman M, Espinoza I, Posi MD, et al. Central nervous system tuberculosis in HIV-infected patients: clinical and radiographic findings. Am J Neurorad 1995; 16: 1319-27
24. Udani PM,Dastur DK. Tuberculous encephalopaaaathy with and without meningitis: clinical features and pathological correlations. J Neurol Sci 1970; 10: 541-61
25. Alrcon F, Duenas G, Cevallos N, et al. Movement disorders in 30 patients with tuberculous meningitis. Mov Disord 2000; 15: 561-69
26. British Medical Research Council. Streptomycin treatment of tuberculous meningitis. Br Med J 1948; 1: 582-97
27. Kumar R, Singh SN, Kohli N. A diagnostic rule for tuberculous meningitis. Arch Dis Child 2999; 221-4
28. Thwaites GE, Chau TT, Stepniewska K, et al. Diagnosis of adult tuberculous meningitis by use of clinical and laboratory features. Lancet 2002; 360: 1287-92
29. Sinner SW, Tunkel AR. Approach to the diagnosis and management of tuberculous meningitis. Current Infectious Disease Reports 2002; 2: 324-31
30. Kennedy DH, Gallon RJ. Tuberculous meningitis. JAMA 1979; 241: 264-8
31. Thwaites GE, Chau TT, Farrar JJ. Improving the bacteriological diagnosis of tuberculosis meningitis. J Clin Microbiol 2004; 42: 378-9
32. Pai M, Flores LL, Pai N, et al. Diagnostic accuracy of neucleic acid amplification tests for tuberculous meningitis: a systematic review and meta-analysis. Lancet Infect Dis 2003; 3: 633-43
33. Bernaerts A, Vanhoenacker FM, Parizel PM, et al. Tuberculosis of the central nervous system: overview of neuroradiological findings. Eur Radiol 2003; 13: 1876-90
34. Kumar R, Kohli N, Thavani H, et al. Value of CT scan in the diagnosis of meningitis. Indian Pediatr 1996; 33: 465-8
35. Andronikou S, Smith B, Hatherhill M, et al. Definitive neuroradiological diagnostic feature of tuberculous meningitis in children. Pediatr Radiol 2004; 34: 876-85
36. Offenbacher H, Fazekas F, Schmidt R, et al. MRI in tuberculous meningoencephalitis: report of four cases and review of the neurimaging literature. J Neurol 1991; 238: 340-4
37. Ranjan P, Kalita J, Misra UK. Serial study of clinical and CT changes in tuberculous meningitis. Neuroradiology 2003; 45: 277-82
38. Thwaites GE, Macmullen-Price J, Chau TT, et al. Serial MRI to determine the effect of dexamethasone on the cerebral pathology of tuberculous meningitis: an observational study. Lancet Neurol 2007; 6: 230-6
39. Morgado C, Ruivo N. Imaging meningo-encephalic tuberculosis. Eur J Radiol 2005; 55: 188-92
40. van Loenhout-Rooyackers JH, Keyser A, Laheij RJ, et al. Tuberculous meningitis: is a 6-month treatment regimen sufficient? Int J Tuberc Lung Dis 2001; 5: 128-35
41. Zager EM, McNerney R. Multidrug-resistant tuberculosis. BMC Infect Dis 2008; 8:10
42. Thwaites GE, Caws M, Chau TT, et al. Comparison of conventional bacteriology with nucleic acid amplification (amplified mycobacterium direct tes) for diagnosis of tuberculous meningitis before and after inception of antituberculosis chemotherapy. J Clin Microbiol 2004; 42: 996-1002
43. Thwaites GE, Ngoc Lon TN, Dung NH, et al. Effect of antituberculosis drug resistance on response to treatment and outcome in adults with tuberculous meningitis. J Infect Dis 2005; 192: 79-88
44. Matteelli A, Migliori GB, Cirillo D, et al. Multidrug-resistant and extensively drug-resistant Mycobacterium tuberculosis: epidemiology and control. Expert Rev Anti Infect Ther 2007; 5: 857-71
45. De Vincenzo JP, Berning SE, Peloquin CA, et al. Multidrug-resistant tuberculosis meningitis: clinical problems and concentrations of second-line antituberculous medication. Ann Pharmacother 1999; 33: 1148-88
46. Pleoquin CA. Therapeutic drug monitoring in the treatment of tuberculosis. Drugs 2002; 62: 2169-83
47. Andries K, Verhasselt P, Guillemont J, et al. A diarylquinoline drug active on the ATP synthase of Mycobacterium tuberculosis. Science 2005; 307: 223-7
48. Koul A, Dendouga N, Vergauwen K, et al. Diarylquinolines target subunit c of mycobacterial ATP synthase. Nat Chem Biol 2007; 3: 323-4
49. Huitric E, Verhasselt P, Andries K, Hoffner SE. In vitro antimycobacterial spectrum of a diarylquinoline ATP synthase inhibitor. Antimicrob Agents Chemother 2007; 51: 4202-4
50. Koul A, Vranckx L, Dendouga N, et al. Diarylquinolines are bactericidal for dormant mycobacteria as a result of disturbed ATP homeostasis. J Biol Chem 2008; 283: 25273-80
51. Diacon AH, Pym A, Grobusch M, et al. The diarylquinoline TMC207 for multidrug-resistant tuberculosis. N Engl J Med 2009; 360: 2397-405
52. Patel VB, Padayatchi N, Bhigjee AI, et al. Multidrug-resistant tuberculous meningitis in Kwazulu-Natal, South Africa. Clin Infect Dis 2004; 38: 851-6
53. Daikos GL, Cleary T, Rodriguez A, Fischl MA. Multidrug-resistant tuberculous meningitis in patients with AIDS. Int J Tuberc Lung Dis 2003; 7: 394-8
54. Prasad K, Singh MB. Corticosteroids for managing tuberculous meningitis. Cochrane Database of Systematic Reviews 2008, Issue 1. Art. No. CD002244, DOI: 10.1002/14651858.CD002244.pub3
55. Thwaites GE, Nguyen DB, Nguyen HD, et al. Dexamethasone for the treatment of tuberculous meningitis. N Engl J Med 2004; 351: 1741-51
56. Grigis NL, Farid Z, Kilpatrick ME, Sultan Y, Mikhail IA. Dexamethasone adjunctive treatment for tuberculous meningitis. Pediatr Infect Dis J 1991; 10: 179-83
57. Greisman LA, Mackowiak PA. Fever: beneficial and detrimental effects of antipyretic. Current Opinion in Infectious Diseases 2002; 15: 241-5
58. Axelrod YK, Diringer MN. Temperature management in acute neurologic disorders. Crit Care Clin 2007; 22: 767-85
59. Wass CT, Lanier Wl, Hofer RE, et al. Temperature changes of > or = 1degree C alter functional neurologic outcome and histopathology in canine model of complete cerebral ischemia. Anesthesiology 1995; 83: 325-35
60. Cairns CJ, Andrews PJ. Management of hyperthermia in traumatic brain injury. Curr Opin Crit Care 2002; 8: 106-60
61. Rossi S, Roncati Zanier E, Mauri I, et al. Brain temperature, body core temperature, and intracranial pressure in acute cerebral damage. J Neurol Neurosurg Pscybiatry 2001; 71: 448-54
62. Syrjanen J, Valtonen VV, Iivanainen J, et al. Preceding infection as important risk factor for ischemic brain infarction in young and middle aged patients. Br J Med 1988; 296: 1156-60
63. Oppenheimer S, Hachinski V. Complications of acute stroke. Lancet 1992; 339: 721-4
64. Thornhill J, Asselin J. Increased neural damage of global hemispheric hypoxic ischemia (GHHI) in febrile but nor nonfebrile lipopolysaccharide Escherichia coli injected rats. Can J Physiol Pharmacol 1988; 76: 1009-16
65. Bryant RE, Hood AF, Hood CE, et al. Factors affecting mortality of gram-negative rod bacteremia. Arch Intern Med 1971; 127: 120-8
66. Christopher R, Gourie-Devi M. The syndrome of inappropriate antidiuretic hormone secretion in tuberculous meningitis. J Assoc Phys Ind 1997; 45: 933-5
67. Singh BS, Patwari AK, Deb M. Serum sodium and osmolal changes in tuberculous meningitis. Indian Pediatr 1994; 31: 1345-50
68. Narotam PK, Kemp M, Buck R, et al. Hyponatremic natriuretic syndrome in tuberculous meningitis: the probable role of arterial natriutetic peptide. Neurosurgery 1994; 34: 982-8
69. Adrogue HJ, Madias NE. Hyponatremia. N Engl J Med 2000; 342: 1681-9
70. Gujjar AR. Sodium dysregulation and infections of central nervous system. Ann Ind Acad Neurol 2003; 6: 253-8
71. Harrigan MR. Cerebral salt wasting syndrome. Crit Care Clin 2001; 17: 125-38
72. Celik US, Alabaz D, Yildizdas D, et al. Cerebral salt wasting in tuberculous meningitis: treatment with fludrocortisone. Ann Trop Paediatr 2005; 25: 297-302
73. Ti LK, Kang SC, Cheong KF. Acute hyponatraemia secondary to cerebral salt wasting syndrome in a patient with tuberculous meningitis. Anaesth Intensive Care 1998; 26: 420-3
74. Ravishankar B, Mangala, Prakash GK, et al. Cerebral salt wasting syndrome in a patient with tuberculous meningitis. J Assoc Physicians India 2006; 54: 403-4
75. Huang SM, Chen CC, Chiu PC, et al. Tuberculous meningitis complicated with hydrocephalus and cerebral salt wasting syndrome in a three-year-old boy. Pediatr Infect Dis J 2004; 23: 884-6
76. Das R, Nagaraj R, Murlidharan J, et al. Hyponatraemia and hypovolemic shock with tuberculous meningitis. Indian J Pediatr 2003; 70: 995-7
77. Loo KL, Ramachandran R, Abdullah BJ, et al. Cerebral infarction and cerebral salt wasting syndrome in a patient with tuberculous meningoencephalitis. Southeast Asian J Trop Med Public Health 2003; 34: 636-40
78. Kroll M, Juhler M, Lindholm J. Hyponatremia in acute brain disease. J Intern Med 1992; 232: 291-7
79. Sarkarcan A, Boochini J.Jr. Rhe role of fludrocortisone in a child with cerebral salt wasting. Pediatr Nephrol 1998; 12: 769-71
80. Camous L, Valin N, Zaragoza JLL, et al. Hyponatremic syndrome in a patient with tuberculosis – always the adrenals? Nephrol Dial Transplant 2008; 23: 393-5
81. Satishchandra P, Sinha S. Seizures in HIV-seropositive individuals: NIMHANS experience and review. Epilepsia 2008; 49(Suppl. 6): 33-41
82. Murthy JMK, Jayalaxmi SS, Kanikannan MA. Convulsive status epilepticus: clinical profile in a developing country. Epilepsia 2007; 48: 2217-23
83. Narayanan JT, Murthy JMK. Nonconvulsive status epilepticus in a neurological intensive care unit: profile in a developing country. Epilepsia 2007; 48: 900-6
84. Hesdorffer DC, Benn EKT, Cascino GD, Hauser AW. Is a first acute symptomatic seizure epilepsy? Mortality and risk for recurrent seizure. Epilepsia 2009; 50: 1102-8
85. Narayanan JT, Murthy JMK. New onset Acute Symptomatic seizures in neurological Intensive Care unit. Neurol India 2007; 55: 136-40
86. Patel NC, Landan IR, Levin J, et al. The use of levetiracetam in refractory status epilepticus. Seizure 2006; 15: 137-41
87. Rupprecht S, Franke K, Fitzek S, et al. Levetiracetam as a treatment option in non-convulsive status epilepticus. Epilepsy Res 2007; 73: 238-44
88. Rossetti AO, Bromfield EB. Determinants of success in the use of oral levetiracetam in status epilepticus. Epilepsy Behav 2006; 8: 651-4
89. Murthy JMK. Acute symptomatic seizures: clinical and etiological spectrum in developing countries. In: Murthy JMK, Senanayake N (eds.). Epilepsy in tropics. Georgetown: Landes Bioscience, 2006; pp. 133-43
90. Patsalos PN, Perucca E. Clinically important drug interactions in epilepsy: interactions between antiepileptic drugs and other drugs. Lancet Neruol 2003; 2: 473-81
91. Miller RR, Porter J, Greebblatt DJ. Clinical importance of the interaction of phenytoin and isoniazid: a report from the Boston Collaborative Drug Surveillance Program. Chest 1979; 75: 353-8
92. Mathew NT, Abraham J, Chandy J. Cerebral angiographic features in tuberculous meningitis. Neurology 1970; 20: 1015-23
93. Figaji AA, Sndler SIJ, Feiggen AG, et al. Continuous monitoring and intervention for cerebral ischemia in tuberculous meningitis. Pediatr Crit Care Med 2008; 9: e25-e30
94. Schoeman JF, Elshof JW, Laubscher JA, et al. The effect of adjuvant steroid treatment on serial cerebrospinal fluid changes in tuberculous meningitis. Ann Trop Paediatr 2000; 21: 299-305
95. van der Fkuer M, Hoppenreijs S, van Rensburg AJ, et al. Vascular endothelial growth factor and blood-brain barrier distruption in tuberculous meningitis. Pediatr Infect Dis J 2004; 23: 608-13
96. Gujjar AR, Srikanth SG, Umamaheshwara Rao GS. HHH regime for arteritis secondary to TB meningitis: a prospective randomized study. Neurocrit Care 2009; 10: 313-7
97. Singh D, Kumar S. Ventriculoperitoneal shunt in post tubercular hydrocephalus. Ind Pediatrics 1996; 33: 854-5
98. Mathew JM, Rajshekhar V, Chandy MJ. Shunt surgery for poor grade patients with tuberculous meningitis and hydrocephalus: effect of response to external ventricular drainage and other factors on long-term outcome. Journal of Neurology, Neurosurgery and Psychiatry 1998; 65: 115-8
99. Agrawal D, Gupta A, Mehta VS. Role of shunt surgery in pediatric tubercular meningitis with hydrocephalus. Indian Pediatrics 2005; 42: 245-50
100. Sil K, Chatterjee S. Shunting in tuberculous meningitis: a neurosurgeon’s nightmare. Childs Nerv Syst 2008; 24: 1029-32
101. Schoeman JF, Laubacher JA, Donald PR. Serial lumbar SCF pressure measurements and cranial computed tomographic findings in childhood tuberculous meningitis. Child’s Nerv Syst 2000; 103: 40-3
102. Goiein KJ, Tamir I. Cerebral perfusion pressure in central nervous system infections of infancy and childhood. J Pediatr 1983; 103: 40-3
103. Rebaud P, Berthier JC, Haremann E, et al. Intracranial pressure in childhood central nervous system infections. Intensive Care Med 1988; 14: 522-5
104. Flores-Cordero JM, Amaya-Villar R, Rincón-Ferrari MD, et al. Acute community-acquired bacterial meningitis in adults admitted to the intensive care unit: clinical manifestations, management and prognostic factors. Intensive Care Med 2003; 29: 1868-70
105. Gliemroth J, Bahlmann L, Klaus S, et al. Long-time microdialysis in a patient with meningoencephalitis. Clin Neurol Neurosurg 2002; 105: 27-31
106. Shetty R, Singhi S, Singhi P, et al. Cerebral perusion pressure-targeted approach in children with central nervous system infections and raised intracranial pressure: is it feasible? J Child Neurol 2008; 23: 192-8
107. Lindvall P, Ahlm C, Ericsson M, et al. Reducing intracranial pressure may increase survival among patients with bacterial meningitis. Clin Infect Dis 2004; 38: 384-90
108. Himmelseher S. Hypertonic saline solutions for treatment of intracranial hypertension. Curr Opin Anaesthesiol 2007; 20: 414-26
109. Schoeman J, Donald P, van Zyl L, et al. Tuberculous hydrocephalus: comparison of different treatments with regard to ICP, ventricular size and clinical outcome. Dev Med Child Neurol 1991; 33: 396-405
110. Lamprecht D, Schoeman J, Donald P, et al. Ventriculoperitoneal shunting in childhood tuberculous meningitis. Br J Neurosurg 2001; 15: 119-25
111. Kemaloglu S, Ozkan U, Bukte Y, et al. Timing of shunt surgery in childhood tuberculous meningitis with hydrocephalus. Pediatr Neurosurg 2002; 37: 194-8
112. Girgis Nl, Sultan Y, Farid Z, et al. Tuberculosis meningitis, Abbassia Fever Hospital-Naval Medical Research Unit No. 3-Cairo, Efypt from 1976 to 1996. Am J rop Med Hyg 1998; 58: 26-34
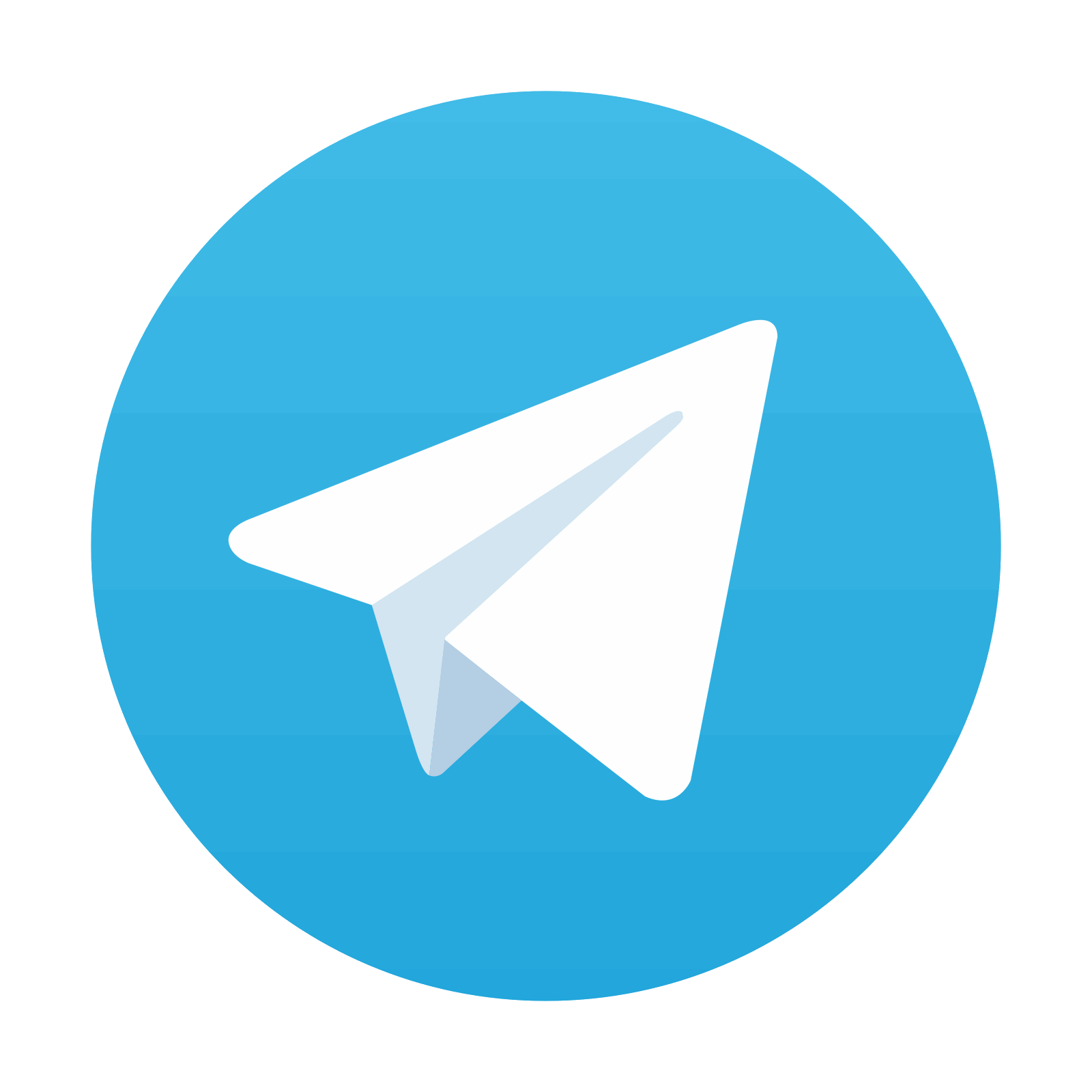
Stay updated, free articles. Join our Telegram channel

Full access? Get Clinical Tree
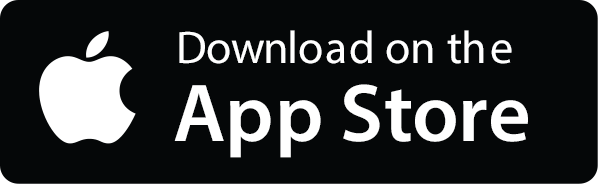
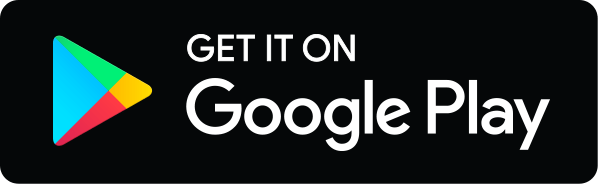