Table 71.2 Efficacy of VNS Therapy in Clinical Studies
aP ≤ 0.05, by Student t-test.
bP < 0.0001, by analysis of variance.
The VNS therapy system is made up of a pulse generator, a bipolar VNS lead, a programming wand with accompanying software for a handheld computer, a tunneling tool, and handheld magnets (Fig. 71.1) (24,25). The generator transmits electrical signals to the vagus nerve through the lead. The software allows placement of the programming wand over the generator for reading and altering stimulation parameters (Fig. 71.2; Table 71.3). Each stimulation period is preceded by 2 seconds of ramp-up time and followed by 2 seconds of ramp-down time.
Figure 71.1. Implantable components of the VNS therapy system.
Figure 71.2. VNS therapy stimulation parameters.
Table 71.3 VNS Therapy Parameters
Values in bold type are the most common settings from the E03 and E05 studies.
VNS, vagus nerve stimulation.
aFrom Refs. A randomized controlled trial of chronic vagus nerve stimulation for treatment of medically intractable seizures: The Vagus Nerve Stimulation Study Group. Neurology. 1995;45:224–230; Vonck K, Thadani V, Gilbert K, et al. Vagus nerve stimulation for refractory epilepsy: a transatlantic experience. J Clin Neurophysiol. 2004;21:283–289; Koo B. EEG changes with vagus nerve stimulation. J Clin Neurophysiol. 2001;18:434–441.
Three models of the VNS therapy generators are currently available: the Pulse Model 102 (single pin) and Pulse Duo Model 102R (dual pin), the Demipulse Model 103 (single pin) and Demipulse Duo Model 104 (dual pin), and the newer AspireHC Model 105 (available in single pin only) (Fig. 71.3). The Demipulse generators, which are smaller and lighter than the Pulse generators, and the AspireHC generators have improved diagnostics and faster communication with the programming system. The Demipulse generators may have shorter battery life at higher duty cycles than the Pulse generators. Currently, two leads are available in the United States: the Perennia Model 303 and PerenniaFLEX Model 304. All current lead models are single pin and come in two sizes: 2.0 or 3.0 mm (inner diameters of the helical coil) to account for various sizes of the vagus nerve. Dual-pin leads are no longer available. Therefore, the dual-pin generators (Model 102R and Model 104) are for replacement procedures only in patients with the previous dual-pin lead models. The Demipulse and AspireHC generators and Perennia model leads are not yet available in all countries (see the Addendum for sources of information on the VNS therapy system).
Figure 71.3. The Pulse Model 102, Demipulse Model 103, and Aspire HC Model 105 VNS therapy generators.
EFFICACY
The two pivotal studies—E03 and E05—were designed to demonstrate that high (therapeutic) and low (nontherapeutic) stimulation of the vagus nerve had different effects on the frequency of partial seizures (26,27). The effects of VNS therapy during the 12-week randomized phases of the studies, which began 2 weeks after implantation, were gauged against 12- to 16-week baseline periods. E03 acute-study patients (27) (N = 114 implanted) had epilepsy for an average of 22 years. Seizure frequency was reduced by at least half in 31% of patients in the high-stimulation group, compared with 14% in the low-stimulation group. No patients became seizure free during the acute phase, but some reported reduced seizure severity and improved postictal recovery periods. Patients in the high-stimulation group either aborted or decreased 59.8% of seizures with the magnet. No factors were identified that predicted response.
The similarly designed E05 study was the largest prospective, controlled trial of a device for epilepsy treatment ever conducted (26). Patients (N = 199) had a median of 0.51 to 0.58 seizures per day during baseline. One patient receiving high stimulation became seizure free, and 23.4% of patients had a 50% or more reduction in seizure frequency after 3 months of treatment. The presence or absence of aura did not predict efficacy. Of the implanted patients, 99% completed the study.
Long-Term Clinical Studies
All patients exiting study E03 were offered indefinite open-label treatment at high (effective) stimulation; 100 (88%) of the 114 patients completed an additional 12 months of VNS therapy at therapeutic stimulation levels (14 patients discontinued because of lack of efficacy but were included in the analysis as intent to treat) (28). A median 20% reduction in seizure frequency occurred in the first 3 months of the extension study and improved over the ensuing months. In two-thirds of patients, a minimum 50% reduction during the initial 3 months continued during months 10 through 12. Results among the 195 patients in the continuing long-term E05 study showed a 50% or more reduction in seizure frequency in 35% of patients and a 75% or more reduction in 20% of patients after an additional 12 months of VNS therapy at therapeutic stimulation levels (29). The median reduction in seizure frequency was 45%, with seizure frequency reductions sustained over time and only mild-to-moderate side effects reported.
Postapproval Long-Term Outcomes
In addition to the clinical trial data, postapproval outcome studies show that VNS therapy is an effective treatment with increasing or sustained response rates over time. Response rates from the literature for studies reporting on at least 100 patients with a minimum of 12 months to more than 5 years of follow-up range from 50% to 64% (30–34). A retrospective review of a prospectively created database of 436 consecutive patients, both adults and children, at a single center showed a significant reduction in mean seizure frequency (mean reduction of 55.8%; P < 0.0001) at a mean follow-up of 4.94 years (34). The overall response rate in this group was 64%. A subset of this group that had at least 10 years of follow-up (n = 65) showed continued improvement over time, with a mean reduction in seizure frequency of 75% at 10 years (last observation carried forward, 76%) (35). At each recorded interval at 6 months and at years 1, 2, 4, 6, 8, and 10, seizure frequency was significantly reduced from baseline (P < 0.001) (35). The overall responder rate at last follow-up was 91%, with 10 patients seizure free for at least 2 years before their last follow-up visit. Another retrospective study with at least 12 months of follow-up (mean of 44 months; range of 12 to 120 months) across multiple centers showed a 51% reduction in mean monthly seizure frequency (32). The overall responder rate was 59%, with an additional 13% of patients having a seizure frequency decrease between 30% and 50%. The seizure-free rate in this study was 9%. A prospective, open evaluation of 64 patients reported results for up to 5 years of follow-up (36). No change in AED dosages occurred during the first 6 months of VNS therapy, which lasted for an average of 20 months. In this population with refractory seizures, 44% experienced a substantial reduction in severity and frequency over a long period. A report on long-term outcomes of 30 patients receiving VNS therapy (37) showed continued improvements over time, with 54% of patients at 1 year and 61% at 2 years exhibiting seizure frequency reductions of 50% or more compared with baseline. In a study of 269 patients on unchanged AEDs over 1 year, seizure frequency rates decreased over time from a median decrease of 45% at 3 months to a median of 58% at 12 months, indicating that response to VNS therapy over the long term is sustained and independent of AED changes (31). Small, prospective studies report similar results as well as additional benefits beyond seizure reduction such as reduced postictal periods and seizure duration (38,39). The mechanisms underlying the gradual improvements in response to VNS therapy seen over time in these long-term studies have yet to be elucidated. However, these findings continue to support the fact that VNS therapy has important long-term efficacy in the refractory epilepsy population.
Pediatric, Elderly, and Special Populations
Studies indicate that response to VNS therapy is independent of age, seizure type, or epilepsy syndrome. In a large consecutive series of 141 children 18 years of age and younger with treatment-resistant epilepsy and at least 1 year of follow-up, seizure frequency significantly improved with VNS therapy (mean reduction 58.9%, P < 0.0001) (40). The mean age at initiation of VNS therapy was 11.1 years (range, 1 to 18); 86 (61%) were under age 12 years when they received VNS therapy. The mean duration of VNS therapy was 5.2 years (range, 25 days to 11.4 years). The overall responder rate for this population was 65%, with 41% experiencing 75% or greater reduction in seizure frequency. Comparisons between those older than 12 years of age and those younger than 12 years of age showed no differences in efficacy or safety between the groups. Additional pediatric studies show similar findings in addition to showed increasing response rates over time similar to those seen in the real-world outcome data for adults with VNS (41–46). A retrospective study of 46 children implanted under the age of 18 (median age of 12.1 years) showed median seizure frequency reductions in the range of 60% over 3 years with VNS therapy, with response rates more favorable among patients <12 years of age (42). Particularly favorable results, including reduced seizure frequency and severity and improved quality of life (QoL), have been reported among patients in open-label studies of Lennox–Gastaut syndrome and other refractory childhood epilepsies, such as hypothalamic hamartomas, epileptic encephalopathies, Rett syndrome, Dravet syndrome, and tuberous sclerosis complex (41,47–66). Verbal performance, alertness, motor and cognitive functions, and general behavior improved, sometimes dramatically (49,51,60,67,68). A retrospective study (68) showed that improved QoL (particularly in the area of alertness) was associated with VNS therapy in patients with autism (N = 59) or Landau–Kleffner syndrome (LKS; N = 6), with more than half of the patients in each group also experiencing a 50% or more reduction in seizure frequency at follow-up (12 months of follow-up for autism and 6 months for LKS patients). Studies have also shown both seizure frequency reductions and improved QoL among both institutionalized and noninstitutionalized patients with mental impairment/developmental delay (69,70). VNS therapy also successfully stopped a case of refractory generalized convulsive status epilepticus in a patient 13 years of age (71). Another report among three children admitted to the intensive care unit (ICU) after developing status epilepticus showed that VNS therapy allowed early cessation of status and discharge from the ICU (61). In a study of VNS therapy among geriatric patients, 50 years of age or more, 21 of 31 patients experienced a 50% or greater decrease in seizure frequency at 1 year, accompanied by significant improvements in QoL from baseline over time (72).
Open studies indicate that VNS is a favorable treatment option for a wide range of patients regardless of age or seizure type (40,73–79). A recent meta-analysis of VNS therapy efficacy showed that children and those patients with generalized epilepsy benefited significantly from VNS therapy (79). A study of stimulation parameters among patients of different ages (80) recommended age-related stimulation adjustments based on age-related changes seen in vagus nerve characteristics. Early studies indicated that children might respond more rapidly than adults, with reductions in the interval between stimulations resulting in improved control (Table 71.3) (51,62,67). Additional pediatric studies reported that higher output currents might be required, particularly when lower pulse durations are used (81–83). Optimal stimulus parameter settings for patients of various ages or with specific seizure types or syndromes, however, have not yet been defined.
MECHANISM OF ACTION
The mechanisms by which VNS reduces seizure activity in humans were not known at the time VNS therapy was approved by the FDA. However, considerable progress in mechanistic VNS research has been made over the last 15 years. Electrical stimulation of the peripheral vagus nerve requires polysynaptic transmission to mediate the antiseizure effect. The anatomical distribution of vagal projections underlies the therapeutic actions of VNS therapy. Vagal visceral afferents have a diffuse CNS projection, with activation of these pathways broadly affecting neuronal excitability (25,84,85). Another review (84) examined the vagus nerve projections and CNS connections, as well as the current animal and human imaging studies, which indicate that VNS exerts both acute and long-term antiepileptic effects.
EXPERIMENTAL STUDIES
The first studies of the anticonvulsant effects of VNS were conducted in 1937 (85). Subsequent experiments in cats showed that vagal stimulation produced EEG desynchronization (86) or synchronization, depending on the parameters used (87,88). Stimulation of the slow-conducting fibers most effectively resulted in EEG desynchronization. Hypersynchronized cortical and thalamocortical neuronal interactions characterize seizures; therefore, it was postulated that desynchronizing these activities would lead to anticonvulsant effects of VNS.
Initial work in cats and recent studies of strychnine-induced seizures in the dog, maximal electroshock and pentylenetetrazol-induced seizures in the rat, and the alumina-gel monkey model (21,86,89–92) showed that cervical vagal stimulation decreased interictal epileptiform discharges (IEDs) and shortened or aborted seizures; the antiepileptic effects outlasted the stimulus (21,89,92,93) and depended on its frequency and cumulative duration (89–91,93). These effects are now known to be mediated by activation of myelinated A and B fibers (94–96). Most central projections of the vagus nerve terminate in the nucleus of the solitary tract, with extensions to brainstem nuclei, thalamus, amygdala, and hypothalamus. Increased release of gamma-aminobutyric acid (GABA) and glycine by brainstem and subcortical nuclei was proposed as the antiepileptic mechanism of VNS therapy (90,91). Brainstem nuclei are known to influence seizure susceptibility (97–101); based on animal studies, the nucleus of the tractus solitarius is likely the key brainstem structure involved in transmitting and modulating VNS antiseizure effects.
Also unknown are the processes that mediate the sustained anticonvulsant effect of VNS therapy, but this effect, which outlasts the stimulation, suggests long-term changes in neural activity. Expression of fos immunoreactivity was induced by VNS in regions of the rat brain important in epileptogenesis (102); fos immunolabeling in the locus caeruleus suggested VNS modulation of norepinephrine release. Increased norepinephrine release by the locus caeruleus is antiepileptogenic. In rats with chronic or acute locus caeruleus lesions, VNS-induced seizure suppression was attenuated, supporting a noradrenergic mechanism (97). This first evidence of a structure mediating the anticonvulsant action of VNS may have pharmacologic implications for clinical practice. Drugs that activate the locus caeruleus or potentiate norepinephrine effects may enhance the efficacy of VNS. Pending the results of further animal testing, it is likely that the antiepileptic action of VNS is mediated through neuronal networks that project from brainstem to forebrain structures. Vagal projections to noradrenergic and serotonergic neuromodulatory systems of the brain may also explain the positive effects of VNS in improving mood disorders.
In summary, animal studies have established three distinct temporal patterns for the anticonvulsant effects of VNS: (i) acute abortive effects, in which an ongoing seizure is attenuated by VNS; (ii) acute prophylactic effects, in which seizure-inducing agents are less effective in provoking seizures when applied at the end of VNS; and (iii) chronic progressive prophylactic effects, in which total seizure counts are reduced more following chronic VNS stimulation. In addition, animal studies have shown that VNS can antagonize the development of epilepsy in the kindling model of epileptogenesis (103). Based on these studies, the mechanism of action of VNS therapy appears to be largely distinct from that of AED therapies (84).
CLINICAL STUDIES
Initial scalp recording performed in a small number of adults did not demonstrate a significant effect of VNS on EEG total power, median frequency, power in any of the conventional frequency bands (104), interictal epileptiform activity, or the waking or sleep background rhythms (22,104–106). At seizure onset, however, VNS has terminated both the clinical and the EEG seizure activity (106). Studies that are more recent have suggested that some patients may have a change in IEDs with VNS. Fifteen adults with refractory partial-onset seizure disorders showed a significant reduction in IEDs during stimulation and the interstimulation period immediately following stimulation, compared with baseline, with the reduction in interical epileptiform discharges greater among patients whose seizures decreased by more than 50% on VNS. Additionally, the patients who had a significant decrease in interical epileptiform discharges experienced the positive effect of magnetic activation, resulting in extra stimulation, abolishing seizures (107). A single adult patient undergoing presurgical evaluation with intrahippocampal depth electrodes showed alteration of interical epileptiform discharges by VNS (increased spikes at 5 Hz, decreased at 30 Hz) (108). Chronic VNS in children was recently reported to reduce IEDs (109). However, this population was quite different from that in the earlier adult series. Included were patients with generalized and partial-onset seizures, greater frequency of interical epileptiform discharges, and younger age. During 12 months of VNS therapy, both generalized and focal spikes were diminished; however, this did not correlate well with seizure reduction. Pattern reversal visual evoked potentials, brainstem auditory evoked potentials, and cognitive (P300) potentials were all unaffected by VNS (110).
Release of anticonvulsant neurotransmitters at the projection sites of vagus nerve afferent fibers was hypothesized as a mechanism of action (110,111). Cerebrospinal fluid samples assayed for amino acid and neurotransmitter metabolites in 16 patients before and after 3 months of VNS therapy showed a treatment-induced increase in GABA (an inhibitory amino acid), a decrease in aspartate (an excitatory amino acid), and an increase in ethanolamine (a membrane lipid precursor) (111).
Positron emission tomography (PET) H215O cerebral blood flow (CBF) imaging identifies the neuroanatomical structures recruited by VNS in humans. A pilot study of three adults showed activation of the right thalamus, right posterotemporal cortex, left putamen, and left inferior cerebellum (112). Localization to the thalamus may explain the therapeutic benefit of VNS and is consistent with the role of that structure as a generator and modulator of cerebral activity. Moreover, anatomic and physiologic evidence from both animal and human data further support the role of the thalamus in epilepsy (113), with stimulation of either the anterior thalamic nucleus or centromedian thalamic nucleus in animals being associated with anticonvulsant effects (114). In a study of high and low stimulation (115), PET demonstrated CBF alterations at sites that receive vagal afferents and projections, including dorsal medulla, right postcentral gyrus, thalamus, cerebellum bilaterally, and limbic structures (bilateral hippocampus and amygdala). The high-stimulation group had more activation and deactivation sites, although the anatomical patterns during VNS were similar in both groups. Finally, acute CBF alterations were correlated with long-term therapeutic response, in an attempt to exclude those regions that show changes in VNS-induced synaptic activity, but may not participate in VNS-related anticonvulsant actions (116). Decreased seizure frequency was associated with increased CBF only in the right and left thalami. Studies of chronic VNS therapy have shown the same anatomical distribution of CBF (112,117). Demonstration of these acute regional alterations does not clarify the mechanism of action of long-term, intermittent VNS, which may involve neurotransmitters or neurochemicals at those sites that outlast the stimulation.
Functional magnetic resonance imaging (fMRI) evaluating the time course of regional CBF alterations during VNS therapy can be performed safely in patients implanted with a vagal nerve stimulator (118). Preliminary fMRI studies have agreed with the PET studies, with the most robust activation observed in the thalami and insular cortices, with some activation also seen in ipsilateral basal ganglia, anterior parietal cortex, and other cortical areas (118,119).
SELECTION OF CANDIDATES
In the United States, VNS therapy is indicated as an adjunctive treatment for adults and adolescents 12 years of age or older with refractory partial-onset seizures (24). In the European Union, VNS therapy is indicated as an adjunctive treatment for patients with partial- or generalized-onset seizures without an age limitation. However, indications for VNS therapy were derived from the clinical trial experience, not from an understanding of its physiologic action. Age, sex, seizure type or syndrome, etiology, frequency of seizures, or IEDs do not predict response to VNS therapy. The type or number of coadministered AEDs also does not predict response (73,120). However, many studies show favorable outcomes in many of these populations, although complete seizure freedom is rarely achieved (79). A recent study also indicates that VNS therapy should be considered in patients with posttraumatic epilepsy, which is often resistant to AED therapy and not often amenable to epilepsy surgery (121).
In October 2013, the American Academy of Neurology updated the evidence-based VNS therapy guidelines for the treatment of epilepsy (122). The updated guidelines provide an update on data regarding the efficacy and safety of VNS therapy for epilepsy since publication of the original guidelines in 1999. Previous guidelines did not provide guidance on many of the epilepsy patient populations in which VNS therapy is actively being used. The literature review found 1274 manuscripts for VNS therapy since the last review. Of these, 216 articles were reviewed to answer 8 clinical questions, summarized in Table 71.4. The clinical evidence supports the use of VNS therapy across a range of refractory epilepsy populations, and the impact of the reviewed studies (with specific focus on the intensity and magnitude of the responses) continues to be quite supportive.
Table 71.4 Summary of AAN Updated Evidence-Based Guidelines for VNS Therapy
aRapid cycling is shorter On times and Off times, typically 7 s On and 30 s Off compared with standard settings, typically 30 s On and 5 min Off.
C, based on data from class III studies; U, unproven, data inadequate or conflicting.
Although optimal use parameters continue to be defined, candidates should meet the following criteria: (i) medically refractory seizures, (ii) adequate trials of at least two AEDs, (iii) exclusion of nonepileptic events, and (iv) ineligibility for epilepsy surgery (Fig. 71.4). Focal resective surgery (temporal lobectomy or lesional neocortical epilepsy) is preferred in appropriate patients because of its superior seizure-freedom rate (123–125). Recent open studies suggest that VNS therapy may be used among patients considered for corpus callosotomy, producing lower rates of morbidity (116–129), and among those who have previously undergone epilepsy surgery (41,130–132). A study of 376 patients (110 who had at least 1 failed craniotomy before VNS and 266 with no history of intracranial epilepsy surgery [IES] before VNS) showed no significant difference in the mean percentage seizure reduction between patients with and without a history of IES (59% vs. 57%; P = 0.42) (132). Earlier use (within 2 years of seizure onset or after failure of two or three AEDs) of VNS therapy may also produce a higher response rate and reduce the negative side effects associated with long-term epilepsy and AED therapy, which hinder development (30,40,133,134). Patients with a history of nonadherence to their AED regimens, particularly those on polypharmacy, may also be good candidates for VNS therapy because of the assured compliance and lack of further drug–drug interactions with VNS therapy (135,136).
Figure 71.4. Treatment selection flow chart. AED, antiepileptic drug; VNS, vagus nerve stimulation therapy.
Use of VNS therapy is contraindicated in patients with prior bilateral or left cervical vagotomy, and safety and efficacy have not been established for stimulation of the right vagus nerve. Patients with existing pulmonary or cardiac disease should be evaluated carefully before implantation; chronic obstructive pulmonary disease may increase the risk for dyspnea. Patients with cardiac conduction disorders were not studied in the controlled trials. A cardiologist’s evaluation should precede implantation, with postprocedural Holter monitoring performed if clinically indicated. Patients with a history of obstructive sleep apnea should be treated with care, as an increase in apneic events during stimulation is possible (137,138). Lowering stimulation frequency (i.e., pulse width and signal frequency to 250 μs and 20 Hz, respectively) may prevent exacerbation of this condition (137). However, most studies showing a decrease in airflow during sleep with VNS therapy reported this condition to be clinically insignificant (138). Moreover, beneficial effects on sleep and increases in slow-wave sleep also have been reported with VNS therapy, which may play a role in the antiepileptic mechanisms of VNS (139,140).
INITIATION AND MAINTENANCE
Hospitalization for implantation of the device is preceded by evaluations by a neurologist and by a surgeon with experience in the carotid sheath. With the patient typically under general anesthesia (although local or regional anesthesia has been used successfully as well) (141), the lead wires are placed on the left cervical vagus nerve and the generator is placed in a subcutaneous pocket in the left upper chest (142,143). Intraoperative electrical impedance testing ensures integrity of the system. Rare cases of bradycardia, asystole, or both mandate initial lead testing in the operating room (24,144,145); the anesthesiologist should be notified immediately before this test. Stimulation following intraoperative bradycardia has been shown to be safe, with no change in cardiac rhythm upon initiation of postoperative VNS, which was started under ECG monitoring (146). Reimplantation of a second VNS therapy generator upon battery depletion in two patients also showed no occurrence of bradycardia (146). Correct placement of the lead electrodes around the vagus nerve is critical. If there is concern about the lead placement, two methods have been utilized to help confirm correct placement of the electrodes intraoperatively (147), depending on the type of anesthesia used for the procedure. For patients receiving general anesthesia, the larynx and vocal cords can be monitored by fiberoptic endoscopy for contraction of the left lateral larynx wall and vocal cord tightening. For patients being implanted under local and regional anesthesia, stimulation intensities can be increased until a voice alteration is noticed. The procedure neither is harmful to the patient nor greatly extends the length of the surgery.
Prophylactic antibiotics may be administered both in the operating room and postoperatively. The patient can be discharged after the procedure, which usually lasts for <1 hour, or can be observed overnight. Discharge education should include care of the incisions and use of the magnet. In clinical studies, the generator’s output current was kept at 0 mA for the first 2 weeks; however, programmed stimulation is now being initiated at 0.25 mA in many operating rooms (41). Dosages of AEDs are generally kept stable for the first 3 to 6 months of stimulation unless an early response is noted.
A few weeks after implantation, the patient is examined to confirm wound healing and proper generator operation either to begin or to continue programming. Output current is increased in 0.25 mA increments as long as stimulation is comfortable (Table 71.1). The subsequent stimulation schedule is determined by patient response. Standard parameter settings range from 20 to 30 Hz at a pulse width of 250 to 500 μs for 30 seconds “on” time and 5 minutes “off” time (83). For an adult, to generate an action potential in the nerve (a therapeutic effect), research shows that optimal output current settings may range from 0.75 to 1.75 mA (148). At each visit, the generator and the battery are assessed for end of service; the battery’s life expectancy of 7 to 10 years depends on the programmed stimulation parameters. If VNS therapy is to be continued, the generator can be replaced at the appropriate time in <20 minutes.
VNS may be continued indefinitely and without damage to the vagus nerve as long as the stimulation is <50 Hz and the on time remains less than the off time (24,149,150). Two safety features that protect patients from continuous stimulation or uncomfortable side effects are the magnet and the watchdog timer. The magnet can act as an “off” switch when held or taped over the generator. The watchdog timer is an internal monitor that limits the number of pulses to be delivered without an “off” time to prevent excess stimulation.
COMPLICATIONS AND ADVERSE EFFECTS
Surgical complications and difficulties are rare. Fracture of the electrode, related to fatigue at the junction between contact and the lead wire, was a common problem with early devices (23,151–154). Substitution of a quadrifilar wire and, later, a trifilar lead body coil improved electrode tolerance that had been compromised by repetitive neck motion. The two newest lead models (Perennia Model 303 and PerenniaFLEX Model 304) are designed to be even more resistant to fractures than previous models. The PerenniaFLEX is similar in design to the Model 302 but has a lead body designed with three high fatigue silicone tubes; the bifurcation is still caudal to the anchor tether but designed with a smoother transition to facilitate strain relief bend. The Perennia is constructed with a trifilar lead body coil and a continuous bilumen lead body silicone tube with the bifurcation cephalad to the anchor tether; this design makes the handling characteristics of the Perennia lead feel stiffer during the implantation procedure compared with the Model 302 and 304 leads. The Perennia model leads are approved by the FDA but are not currently available in all countries.
Incisional infections are unusual and generally respond to antibiotic therapy. Fluid accumulation at the generator site with or without infection occurs in 1% to 2% of implantations and resolves with aspiration and antibiotics; the rare cases of refractory infection require removal of the generator. However, one case of deep wound infection associated with implantation of the generator was reported to be managed successfully with open wound treatment without removal of the device, an alternative option if removal of the device appears hazardous (155). Unilateral vocal cord paralysis, which accompanies approximately 1% of implants, may be caused by excess manipulation of the vagus nerve, and subsequent damage to the vagal artery and its reinforcing arterioles (156); in most cases, it remits completely over several weeks.
Common side effects, which occur primarily when the stimulator is actually delivering a pulse (Table 71.5), are dose dependent and usually mild or absent when VNS parameters are appropriately programmed (26,27,157); many patients become accustomed to them with time. Most patients experience hoarseness or a change in vocal quality and tingling over the left cervical region on delivery of the electrical pulse. Subjective dyspnea or a sensation of muscle tightening in the neck may occur, without changes on pulmonary function testing (26). Cough or throat pain during stimulus delivery sometimes necessitates a reduction in current or pulse width (158).
Table 71.5 Adverse Events with Vagus Nerve Stimulationa
aNumber of patients reporting the adverse event at least once in the E03 and E05 randomized studies.
Despite the widespread visceral efferent projections of the vagus nerve, systemic effects are rare. Pulmonary function does not change significantly in patients without concomitant lung disease (26,159) but may deteriorate in the face of intense stimulation and obstructive lung disease (159). Inhalation of ipratropium bromide or lowering of the stimulus frequency or current is recommended. No substantial effects on cardiac function were reported during clinical studies (24,26,27,157,160). An analysis of total mortality and sudden death in epileptic patients (to August 1996) revealed the expected rate in individuals with severe, intractable epilepsy (161,162). The clinical studies demonstrated no clinically relevant effects on the gastrointestinal system, serum chemistries, AED concentrations, vital signs, or weight.
Rare reported side effects associated with VNS therapy include diarrhea (163), sternocleidomastoid muscle spasm (164), phrenic nerve stimulation (165), tonsillar pain (166), emergent psychiatric disorders (167,168), and prominent drooling and vomiting (169). Of seven patients treated with VNS therapy who developed a major psychiatric disorder (167), all had a history of a dysphoric disorder and most had daily seizures before treatment with VNS. The severe dysphoric or psychotic conditions emerged once seizure frequency was reduced by 75% or more but remitted or improved satisfactorily with psychotropic medication, with two patients also requiring a decrease or interruption of VNS therapy. Children with a history of dysphagia may experience swallowing difficulties during VNS therapy (169–171); adjusting the device settings or using a magnet to turn off the stimulator during mealtime may help. The majority of side effects, including many of the rare incidents reported, are amenable to stimulus modifications, which could include changes in output current and/or pulse width.
ADVANTAGES AND DISADVANTAGES
Many patients maintained on VNS therapy can decrease their total AED burden, which typically results in a more alert patient who, while still receiving polytherapy, is without the cognitive or systemic side effects typically associated with multiple therapies. Therefore, use of AED monotherapy with VNS therapy may produce a better risk to benefit ratio than treatment with two AEDs. Even when AEDs cannot be substantially decreased or withdrawn, however, VNS therapy may allow amelioration of seizures with no risk of toxic organ reactions, drug interactions or failures, allergies, rashes, and other systemic adverse effects or cognitive side effects (172,173); in some patients, memory, alertness, mood, and communication have been shown to improve (105,174–178). Improvements in QoL independent of treatment effect on seizure frequency, as well as increased daytime vigilance, have also been reported (179–181). In addition, because the beneficial results are maintained without active patient participation, VNS therapy may be an ideal treatment for the partially compliant (135,136). Teratogenesis is not expected with VNS therapy. Although no controlled studies of VNS therapy in pregnancy have been conducted, an animal study showed no harm to fertility or to the fetus (182). Cases also have been reported in the literature of patients who became pregnant while on VNS therapy and gave birth to healthy babies (36,183). Finally, VNS therapy can both prevent and abort seizures. The ability to trigger the device externally (with the magnet) and to interrupt the seizure or improve the postictal phase empowers the patient and provides a sense of control over epilepsy.
On the other hand, VNS is an empiric therapy, with no way to predict response except by trial. The initial cost (often between $15,000 and $25,000) can be prohibitive without coverage by a third-party payer. Over the life of the system, however, this cost approximates that of many of the new AEDs (184). Moreover, although weeks to months may elapse before seizure frequency decreases, cost-effectiveness studies indicate that VNS therapy provides a substantial cost-savings benefit to hospitals over the long-term course of treatment (185,186). These cost benefits are sustained over time and are sufficient to cover or exceed the cost of the device. Further savings can be seen in significant reductions in health care utilization and time spent on epilepsy-related matters with VNS therapy over time. One study looked at health care utilization of 138 patients with refractory epilepsy comparing 1 year of baseline data followed by 4 years of quarterly follow-up data with VNS therapy and showed significant reductions in the numbers of emergency department visits (99% decrease), hospitalizations (70% decrease), and hospital lengths of stay (67% decrease) beginning with the first quarter after VNS implantation and therapy (P < 0.05 for all postimplantation quarters) (187). A 91% decrease was also seen in outpatient visits post-VNS therapy, and significant decreases were seen for average number of days on which patients could not work because of health-related concerns (P = 0.002) and average time spent caring for health problems (P < 0.001), which further reflect positive changes in the QoL of both patients and their caregivers as a result of VNS therapy in addition to health care utilization savings. In a US study evaluating the long-term medical and economic benefits of VNS therapy using Medicaid data from 5 states (n = 1655), VNS therapy was associated with lower average health care costs and epilepsy-related clinical events (135
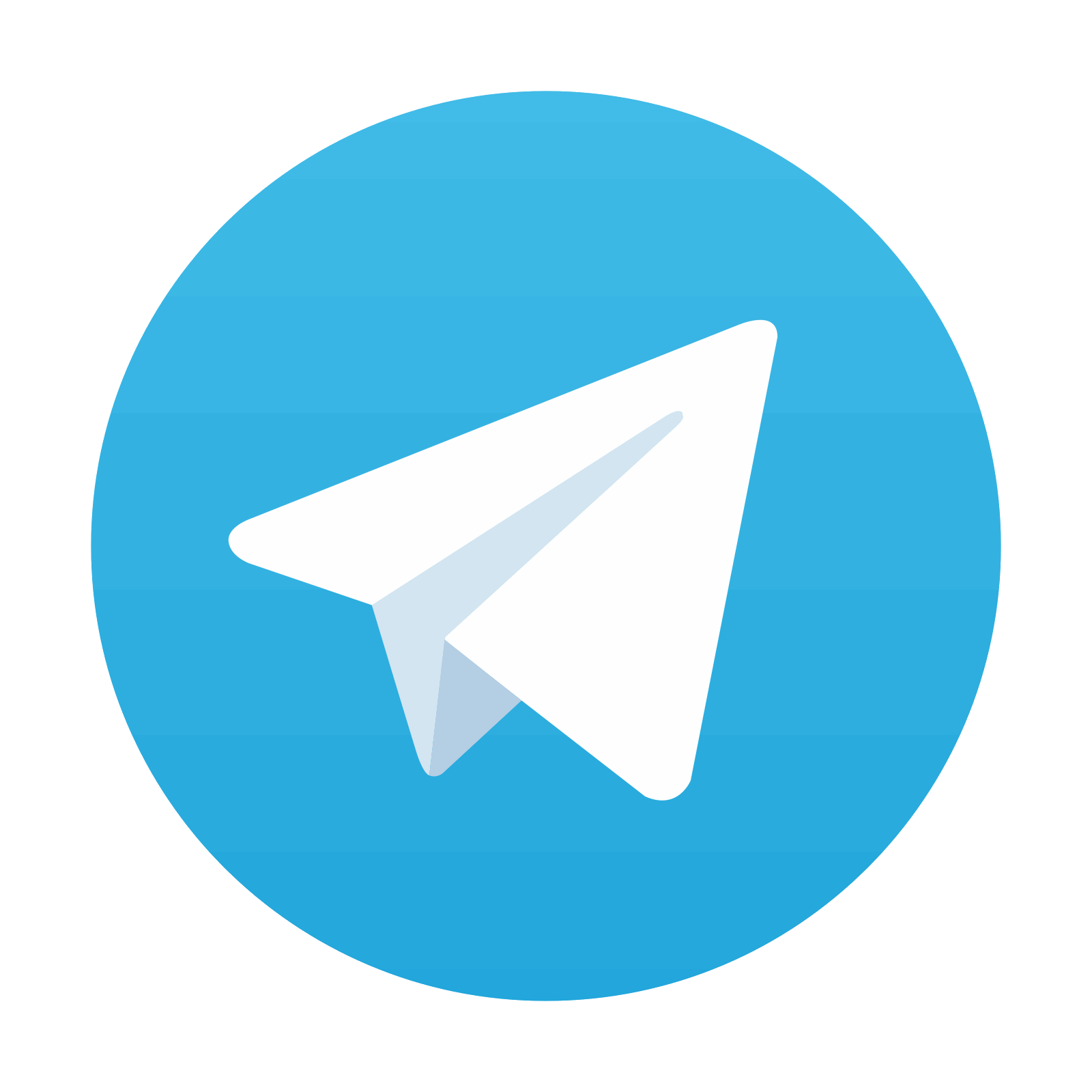
Stay updated, free articles. Join our Telegram channel

Full access? Get Clinical Tree
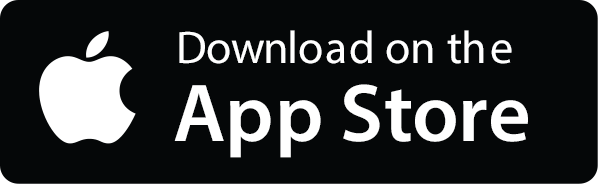
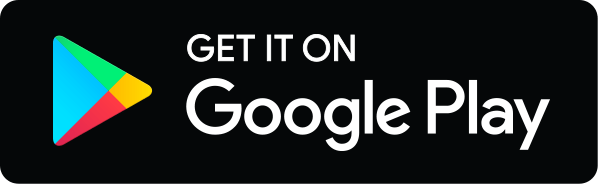