The purpose of vestibular laboratory testing is to assess the peripheral and central vestibular system in patients with suspected labyrinthine dysfunction. Such information can be used to help establish a diagnosis and develop a treatment plan. Vestibular laboratory testing sometimes provides lateralizing information (i.e., information regarding whether the lesion is on the left or the right). It also can help to assess the status of central nervous system (CNS) compensation for a unilateral peripheral vestibular loss.
The majority of the involved laboratory techniques focus on the assessment of the horizontal vestibulo-ocular reflex (VOR). Newer technologies such as vestibular evoked myogenic potentials, galvanic vestibular stimulation, and off-axis rotation (unilateral centrifugation) may allow a more thorough assessment of the vestibular system. This chapter focuses on how and why the VOR is assessed in the vestibular laboratory. Additionally, computerized dynamic posturography and newer technologies are discussed that assess other aspects of vestibular function. The clinical application of vestibular laboratory testing is also considered in selected disorders. Figure 32-1 provides an overview of those portions of the vestibular labyrinth that are assessed by the various tests discussed in this chapter.

Indications for vestibular testing
Table 32-1 lists the uses of vestibular laboratory testing. A specialist with a high level of suspicion that a vestibular disorder is present typically requests evaluation in the laboratory. Such testing can aid in determining whether a vestibular system abnormality is present, whether the problem can be localized to the central or peripheral vestibular system, whether the problem can be lateralized to the left or right side, and whether a peripheral abnormality can be localized to the semicircular canals or otolith organs. Vestibular testing provides quantitative information useful for documenting the presence of an abnormality only suspected by bedside evaluation. Moreover, vestibular laboratory testing can provide information that may be useful in deciding about treatment with balance rehabilitation. Occasionally, by repeating some of the tests, vestibular laboratory testing is helpful in the long-term management of patients.
|
Techniques for recording eye movements
Electro-Oculography
Electro-oculography (EOG), also called electronystagmography (ENG), is based on the corneo-retinal dipole potential of the eyeball ( Fig. 32-2 ). This potential is thought to result from the metabolic activity of the retina. When the eye rotates in the orbit, the dipole also rotates. Silver–silver chloride electrodes placed near the orbit can be used to record this dipole and, thus, eye position. The combined movement of both eyes can be recorded with one pair of electrodes placed bitemporally. The movement of each eye can be recorded separately ( Fig. 32-3 ) by placing electrodes to the left and right of each eye. A reference electrode typically is placed on the forehead. The electrodes should not be placed too close to the lateral canthi because this sometimes causes frequent blinking and thereby increases artifacts.


Before the application of the electrodes, the skin is cleansed and prepared thoroughly so as to reduce its impedance to less than 25k ohms. Electrode impedance should not vary from one electrode to another by more than 10k ohms. The electrical signal from the electrodes is amplified using direct-current (DC) rather than alternating-current (AC) coupling because this type of recording provides a more reliable measurement of eye position, especially during visual fixation. For vertical eye-movement recording, electrodes are placed above and below one eye. The signal from vertically placed electrodes is useful, but eyelid movement, muscular activity near the eyebrow, and eye blinks cause artifacts that interfere with the reliability of the recording. Frequent eye blinks can also interfere with horizontal recordings and render the horizontal EOG recording uninterpretable ( Fig. 32-4 ).

The corneo-retinal dipole potential fluctuates during laboratory assessment, especially with changes in ambient luminance ( Fig. 32-5 ). Thus, before the test begins, the patient is kept in a semidarkened room for at least 5 minutes so that the eyes can adapt to the near-darkness. Even with this precaution, the variability in the corneo-retinal dipole potential makes it imperative that calibrations of the EOG signal are performed repeatedly throughout the assessment. Calibration of the EOG signal is performed by asking the patient to look at visual targets placed at 10 degrees to the left and right of center. Most ENG systems use computer-based analysis techniques. Laboratories should adhere to a standardized method of stimulus presentation (e.g., as proposed in the American National Standards Institute [ANSI] protocols). Additionally, the interpretation of eye-movement data should never rely solely on a computer output; rather, an experienced individual should inspect the eye-movement recordings independently.

Video-Oculography
Video-oculography (VOG) is replacing EOG as the eye-movement recording system of choice. The main component of a VOG system, also sometimes called video-ENG or VNG, is a small video camera that sends images to a personal computer for image processing. A representative VOG system is illustrated in Figure 32-6 . Two-dimensional VOG systems extract horizontal and vertical eye movements from the video images using the pupil; three-dimensional VOG systems additionally extract torsional eye position using iris landmarks. Most VOG systems use infrared light and thus can function in complete darkness. VOG systems may be monocular or binocular. Most commercial VOG systems have a standard frame rate of 50 or 60 Hz, which may limit the accuracy of VOG for some ocular motor testing (e.g., assessment of saccades). Some manufacturers offer a higher frame-rate system, but these are considerably more expensive. Two-dimensional VOG, because of its reliable vertical as well as horizontal eye-movement detection, has an advantage over conventional EOG. With VOG, it is essential that the goggles are attached firmly to the patient’s head because any relative movement of the goggles with respect to the head will result in an artifactual change in eye position during the recording. Table 32-2 compares the properties of EOG (or ENG), two-dimensional VOG, and three-dimensional VOG systems.


Characteristics | Electronystagmography | Two-Dimensional Video-Oculography | Three-Dimensional Video-Oculography |
---|---|---|---|
Recording device | Paste-on electrodes (AgCl–Ag) | Video camera | Video camera |
Principle | Corneo-retinal dipole potential | Image processing | Image processing |
Contrast enhancement of pupil | Pupil and iris structure analysis | Cross-correlation or neural network | |
Horizontal eye movement | Reliable | Very reliable | Very reliable |
Vertical eye movement | Unreliable | Very reliable | Very reliable |
Torsional eye movement | Not possible | Not possible | Reliable |
Approximate accuracy | 1–2 degrees | 1–2 degrees | 1 degree |
Head movement resistance | High | If properly secured | If properly secured |
Sampling rate | > 150 Hz | 25–60 Hz | 25–60 Hz |
Iris structure dependence | None | No | Yes |
Amplifier drift | Yes | No | No |
Calibration | Repeatedly | Once | Once |
Sensitive to | |||
Blink artifacts | Yes | Yes | Yes |
Changes in room lighting | Yes | No | No |
Myogenic activity | Yes | No | No |
Vestibular stimuli possible | Yes | Yes | Yes |
Functions in total darkness | Yes | Yes | Yes |
Patient tolerance | About 1 hour | About ½ hour | About ½ hour |
Scleral Search Coil
The scleral search coil method is based on the recording of small electric currents induced by a magnetic field in a coil of very narrow-gauge wire embedded in a pliable donut-shaped plastic ring that is placed on the eye ( Fig. 32-7 ). The scleral search coil method has emerged as the “gold standard” for the accurate recording of eye movements; however, it is an invasive technique that may cause discomfort for the patient. Also, recording time is limited to approximately 30 minutes. The scleral search coil technique generally is confined to research purposes.

Choice of Eye-Movement Recording Technique
EOG has, for many decades, been the standard eye-movement measurement technique. It is a safe, convenient, and efficient means of recording eye position in persons of all ages. The primary limitation of EOG is its inability to provide consistently reliable vertical eye-movement recording. Also, eye blinks produce problematic artifacts, and fluctuations in the corneo-retinal dipole potential can reduce the accuracy of the technique. VOG will replace EOG eventually as the method of choice for recording eye movements during vestibular laboratory testing. It has the advantage of allowing both horizontal and vertical eye-movement recording. As with EOG, eye blink artifacts can be problematic during VOG recordings. Also, relative movement between the VOG goggles and head can produce artifacts.
Definition of Nystagmus
By convention, the eye position that is displayed on a computer screen or chart recorder represents rightward eye movement by an upward deflection and leftward eye movement by a downward deflection. Torsional eye movements should be described from the patient’s viewpoint (i.e., movement of the upper pole of the eye toward the right ear should be displayed as an upward deflection). A slow eye movement followed by a fast movement in the opposite direction is called jerk nystagmus; by convention, this is named after its fast component. Nystagmus is analyzed by computing the slow component velocity (SCV) because the slow movement reflects that portion of the nystagmus generated by the VOR, whereas the fast component is merely a saccadic reset movement ( Fig. 32-8 ).

Vestibular laboratory testing
Reducing Visual Fixation
When testing requires the absence of visual input, two different methods can be used: (1) eyes opened in darkness or (2) eyes closed in a semi-darkened room. The former method is recommended by ANSI because it produces more reliable and reproducible results. Also, closing the eye induces the Bell phenomenon, which may interfere with nystagmus production. Testing with eyes opened in darkness may lead to excessive eye blinking; this sometimes produces artifacts that should not be confused with eye movement (see Fig. 32-4 ). Regardless of the mechanism used to remove visual input, patients should be kept alert because drowsiness reduces the consistency of the VOR. Alerting is often accomplished by asking patients to perform simple mental tasks.
Ocular Motor Screening Battery
The purpose of the ocular motor screening battery is to uncover eye-movement abnormalities that may interfere with the interpretation of the positional, caloric, or rotational tests. Furthermore, the ocular motor screening battery can provide some information concerning CNS abnormalities and, in particular, can provide information regarding CNS function that relates to balance. The ocular motor screening battery consists of several tests, including an assessment of spontaneous nystagmus, gaze-evoked nystagmus, saccadic eye movements, pursuit eye movements, and optokinetic nystagmus.
The term spontaneous nystagmus sometimes denotes a nystagmus that occurs during visual fixation; at other times it denotes nystagmus that occurs only with loss of visual fixation. The term spontaneous vestibular nystagmus should be used to describe nystagmus that is of significantly greater magnitude when visual fixation is lost ( Fig. 32-9 ) and is thus likely to be of vestibular origin. Spontaneous nystagmus should be used for nystagmus seen with visual fixation. Thus, the search for spontaneous vestibular nystagmus should be performed with the patient in darkness. To maintain alertness, the patient should be asked to perform simple mental tasks such as counting backward by threes. To confirm the presence and direction of a spontaneous nystagmus, the patient is asked to look left and right while in darkness. The nystagmus should change its magnitude according to Alexander’s law (i.e., it should increase when the patient looks in the direction of the fast component). Normal individuals may have spontaneous nystagmus, but the SCV of this nystagmus should not exceed about 4 deg/sec. Also, a clinically significant spontaneous nystagmus should be observed consistently during testing; a few isolated beats of nystagmus should not be considered abnormal. Spontaneous vestibular nystagmus is typically horizontal-torsional. As a result, both EOG and two-dimensional VOG will record only the horizontal component of this nystagmus. Spontaneous nystagmus may be either pendular (i.e., having no clearly defined fast and slow components) or jerk (i.e., with clearly defined fast and slow components). Pendular nystagmus may be congenital or acquired. Spontaneous jerk nystagmus most commonly occurs as the result of an acute vestibular lesion. In this case, the spontaneous vestibular nystagmus usually beats away from the impaired labyrinth. For example, an acute right-sided peripheral vestibular lesion usually will be associated with a left-beating spontaneous vestibular nystagmus. Vertical nystagmus seen during ocular motor screening suggests a central abnormality. Different facilities have different criteria to define the limits of normality during ocular motor screening, as is discussed later.

To search for gaze-evoked nystagmus, the patient should be asked to look at visual targets directly in front of him or her and at 30 degrees left and right and 15 degrees up and down. The most common type of gaze-evoked nystagmus is bidirectional, as illustrated in Figure 32-10 . Gaze-evoked nystagmus is considered a result of an abnormal “ocular motor integrator.” Gaze-evoked nystagmus typically is seen with both leftward and rightward gaze and may be seen with upgaze as well. By definition, the fast component of gaze-evoked nystagmus is always in the direction of gaze. Because of Alexander’s law, a spontaneous vestibular nystagmus may be evident only with gaze deviation. For example, a patient with a right-sided peripheral vestibular lesion may demonstrate a left-beating nystagmus only with gaze to the left.

Saccadic eye movements (i.e., rapid eye movements) are tested by asking the patient to look at small visual targets that move abruptly to the left or right at random times, in random directions, and for random distances. Saccadic eye movement typically is recorded monocularly from each eye to uncover abnormalities in voluntary gaze, limitations of gaze, and dysconjugacies. Eye position is used to evaluate the latency, accuracy, and velocity of saccades. Normal values for peak velocity and latency of saccades differ in different laboratories ( Table 32-3 ), as is discussed later. The presence of abnormal saccadic eye movements usually suggests a CNS abnormality, assuming that vision is normal.
Test Items | Normative Limits * | Reference No. |
---|---|---|
Spontaneous nystagmus is abnormal if slow component velocity is | > 4 deg/sec | , |
> 5 deg/sec | , | |
> 6 deg/sec | , | |
> 7 deg/sec | , , | |
> 6 beats/10 sec | ||
Ocular motor testing | ||
Saccades Lower limit of peak velocity for 20-deg saccade | ||
210 deg/sec | , | |
252 deg/sec | ||
283 deg/sec | ||
Latency for 20-deg saccade | 104–365 msec | |
128–255 msec | ||
Smooth pursuit gain at 0.3 Hz | > 0.80 | , , |
Optokinetic nystagmus asymmetry | < 13 % | |
< 16 % | ||
Positional testing | < 6 deg/sec | |
Caloric testing | ||
Reduced vestibular response | > 25 % | |
> 20 % | ||
> 19 % | ||
> 15 % | ||
> 22 % | , | |
> 20 % | ||
> 20 % | ||
> 25 % | ||
General reduced vestibular response limit | > 22 % | |
Directional preponderance | > 23 % | |
> 23 % | ||
> 16 % | ||
> 18 % | ||
> 28 % | , | |
> 26 % | ||
> 27 % | ||
> 32 % | ||
General directional preponderance limit | > 26 % | Meta-analysis |
Rotational chair test | ||
Sinusoidal testing | ||
Gain | ||
0.05 Hz, 60 deg/sec | 0.20–0.80 | |
0.05 Hz, 60 deg/sec | 0.13–0.77 | |
0.05 Hz, 50 deg/sec | 0.24–0.85 | |
0.05 Hz, 60 deg/sec | 0.38–0.98 | |
Phase | ||
0.05 Hz, 60 deg/sec | 6–14 deg | |
0.05 Hz, 60 deg/sec | 2–20 deg | |
0.05 Hz, 50 deg/sec | −1–18 deg | |
0.05 Hz, 50 deg/sec | −1.9–24 deg | |
0.05 Hz, 60 deg/sec | 0.8–20.2 | |
Directional preponderance | ||
0.05 Hz, 60 deg/sec | > 15 % | |
0.05 Hz, 50 deg/sec | > 24 % | |
Velocity step testing at 90 deg/sec | ||
Gain | 0.33–0.72 | |
Time constant | 11–26 sec | |
Directional preponderance | > 22 % | |
Velocity step testing at 100 deg/sec | ||
Gain | 0.27–0.99 | |
Time constant | 5–19.4 sec | |
Cervical vestibular evoked myogenic potentials | ||
Interaural ratio | < 36% | |
< 32% | ||
< 35% | ||
< 52% | ||
Threshold | 69–92 dB nHL | |
68–108 dB nHL | ||
65–89 dB nHL | ||
58–93 dB nHL | ||
Latency | ||
p13 | < 18.4 msec | |
< 15.4 msec | ||
< 17.8 msec | ||
< 17.9 msec | ||
< 18.2 msec | ||
n23 | < 28.1 msec | |
< 23.1 msec | ||
< 26.8 msec | ||
< 28.1 msec | ||
< 25.4 msec |
To assess smooth pursuit, the patient should be asked to follow a visual target moving slowly horizontally. The total excursion of the visual target should be restricted to 30 degrees. Figure 32-11 illustrates asymmetric smooth pursuit while a patient follows a target moving horizontally in a sinusoidal pattern. This type of abnormality suggests a CNS problem. Table 32-3 provides normal values for smooth pursuit gain.

Optokinetic nystagmus is elicited by asking the patient to look at a large visual moving stimulus such as full-field vertical stripes. When the stripes are moved at a constant velocity, the patient should experience circular vection (i.e., an illusory sense of motion of self rather than of the surrounding world). Both clockwise and counterclockwise optokinetic stimuli should be presented. Eye position during optokinetic nystagmus is analyzed by computing the magnitude of the SCV and relating this magnitude to the stimulus velocity. The amount of left–right asymmetry of optokinetic nystagmus should also be computed. Optokinetic nystagmus provides information that is largely redundant because of that provided by ocular pursuit testing. Table 32-3 provides normative data for optokinetic nystagmus.
Positional and Positioning Testing
Positional testing is performed by placing the patient in one of the head lateral positions including the supine, supine-head right, supine-head left, right lateral, and left lateral positions ( Fig. 32-12 ). The nystagmus elicited during positional testing may be direction-fixed or direction-changing. If direction-changing, the nystagmus may be either ageotropic (i.e., beating away from the earth) or geotropic (i.e., beating toward the earth). An example of positional nystagmus is shown in Figure 32-12 . Persistent positional nystagmus is considered a nonspecific, nonlocalizing abnormality that can be caused by a central or peripheral vestibular abnormality. However, persistent ageotropic horizontal positional nystagmus, especially one of high magnitude, may suggest horizontal semicircular canal cupulolithiasis. Paroxysmal rather than persistent geotropic positional nystagmus seen in the ear-down positions (i.e., paroxysmal left-beating in the head left and left lateral positions and paroxysmal right-beating in the head right and right lateral positions) suggests horizontal semicircular canal canalithiasis.


Positioning nystagmus refers to paroxysmal nystagmus that occurs after the patient is moved rapidly into an ear-down position using the Dix–Hallpike maneuver, which is illustrated in Figure 32-13 . The most common form of positioning nystagmus is that associated with benign paroxysmal positional vertigo (BPPV) as a result of movement of free-floating debris in the posterior semicircular canal. The nystagmus is predominantly torsional with a small upbeating component. Thus, only with three-dimensional VOG is this type of nystagmus recorded adequately; when using EOG or two-dimensional VOG, reliable information is not obtained.

Caloric Testing
Despite its numerous disadvantages, the caloric test remains the most established test that can provide lateralizing information about a peripheral vestibular lesion. In particular, caloric testing has low sensitivity and specificity, and assesses only the function of the horizontal semicircular canal. The patient is placed supine with the head tilted up by 30 degrees. In this way, the horizontal semicircular canal is oriented in a vertical plane. After careful inspection of the external auditory canals to exclude a perforated tympanic membrane or other adverse conditions (e.g., impacted cerumen), the external auditory canal is warmed or cooled with water or air sequentially for 30 to 40 seconds. A thermal gradient across the horizontal semicircular canal thus develops and produces a convection current within the endolymph ( Fig. 32-14 ). The warm stimulus induces utriculopetal endolymph flow, which corresponds to an excitation of the horizontal semicircular canal and results in a nystagmus that beats toward the stimulated ear. The cool stimulus induces utriclofugal endolymph flow, which corresponds to an inhibition of the horizontal semicircular canal and results in nystagmus that beats away from the stimulated ear. Although thermal convection accounts for the majority of the caloric response, direct thermal stimulation of the vestibular labyrinth and neural elements appears to account for some of the response. During caloric testing, patients may feel vertiginous. It is very important that patients be informed about this physiologically normal response in an effort to minimize anxiety.

The order in which the four caloric irrigations are performed is not standardized, but the Dutch Vestibular Society suggests that they be delivered in the following order: warm right, warm left, cold right, and finally cold left. The patient should be kept alert using mental tasks. When water is used, a typical stimulus consists of a 30- to 40-second irrigation with water of 30 degrees C and 44 degrees C using a flow rate of 350 ± 30 ml per minute. Following each irrigation, at least 5 minutes are needed to allow temperature stabilization of the labyrinth. To analyze the eye movement induced by caloric stimulation, a computer calculates the maximum slow component velocity during the eye movement response for each stimulus. This usually is accomplished by computing the average slow component velocity during about 5 to 10 seconds around the time of the peak eye velocity response ( Figs. 32-15 and 32-16 ). If the magnitude of the response to one of the four caloric responses is not in agreement with the responses to the other three, this irrigation should be repeated. Reduced vestibular response (RVR), also sometimes known as labyrinthine asymmetry, and directional preponderance (DP) are calculated using Jongkees’ formula:
RVR = right cold + right warm – ( left cold + left warm ) ( left cold + right cold + left warm + right warm ) DP = left cold + right warm – ( right cold + left warm ) ( left cold + right cold + left warm + right warm )
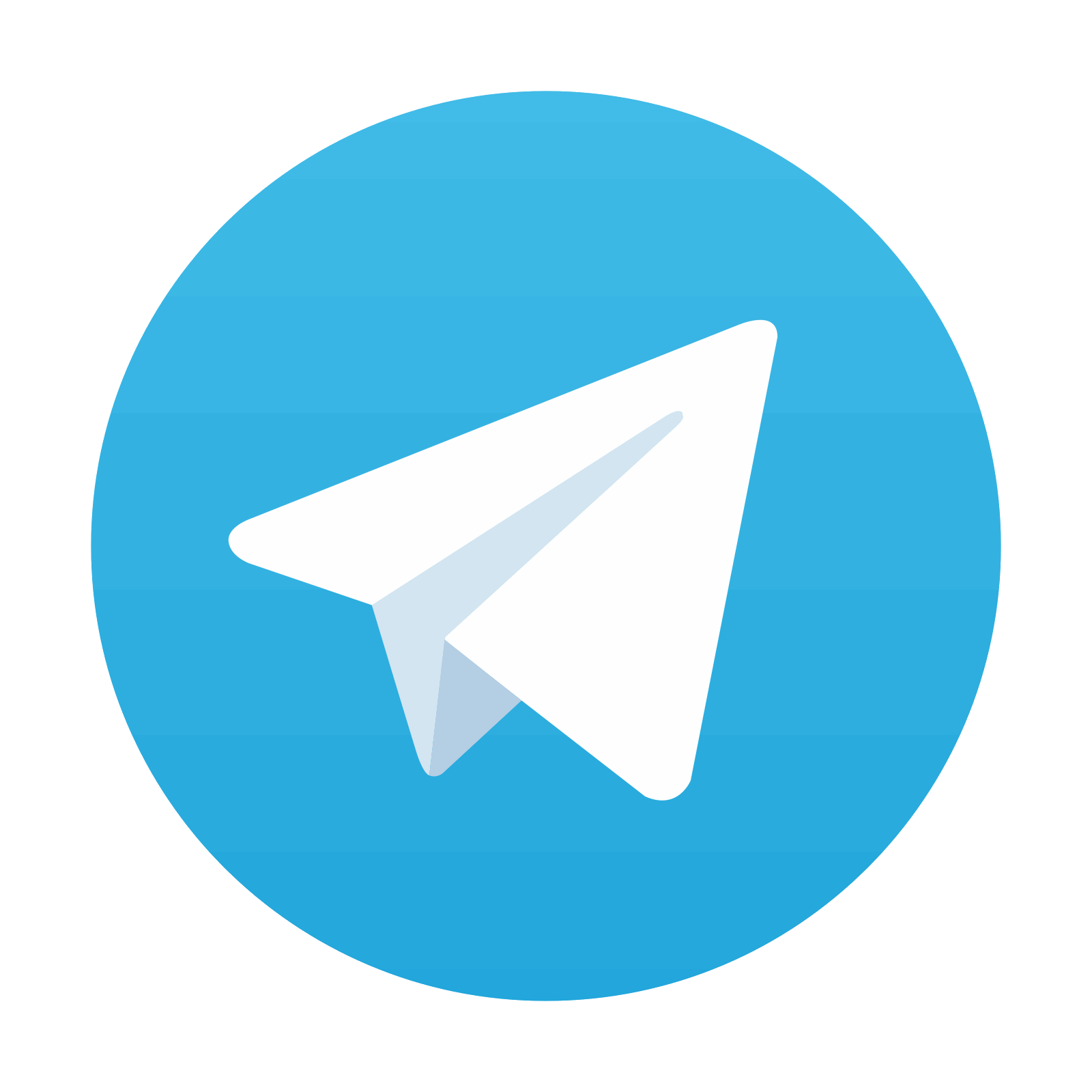
Stay updated, free articles. Join our Telegram channel

Full access? Get Clinical Tree
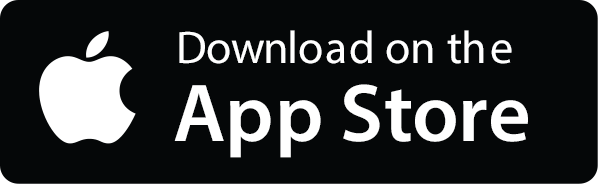
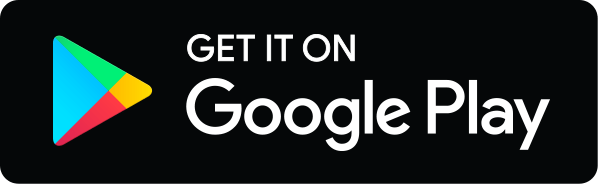