The monoamine neurotransmitters (dopamine [DA], norepinephrine [NE], epinephrine, serotonin, and histamine), the related small molecule neurotransmitter, acetylcholine (ACh), and the neuropeptides, orexin A and B, have an unusual but functionally significant organization in the brain. Their cell bodies are restricted to a small number of nuclei in the brainstem, hypothalamus, and basal forebrain, but their axons project widely throughout the central nervous system. This widely projecting organization permits each of these neurotransmitters to modulate activity in diverse circuits, sometimes in a coordinated fashion.
For example, these systems play critical roles in sleep, arousal, and attention, and in survival responses to relevant stimuli.
Widely projecting DA neurons have their cell bodies in the midbrain, within the substantia nigra pars compacta and the ventral tegmental area. DA is also produced by neurons in the arcuate nucleus of the hypothalamus, and by local circuit neurons in the retina. Midbrain DA neurons project widely to the forebrain and influence motivation, motor behavior, and multiple forms of memory. DA released from the hypothalamus suppresses synthesis and release of prolactin by the anterior pituitary.
NE is synthesized in nuclei within the medulla and pons, the most prominent of which is the locus ceruleus (LC). The LC provides virtually all of the NE to the cerebral cortex. NE regulates arousal, attention, vigilance, and memory. Descending NE fibers modulate afferent pain signals.
Serotonin (5HT or 5-hydroxytryptamine) is synthesized by neurons within the raphe nuclei of the midbrain. Their axons project very widely in the brain to influence diverse circuits involved in arousal, sensory processing, mood, and emotions.
ACh is the neurotransmitter at the neuromuscular junction (nerve–muscle synapses). In the brain it is produced by widely projecting neurons with cell bodies in the brainstem and in the basal forebrain that project to the cerebral cortex and hippocampus. It is also produced by interneurons in the striatum. ACh in the forebrain influences many processes including motivation, learning, and memory.
Histamine is produced by neurons in the tuberomammillary nucleus that lies within the posterior hypothalamus. These neurons project throughout the brain to regulate arousal. Inactivity of histamine neurons promotes sleep. Peripherally, histamine in the stomach promotes secretion of gastric acid via H2 receptors; histamine released from mast cells is involved in allergic responses mediated via H1 receptors.
Orexin A and B (also known as hypocretin 1 and 2) are related neuropeptides that regulate sleep and wakefulness by interacting with monoaminergic and cholinergic neurons that in turn regulate arousal, emotion, motivation, and feeding. Orexin neurons have cell bodies in the lateral hypothalamus, and project widely throughout the brain. Among the major recipients of orexinergic projections are the LC, a source of NE, and the tuberomammillary nucleus of the hypothalamus, the source of histamine.
All receptors for DA, NE, and histamine and 12 of the 13 5HT receptors are G protein–coupled receptors. The 5HT3 receptor is a ligand-gated ion channel. ACh receptors are divided into two major classes: nicotinic receptors, which are ligand-gated ion channels, and muscarinic receptors, which are G protein–coupled receptors. Like most neuropeptide receptors, both orexin receptors, OX1 and OX2 receptors, are G protein–coupled receptors.
The monoamines, DA, NE, and 5HT, share a common vesicular monoamine transporter (VMAT2) that loads them into synaptic vesicles to be stored for release. DA, NE, and 5HT each has a specific plasma membrane transporter in their respective presynaptic terminals that terminates their synaptic actions after release by reuptake into the terminals. The monoamines are metabolized by monoamine oxidase (MAO), which exists in two forms, MAOA and MAOB. The catecholamines, DA and NE, are also metabolized by catechol-O-methyltransferase.
Degeneration of dopaminergic neurons in the substantia nigra causes Parkinson disease; dopaminergic ventral tegmental area neurons play a central role in normal reward-related behavior and in addiction and also in attention and working memory.
DA acts on two related families of G protein–linked receptors, the D2 family (D2, D3, and D4 receptors) that are coupled to Gi/Go and the beta arrestin pathway and the D1 family (D1 and D5 receptors) that are coupled to Gs and the closely related Golf.
Proteins within dopaminergic synapses are targets for several significant classes of drugs. The psychostimulants, cocaine, amphetamine, and methylphenidate, are indirect DA agonists that interact with DA transporters. Parkinson disease is treated with the DA precursor L-dihydroxyphenylalanine (L-dopa), D2 receptor agonists, and monoamine oxidase inhibitors (MAOIs). All currently used antipsychotics are D2 receptor antagonists.
NE interacts with α- and β-adrenergic receptors. Many antidepressants (which are also anxiolytic) increase synaptic NE or 5HT levels by blocking their plasma membrane transporters; others increase synaptic NE or 5HT by blocking metabolism (MAOIs). β-Adrenergic antagonists may dampen memories encoded under strong emotion including trauma.
Proteins within 5HT synapses are targets for the treatment of depression and anxiety. Drugs that block the serotonin transporter are efficacious in obsessive–compulsive disorder (OCD). Many second-generation antipsychotic drugs, such as clozapine and many others, block the 5HT2A receptor, which may help limit Parkinson-like side effects due to concomitant blockade of D2 DA receptors. Hallucinogens, such as LSD, are partial agonists at 5HT2A receptors.
The five different muscarinic ACh receptors can be divided into two families based on the subtype of G protein to which they couple.
Nicotinic ACh receptors (nAChRs) are pentamers constructed of subsets of 12 different subunits. Stimulation of nAChRs at the neuromuscular junction triggers muscle contractions. nAChRs are also found in the brain, where, among other roles, they are targets of the addictive drug, nicotine.
The survival and reproduction of free-living animals requires that they obtain nutrition and hydration, find safety, succeed in mating, and avoid predators and other dangers. Such behaviors require regulation of sleep–wake cycles in response, for example, to the waking times of predators or prey and the predominant environment (such as dark–light cycles and diurnal temperature variation). Successful survival also requires that arousal, vigilance, and attention be regulated appropriately in response to salient stimuli, and that behaviors be selected and executed in accordance with adaptive goals. Likewise, success demands that an organism engage in goal-directed behaviors when the risks and costs warrant it and disengage when the costs are too high. An organism must be able to record in memory the circumstances under which significant threats or rewards are found and learn motor programs that will maximize the speed and efficiency of escape from threats or the attainment of rewarding goals. Many circuits and neurotransmitters are involved in such behaviors, but the neurotransmitters discussed in this chapter play the central coordinating roles. Chapters on arousal and sleep (Chapter 13), higher cognitive function (Chapter 14), mood and emotion (Chapter 15), and reinforcement and addiction (Chapter 16) will develop many of these topics in greater detail, but will depend on the basic information presented in this chapter.
An important goal of neuroscience, often described under the rubric of “connectomics,” is to map the connectivity of animal and human brains, with the ultimate goal being the elucidation of functional maps. Maps of functional activity will depend not only on the locations and connectional patterns of cells, synapses, and circuits but also on the types and strength of synaptic connections, and thus on the “chemical neuroanatomy” that reflects the precise distribution of neurotransmitters, receptors, and their intracellular signaling mechanisms. At the simplest level of generalization, a diversity of important circuits in the brain underlies the detailed information processing and accurate point-to-point communication that permits the brain to create rich sensory representations of the world and to learn, plan, and execute effective motor programs. Such circuits generally depend on excitatory and inhibitory amino acid neurotransmitters acting on rapidly and transiently responsive ligand-gated ion channels (Chapter 5) to achieve spatially and temporally precise communication. Overlaying such circuits are diverse neurotransmitter systems that “fine-tune” their activity in response to salient stimuli, homeostatic needs, and emotional states. These neurotransmitters generally, but not exclusively, produce slower forms of synaptic transmission acting via G protein–linked receptors.
Neurotransmitters exerting such modulatory functions may act at varying distances from their targets. They may act locally, as in the case of cannabinoids and purines (Chapter 8); they may act at intermediate or longer distances, as is the case for some neuropeptides (Chapter 7). A small number of neurotransmitters exhibit a striking organization: they are synthesized by a relatively small number of neurons located within the brainstem, hypothalamus, or basal forebrain. These neurons project very widely throughout the brain, and in some cases descend also into the spinal cord, with some individual axons innervating an astoundingly large number of targets. Such architecture is not consistent with precise information transfer, but rather with the ability to coordinate the responses of many neurons in response to global state changes or significant stimuli. Neurotransmitters with such widely projecting organization include the monoamines (so-called because they contain a single amine group; 6–1), the functionally closely related neurotransmitter acetylcholine (ACh), and the peptide orexin (also called hypocretin). The monoamines include three catecholamines: dopamine (DA), norepinephrine (NE), and epinephrine (E), which are produced from a shared biosynthetic pathway, as well as serotonin (5-hydroxytryptamine or 5HT), and histamine. These neurotransmitters have sometimes been referred to as neuromodulators because their actions via G protein–linked receptors alter the responses of neurons to excitatory and inhibitory amino acids. We discourage the use of the term neuromodulator because many neurotransmitters, including glutamate, GABA, ACh, and serotonin, have both G protein–linked receptors and receptors that are ligand-gated ion channels. Function is determined by the receptor and its associated effector molecules rather than by the neurotransmitter.
DA illustrates the remarkable organization of widely projecting neurotransmitter systems: there are approximately 100 billion neurons in the human brain, but only about 500,000 produce DA. The cell bodies of most of these neurons lie in two contiguous regions of the midbrain, the substantia nigra pars compacta (SNc) and the ventral tegmental area (VTA). This small number of midbrain DA neurons innervates extensive terminal fields within the forebrain. Neurons from the SNc densely innervate the dorsal striatum where they play a critical role in learning and executing motor programs (Chapter 14). Neurons from the VTA innervate the ventral striatum (nucleus accumbens), olfactory bulb, amygdala, hippocampus, orbital and medial prefrontal cortex, and cingulate cortex 6–1. VTA DA neurons play a critical role in motivation, reward-related behavior, attention, and multiple forms of memory (Chapters 14 and 16). This organization of the DA system, wide projection from a limited number of cell bodies, permits coordinated responses to potent new rewards. Thus, acting in diverse terminal fields, DA confers motivational salience on the reward itself or associated cues (nucleus accumbens shell region), updates the value placed on different goals in light of this new experience (orbital prefrontal cortex), helps consolidate multiple forms of memory such as the location and cues of the reward (amygdala and hippocampus), and encodes new motor programs that will facilitate the ability to obtain this reward in the future (nucleus accumbens core region and dorsal striatum). In this example, DA modulates the processing of sensorimotor information in diverse neural circuits to maximize the ability of the organism to obtain future rewards.
6–1
The three major dopaminergic projections in the CNS. (1) The mesostriatal (or nigrostriatal) pathway: the substantia nigra pars compacta (SNc) projects to the dorsal striatum (upward dashed arrows); this is the pathway that degenerates in Parkinson disease. (2) The ventral tegmental area (VTA) projects to the ventral striatum (nucleus accumbens), olfactory bulb, amygdala, hippocampus, orbital and medial prefrontal cortex, and cingulate gyrus (solid arrows). The terms “mesoaccumbens” and “mesocortical” are sometimes used to describe components of the VTA projection. (3) The arcuate nucleus of the hypothalamus projects via the tuberoinfundibular pathway in the hypothalamus, from which dopamine is delivered to the anterior pituitary (downward dashed arrow). In rat brain, the SNc is often still designated as area A9 and the VTA as A10 based historically on maps generated by Dahlstrom and Fuxe using the Falck–Hillarp method, the earliest method to detect monoamine neurotransmitters in the brain. This method is based on autofluorescence of monoaminergic neurons after exposure of brain sections to formaldehyde.

Catecholamines are molecules that contain a catechol nucleus with an ethylamine group attached at the 1 position 6–2. 5HT is an indolamine with a hydroxy group at the 5 position and a terminal amine group on the carbon chain 6–3. The catecholamine neurotransmitters, NE, DA, and E, are sequential products of a single biosynthetic pathway that originates with the amino acid tyrosine 6–4. 5HT and melatonin are synthesized from the amino acid tryptophan 6–5. The catecholamine neurotransmitters and serotonin are discussed together because they have similar widely projecting anatomic organizations, interact with each other functionally, have related mechanisms of clearance from synapses and some shared pathways of metabolism, and are jointly targeted by several important classes of drugs, including psychostimulants (DA, NE, and 5HT), tricyclic antidepressants (TCAs; NE and 5HT), serotonin and NE reuptake inhibitor antidepressants (NE and/or 5HT), and monoamine oxidase inhibitor (MAOI) antidepressants (DA, NE, and 5HT).
6–4
Biosynthetic pathway of catecholamines. Dopamine, norepinephrine, and epinephrine are derived from the multistep processing of tyrosine, a dietary amino acid that is actively transported across the blood–brain barrier and concentrated in catecholaminergic neurons. Region-specific expression of the enzymes shown here determines which neurotransmitters are expressed in a given cell; for example, both dopaminergic and noradrenergic cells express tyrosine hydroxylase (TH) and L-amino acid decarboxylase (AADC), but only noradrenergic cells express dopamine-β-hydroxylase (DBH). The principal metabolites of dopamine and norepinephrine are HVA, VMA, and MHPG. MAO, monoamine oxidase; COMT, catechol-O-methyl transferase; HVA, homovanillic acid; VMA, 3-methoxy-4-hydroxy-mandelic acid (also known as vanillylmandelic acid); MHPG, 3-methoxy-4-hydroxy-phenylglycol.

6–5
Biosynthetic pathway of serotonin and melatonin. Both serotonin, also known as 5-hydroxytryptamine or 5HT, and melatonin are derived from the multistep processing of the dietary amino acid tryptophan. Serotonin is synthesized neuronally in various brainstem nuclei and is converted by monoamine oxidase and aldehyde dehydrogenase into its primary metabolite, 5-HIAA. Serotonin is also produced in cells of the pineal gland, which contain two enzymes—5HT N-acetylase and 5-hydroxyindole-O-methyltransferase—not expressed in serotonergic cells. These enzymes rapidly convert serotonin to melatonin.

Catecholamine biosynthesis begins with dietary tyrosine, which is actively transported into the brain (or peripheral sympathetic neurons; Chapter 9). It is hydroxylated within neurons at the 3 position by the enzyme tyrosine hydroxylase (TH) to form dihydroxyphenylalanine (dopa; known as levodopa orL-dopa). TH requires Fe2+ as a cofactor, as well as molecular oxygen and tetrahydrobiopterin (a hydrogen donor). An inhibitor of TH, α-methylparatyrosine (AMPT), has been used historically as an experimental tool to study catecholamine function; more recent alternatives include mice genetically engineered to lack TH or other enzymes in the biosynthetic pathway.
In dopaminergic neurons, one additional enzyme in this pathway is expressed, L-aromatic amino acid decarboxylase (AADC), which converts dopa to DA. AADC is a cytoplasmic enzyme that requires pyridoxal phosphate, a cofactor derived from vitamin B6. AADC was originally known as dopa decarboxylase until it was recognized that it decarboxylates other substrates, including 5-hydroxytryptophan, the precursor of serotonin 6–5.
In noradrenergic neurons, an additional enzyme, dopamine-β-hydroxylase (DBH), is expressed that catalyzes the conversion of DA to NE. DBH requires Cu2+ and ascorbic acid (vitamin C) as cofactors. DBH is associated with synaptic vesicles that store NE. In the adrenal medulla and in brainstem neurons that produce E, an additional enzyme, phenylethanolamine-N-methyltransferase (PNMT), is expressed that converts NE to E. S-Adenosyl-L-methionine (SAM), a methyl donor, is a required cofactor for this step.
TH, the rate-limiting enzyme in catecholamine synthesis, is regulated by multiple mechanisms. Increased catecholamine release leads to increased TH activity that results from regulation at the transcriptional, translational, and posttranslational levels. Rapid activation of TH activity occurs via its phosphorylation at four serine residues in the N terminus of the protein by several protein kinases, including protein kinase A, Ca2+/calmodulin-dependent protein kinase II (CaM-kinase II), and protein kinase C. It is believed that such phosphorylation induces a conformational change in the protein that results in a higher affinity for its tetrahydrobiopterin cofactor and a lower affinity for catecholamines that trigger end-product inhibition of TH. The end result is an increase in the TH catalytic activity. Longer-term changes in TH activity can occur through transcriptional regulation of the TH gene by extracellular stimuli. Stimuli that upregulate TH expression include chronic environmental stress and drugs such as caffeine, nicotine, and morphine; drugs that downregulate TH expression include many antidepressants (which initially increase synaptic concentrations of NE and/or 5HT).
The ability of tyrosine to penetrate the blood–brain barrier depends on an active transport process. With normal dietary consumption of tyrosine, both active transport and TH activity are fully saturated. Thus, the administration of supplemental tyrosine does not produce significant increases in catecholamine synthesis in the central nervous system (CNS). However, increased catecholamine synthesis can be achieved by peripheral administration of L-dopa, which bypasses this rate-limiting enzymatic step and penetrates the blood–brain barrier, so long as its peripheral metabolism is blocked. For this reason, L-dopa is used in the treatment of Parkinson disease (Chapter 18).
5HT is synthesized from the amino acid tryptophan 6–5, which is actively transported across the blood–brain barrier and hydroxylated by tryptophan hydroxylase (TPH) to produce 5HT. This product is then decarboxylated to form 5HT by AADC, the same enzyme involved in the biosynthesis of catecholamines. In the pineal gland, additional enzymatic steps convert 5HT to melatonin, which is discussed further in Chapter 13.
TPH is the rate-limiting enzyme for 5HT biosynthesis. There are two closely related genes: TPH1, predominantly expressed in the periphery, and TPH2, expressed preferentially in the brain. TPH is subject to short-term and long-term regulatory processes similar to those described for TH, a related amino acid hydroxylase. Like TH, TPH requires molecular oxygen and tetrahydrobiopterin as cofactors, and can be activated by protein kinase A and CaM-kinase II. Although genetic variations in TPH2 have been linked weakly with depression, such findings are not significant in large genome-wide association studies.
An additional member of the amino acid hydroxylase family is phenylalanine hydroxylase, which converts phenylalanine to tyrosine. Mutations of this enzyme that decrease its catalytic activity result in phenylalaninemias (eg, phenylketonuria) that, among other ill effects, can damage the developing brain and cause generalized intellectual disability. Interference with the metabolism of phenylalanine causes the buildup of oxidized derivatives such as phenylketones, which exert toxic effects on neurons. Individuals with such mutations can prevent phenylketonuria by eliminating phenylalanine from the diet, a remarkable example of preventing a genetic disease with an environmental intervention.
Levels of 5HT in the brain can be altered by several means. Drugs such as p–chlorophenylalanine (PCPA), for example, can irreversibly inhibit TPH to produce a long-lasting depletion of 5HT. Experimental manipulation of tryptophan intake also can reduce levels of 5HT in the brain. Individuals who are asked to follow a low-tryptophan diet and subsequently are challenged with a beverage containing other amino acids but lacking tryptophan typically experience not only a dramatic reduction in blood tryptophan levels but also a substantial reduction of 5HT in the brain. In nonhuman primates, where direct measures are possible, a 90% reduction can be achieved. Among patients who have recovered from depression, tryptophan depletion induces a return of depressive symptoms in those who were successfully treated with a selective serotonin reuptake inhibitor (SSRI); however, depressive symptoms do not occur in healthy individuals or in those who were treated with antidepressants that influence NE reuptake. Thus, depletion of 5HT most likely does not cause depression; instead, patients treated with SSRIs may experience withdrawal symptoms on tryptophan depletion that include transient return of depressive symptoms (Chapter 15).
The most significant mechanism by which the synaptic actions of catecholamines, 5HT, and histamine are terminated is by reuptake into the nerve terminal via neurotransmitter-specific transporters expressed on the plasma membranes of presynaptic terminals. In addition, these monoamines are enzymatically catabolized by monoamine oxidase (MAO). The catecholamines, but not 5HT or histamine, are also metabolized by catechol-O-methyltransferase (COMT).
MAO has both intracellular and extracellular forms. The intracellular form is associated with the outer membrane of mitochondria; given that mitochondria are plentiful in presynaptic terminals, the primary action of MAO is to metabolize catecholamines, 5HT, and histamine after they are taken up into presynaptic terminals. However, the extracellular form may also act to metabolize neurotransmitter while in the synapse. Two major forms of MAO have been described: MAOA and MAOB. These forms are derived from distinct genes on the X chromosome and differ with regard to several biochemical properties, including their substrate specificity, cellular localization, and regulation by pharmacologic agents. MAOA mRNA is expressed almost exclusively in noradrenergic neurons, such as those in sympathetic ganglia and locus ceruleus (LC; described below and shown in 6–7). MAOB mRNA is detected predominantly in serotonergic and histaminergic neurons. There are conflicting reports about the expression of MAO genes and protein in dopaminergic neurons, although the evidence favors expression of MAOA.
Both enzymes oxidize monoamines but differ somewhat in their affinity for substrates. MAOA displays a strong affinity for NE and 5HT, even though it is not expressed in serotonergic neurons. The function of MAOB may be not to oxidize 5HT, but rather to metabolize DA or trace amines that might act as false neurotransmitters, such as β-phenylethylamine, for which it has highest affinity. Extracellular 5HT appears to be oxidized by MAOA derived from sources other than 5HT neurons.
MAOIs, such as phenelzine and tranylcypromine, are used to treat depression and anxiety disorders; the MAOIs selegiline and rasagiline are used to treat Parkinson disease (Chapters 15 and 18). However, clinical use of MAOIs as antidepressants and antianxiety agents has been limited by their side effects 6–1. Unsuccessful efforts to link naturally occurring variations in MAO genes with risk for certain complex behaviors are discussed in 6–2.
6–1 Monoamine Oxidase Inhibitors
Most MAOIs, such as phenelzine, tranylcypromine, and isocarboxazid, that have been used clinically are nonselective, blocking both MAOA and MAOB. The first MAOI used in the clinic, iproniazid, was tested in the 1950s as a treatment for tuberculosis. Although it was ineffective against mycobacteria, it relieved the depression that was common among patients hospitalized with TB. Its actions on MAO were only subsequently recognized. Iproniazid is no longer used clinically because it is hepatotoxic, but the other MAOIs proved highly efficacious in the treatment of depression and diverse anxiety disorders. Today, serotonin and norepinephrine reuptake inhibitors are far more widely used clinically because of their superior tolerability (Chapter 15).
MAOs are expressed not only in brain but also in peripheral tissues. MAOA, found in gut and liver, catabolizes biogenic amines present in foods. Some aged or fermented foods, including many wines and cheeses, have particularly high levels of biogenic amines such as tyramine. When MAOA is inhibited, as in response to the therapeutic use of nonselective MAOIs, biogenic amines in foods can enter the general circulation and can be taken up into sympathetic nerve terminals by norepinephrine transporters. This process can lead to the displacement and release of norepinephrine from sympathetic nerve terminals and the release of epinephrine from the adrenal medulla. Such release can produce a hyperadrenergic crisis, which is characterized by headache, hypertension that can be severe, and chest pain. To prevent a potentially dangerous syndrome, individuals who take nonselective MAOIs must eliminate tyramine-containing foods from their diet. Despite their efficacy, it is this complexity of use that has relegated MAOIs to limited clinical utilization.
Because the inhibition of MAOA appears to be required for antidepressant action and also necessitates dietary restrictions, there has been considerable interest in the development of reversible inhibitors of MAOA (so-called RIMAs such as meclobemide). Unfortunately, RIMAs may be less efficacious than other antidepressants.
MAOIs also have been used to treat Parkinson disease. They were initially tested for this purpose after investigators discovered that the dopamine neurotoxin, 1-methyl-4-phenyl-1,2,3,6-tetrahydropyridine (MPTP), which can cause Parkinson disease, must be converted to MPP+ by MAOB before it can exert its toxic effects. MPTP was discovered when an illicit drug laboratory, attempting to make the opiate meperidine, left MPTP as a contaminant. The individuals who injected it became acutely and severely Parkinsonian and were found to have destroyed their SNc dopamine neurons, likely by extreme oxidative damage. As a result, the MAOB-selective inhibitors selegiline (also known as deprenyl) or rasagiline were administered to patients with early Parkinson disease in clinical trials as a putative neuroprotective agent (presumably preventing the activation of endogenous or exogenous MPTP-like neurotoxins). Although these drugs are efficacious, their mechanism of action remains unclear; their modest benefits might be related to their ability to increase levels of synaptic dopamine rather than to any putative neuroprotective effect.
6–2 Alleged Association of the MAOA Gene With Aggression: Lessons for Psychiatric Genetics
In a large Dutch family, multiple males with mild intellectual disability and aggression were found to have a loss-of-function mutation in the MAOA gene. Given the small numbers involved in a single family, and the complexity of the aggression phenotype, causality could not be proved. Nonetheless, a large number of studies have investigated possible associations between MAOA gene variants with aggression and antisocial behavior. These have extended beyond simple association studies to studies of gene–environment (G × E) interactions in which an MAOA gene variant that results in lower MAOA levels than other common variants is associated with aggression conditional on a history of childhood abuse. Despite the inconclusive nature of these studies, they have influenced findings of culpability in the sentencing phase of murder trials. In 2009 and 2011, two separate Italian courts reduced the duration of prison sentences based in part on the finding that the defendants had the low-activity allele of MAOA presumed to increase risk of reactive aggression given childhood histories of maltreatment.
From a genetics perspective, the existing evidence that attempts to associate a complex behavioral phenotype either with a single genetic variant or with a G × E interaction remains unconvincing. Except for extreme behavioral phenotypes (typically characterized by severe intellectual disability), in which single genes can explain a significant fraction of the variance, mental illnesses and normal behavioral variation have proven to be genetically highly complex. The study of environmental risk has generally relied on epidemiologic studies that have identified risk factors (eg, child maltreatment) that are often associated with increased probability of many negative outcomes, and where the causal mechanisms are yet to be understood. Given these complexities, there are no studies of candidate genes or environments related to aggression that have been adequately powered to test the association hypothesis.
Perhaps the most important question to ask in judging the relevance of a putative association between a particular allele and a disease phenotype or behavior is how much of the phenotypic variance it explains (relative to other genetic and nongenetic factors), and what is the magnitude of the effect in a given individual. For virtually all candidate gene and G X E studies in psychiatry (eg, variants in MAOA, the 5HT transporter, and COMT) significance is fragile at best, and even if the associations survive methodologically stronger studies, their effect size is likely to be very small and to be swamped by the aggregate influence of many other factors. With the advent of large-scale genetic studies of mental illness and eventually other behavioral phenotypes (assuming that they can be objectively defined), it is at long last becoming possible to identify bona fide risk genes for some of these conditions (see Chapters 14 and 17 for further discussions related to autism, schizophrenia, and bipolar disorder).
Catecholamines are also catabolized by COMT. Peripherally the major isoform is soluble, but, in the brain, a longer, membrane-bound isoform predominates, which is found in catecholamine synapses. COMT methylates catecholamines using S-adenosyl-methionine as a methyl donor. COMT inhibitors, such as entacapone and tolcapone, increase levels of catecholaminergic neurotransmitter in synapses and prolong receptor activation. In general, COMT appears to play a far smaller role in terminating the synaptic action of DA and NE than their specific membrane transporters, but in the prefrontal cortex, where the dopamine transporter (DAT) is expressed at relatively low levels, COMT may exert a more significant effect.
The major products that emerge from the enzymatic breakdown of catecholamines by MAO and COMT are shown in 6–4. Historically, these metabolites were investigated as indirect measures of brain catecholaminergic function in depression and schizophrenia. They were measured in cerebrospinal fluid, blood, and urine; however, interpretation of metabolite levels was significantly confounded by activity of the sympathetic nervous system and adrenal medulla and by many other factors. Thus, their usefulness as markers of CNS catecholamine function proved quite limited. 5HT metabolites were also historically investigated. After 5HT is oxidized by MAO, aldehyde dehydrogenase acts to produce 5-hydroxyindoleacetic acid (5-HIAA) as an end product 6–5. Reduction of 5-HIAA in cerebrospinal fluid has been reported to correlate with impulsive violence in some circumstances, most notably among individuals who have attempted suicide by violent means. Despite considerable research, the significance of these findings remains unclear. Overall, it may have been naïve to believe that major neuropsychiatric disorders reflected global abnormalities in levels of one neurotransmitter, rather than disorders of more specific neural circuits that could be influenced by DA, NE, or 5HT.
The overall anatomy of DA in the brain is shown in 6–1. Historically, the first recognition of the importance of DA in the brain came from the investigation of Parkinson disease, which results from degeneration of SNc DA neurons. Death of these neurons results in denervation of the neostriatum (composed of the caudate and putamen in the human brain and the dorsal striatum in the rat and mouse brains), resulting in a movement disorder characterized by tremor, rigidity, abnormal gait, and difficulty initiating voluntary movement (Chapter 18). The major strategy for treatment is DA replacement, but DA is polar and cannot penetrate the blood–brain barrier. Instead, L-dopa is administered. After it crosses the blood–brain barrier it is taken up by dopaminergic nerve terminals via DAT and subsequently converted into DA by AADC 6–6. However, because AADC also resides in peripheral tissues, a significant fraction of L-dopa is decarboxylated into DA before it can be transported into the brain. Among other side effects, this produces nausea because there are DA receptors in the area postrema of the medulla, an area of the brain that controls nausea and vomiting, and which lies outside the blood–brain barrier so that it can sample the systemic environment (Chapter 2). L-Dopa is thus coadministered with an AADC inhibitor such as carbidopa that cannot penetrate into the brain.
6–6
Model of a dopaminergic synapse. Presynaptic and postsynaptic molecular entities involved in the synthesis, release, signaling, and reuptake of dopamine are shown. Although MAO is shown extracellularly, it also exists in glia proximate to DA synapses and possibly in mitochondria within dopaminergic nerve terminals. VMAT, vesicular monoamine transporter; Gi and Gs, inhibitory and stimulatory guanine nucleotide–binding proteins; MAO, monoamine oxidase; COMT, catechol-O-methyltransferase; HVA, homovanillic acid; AC, adenylyl cyclase.

Given the role of DA receptors in vomiting, D2 antagonists are effective antiemetic medications. However, due to their risk of causing Parkinson-like side effects, alternatives are often used. 5HT3 antagonists such as ondansetron, and neurokinin 1 (NK1) receptor antagonists (the NK1 receptor recognizes the neuropeptide, substance P; Chapter 7) such as aprepitant, are effective antiemetics and lack the side effects of D2 receptor antagonists.
VTA projections to the limbic forebrain, as noted earlier, are required for reward-related behaviors. VTA DA projections also form the substrate on which certain drugs produce addiction, which is described in detail in Chapter 16. Under normal circumstances VTA DA neurons fire prior to behaviors that are elicited by cues predictive of reward. In order to coordinate responses to such cues, the VTA receives inputs from diverse brain regions. These include reciprocal inputs with regions involved in valuation of rewards and also inputs from other widely projecting systems involved in arousal, attention, and memory. Different subregions of the VTA receive glutamatergic inputs from the prefrontal cortex, orexinergic inputs from the lateral hypothalamus (see 6–25), cholinergic and also glutamatergic and GABAergic inputs from the laterodorsal tegmental nucleus and pedunculopontine nucleus 6–18, noradrenergic inputs from the LC 6–7, serotonergic inputs from the raphe nuclei 6–10, and GABAergic inputs from the nucleus accumbens and ventral pallidum. The activity of VTA DA neurons is also suppressed by activation of the lateral habenula, which sends glutamatergic inputs to GABAergic neurons in the vicinity of the VTA, which normally inhibit VTA DA neurons.
6–7
Noradrenergic projections in the CNS. The locus ceruleus (LC), which lies in the rostral pons, is the largest noradrenergic nucleus. It provides virtually all norepinephrine (NE) to the cerebral cortex and innervates many other forebrain areas (thick arrows). Other sources of NE come from clusters of cells within other brainstem nuclei. In the rat, the LC is often designated as area A6 and the other noradrenergic brainstem nuclei as A1, A2, A5, and A7, based on the Falck–Hillarp method of mapping (see 6–1).

DA has multiple actions in the prefrontal cortex. It promotes the “cognitive control” of behavior: the selection and successful monitoring of behavior to attain chosen goals. Aspects of cognitive control in which DA plays a role include working memory, the ability to hold information “on line” in order to guide actions, suppression of prepotent behaviors that compete with goal-directed actions, and control of attention and thus the ability to overcome distractions (Chapter 14). Cognitive control is impaired in several disorders, including attention deficit hyperactivity disorder (ADHD), which is treated with psychostimulants, a term used to describe indirect DA agonists such as methylphenidate and amphetamines that block DAT or cause reverse transport of DA into synapses. Cognitive control is also deficient in addiction and a range of poorly described conditions known as impulse control disorders. Working memory is impaired in schizophrenia, to a lesser degree in some nonpsychotic relatives of individuals with schizophrenia, and in patients with bipolar disorder with psychotic symptoms (Chapter 17). Antipsychotic drugs do not have a therapeutic benefit for working memory or for any of the other cognitive impairments of schizophrenia.
Antipsychotic drugs produce their therapeutic effects (diminishing psychotic symptoms, eg, delusions and hallucinations) by blocking D2 receptors, presumably those that reside in the terminal fields of VTA DA neurons in subcortical structures in the limbic forebrain. The mechanism by which reducing dopaminergic stimulation of these structures ameliorates psychotic symptoms is unclear. Because antipsychotic drugs block D2 receptors in the caudate and putamen as well as in the neurons where they exert therapeutic effects, these drugs produce side effects that are similar to the symptoms of Parkinson disease. (These are often called extrapyramidal side effects to distinguish striatally based motor systems from the corticospinal motor system, the fibers of which descend in a brainstem structure called the “pyramids.”) Because these are “on-target” side effects, that is, side effects that arise from the same molecular target—the D2 DA receptor—that is required for their therapeutic effects, attempts to mitigate the side effects must depend on stimulation or blockade of other receptors. Antipsychotic drug–induced Parkinson-like effects are not treated with L-dopa or indirect DA agonists because D2 DA receptors are already blocked and, were DA or DA agonists to successfully compete for D2 DA receptors, the likely result would be worsening of psychotic symptoms. Instead extrapyramidal side effects are treated with anticholinergic drugs (ie, drugs that block muscarinic cholinergic receptors). Clozapine and second-generation antipsychotic drugs have less tendency to produce these side effects than first-generation drugs partly as a result of lower affinity for D2 receptors, but also as a result of having other receptor actions, including antagonism of 5HT2A receptors, antagonism of muscarinic cholinergic receptors, or some combination (Chapter 17).
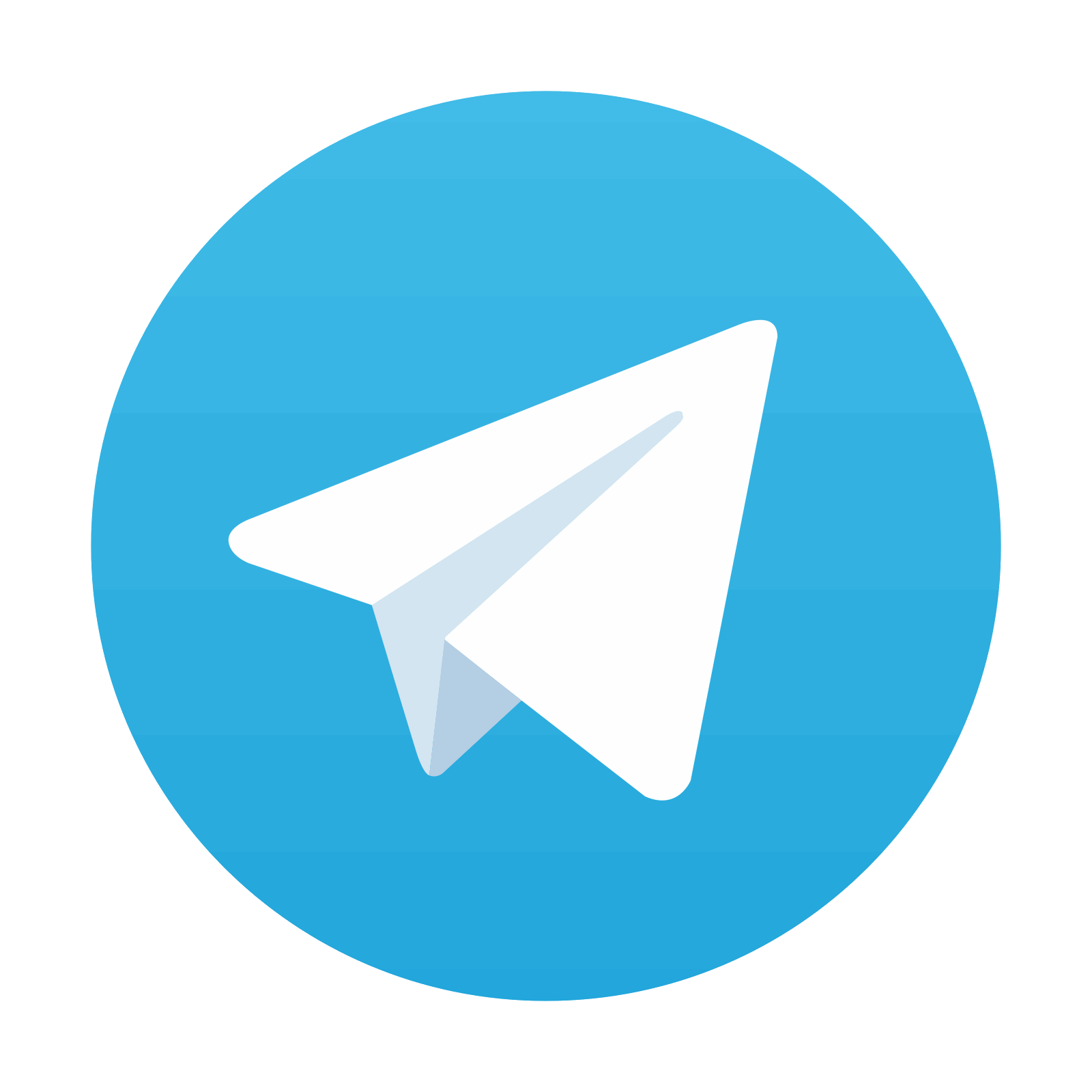
Stay updated, free articles. Join our Telegram channel

Full access? Get Clinical Tree
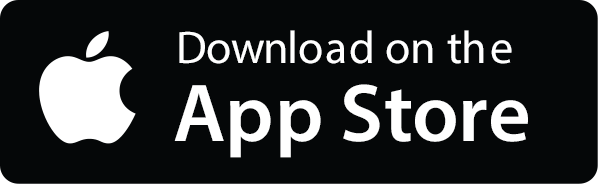
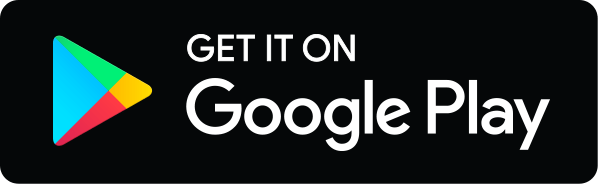