The Localization of Lesions Affecting the Ocular Motor System
Abnormalities of ocular motility serve as valuable signposts for the localization of lesions of the cerebral hemispheres, brainstem, cranial nerves, and even striated muscle. Ocular symptoms and signs are particularly helpful when examining the patient in coma (see Chapter 22).
In this chapter, the term ocular motor refers to cranial nerves III (oculomotor), IV (trochlear), and VI (abducens), collectively, whereas the word oculomotor designates specifically cranial nerve III. This follows the convention adopted by Leigh and Zee [565] in their excellent review of ocular motility.
We first review the anatomy and localization of lesions of the ocular motor nuclei and nerves and then the control systems that the brain uses to produce precise, smooth, quick, stable, and binocular eye movements. Disturbances of these “supranuclear” mechanisms, nystagmus, and eyelid disturbances are then discussed.
Ocular Motor Muscles and Nerves
Orbital Muscles
Each eye globe is moved by six muscles: four recti (superior, inferior, medial, and lateral) and two oblique (superior and inferior). The horizontal recti arise from the annulus of Zinn and course along the medial and lateral orbital walls. The action of the medial (eye-in) and lateral (eye-out) recti requires no further comment. The superior rectus muscle originates from the annulus of Zinn, courses anteriorly upward over the eyeball, and laterally, forming an angle of 23 degrees with the visual axis of the eye. This muscle elevates the eye (displaces the cornea upward) when the eye is deviated outward (abducted) (Fig. 8.1). Likewise, the inferior rectus arises from the annulus, courses anteriorly downward and laterally forming an angle of 23 degrees with the visual axis, and primarily depresses the eye most efficiently when the globe is abducted. By contrast, when the eye is adducted (turned inward), the superior rectus intorts it (moves it counterclockwise in the case of the left eye), and the inferior rectus extorts it (moves the left eye clockwise). The superior oblique muscle arises from the annulus of Zinn and passes anteriorly and upward along the superomedial wall of the orbit, becoming tendinous before passing through the trochlea located on the nasal side of the superior orbital rim. The tendon is then reflected inferiorly, posteriorly, and laterally, forming an angle of 51 degrees with the visual axis. It passes inferior to the superior rectus and inserts in the posterosuperior quadrant of the eyeball. The inferior oblique muscle originates from the periosteum of the maxillary bone, just posterior to the orbital rim, and passes laterally, superiorly, and posteriorly coursing underneath to the inferior rectus muscle and under the lateral rectus (at an angle of 51 degrees with the visual axis) to insert in the posterolateral portion of the globe. The oblique muscles have a similar but complementary action in moving the eyes in the vertical plane. They move the eyeball in a vertical plane when the eye is adducted and act as rotators when it is abducted. Unlike the recti, however, the oblique muscles function as would be expected from the location of their insertional points in the anterior part of the orbit and in the posterior part of the globe. The superior oblique depresses the eye or twists it inwardly (counterclockwise by examiner’s view in the case of the left eye) and the inferior oblique elevates the eye or extorts it when abducted (moves the left eye clockwise by examiner’s view).
Two muscles, both in the upper eyelid, act together to widen the palpebral fissure. Müller’s muscle receives sympathetic innervation and is responsible for the wide stare that accompanies states of enhanced alertness. However, the levator of the lid, innervated by cranial nerve III, plays the greater role in eyelid opening. Eye closure (orbicularis oculi) is effected through cranial nerve VII.
Diplopia
The commonest subjective complaint elicited by lesions in the ocular motor system is diplopia [140,141]. This disorder occurs more frequently with lesions of the extraocular muscles or ocular motor nerves than with supranuclear brainstem lesions, which often result in gaze palsies. Diplopia results from lack of visual fusion. The perceived object is projected to noncorresponding points of the retina and is therefore seen as two objects. Often, particularly when the defect is mild, the patient sees a blurry, rather than a double, image.
FIG. 8.1. Superior view of the right orbit. A. On abduction, the superior rectus acts as an elevator, and the superior oblique intorts the eye (brings the upper pole toward the nose). B. In adduction, the superior oblique acts as a depressor, and the superior rectus intorts the eye. In (B), the superior rectus has been removed to show the position of the superior oblique.
Diplopia that is present monocularly remains present despite covering the fellow eye and then disappears when the involved eye is occluded. It may occur unilaterally or bilaterally. The second image is often described as a less clear and partially superimposed “ghost image” or a “halo” on the first image. A pinhole may dramatically reduce the patient’s symptoms. Monocular diplopia usually implies a problem within the eye itself and may respond to refraction, artificial tear trial, or contact lens trial. Etiologies of monocular diplopia include corneal and lenticular astigmatism and opacities, a foreign body in the aqueous or vitreous media, retinal disease, ocular surgery, strabismus, or psychiatric disease and are listed in Table 8.1.
TABLE 8.1 Ocular Causes of Monocular Diplopia
Another form of monocular diplopia is cerebral polyopia [254,413,460,615,850]. Cerebral polyopia usually can be distinguished from monocular diplopia due to ocular disease because all of the images are seen with equal clarity, the multiple images do not resolve with a pinhole, and the images are unchanged in appearance whether the patient is viewing binocularly or monocularly. Some patients see only two images, while others may see many or even hundreds of images occurring in a grid-like pattern (“entomopia” or “insect eye”) [586]. Some patients experience the polyopia only in certain positions of gaze. Patients with cerebral polyopia often have associated signs of occipital or parietooccipital region damage, such as homonymous visual field defects, difficulty with visually guided reaching, cerebral achromatopsia or dyschromatopsia, object agnosia, and abnormal visual afterimages. The multiple images often appear in the field opposite the lesion. Cerebral infarction is the most common etiology, although cerebral polyopia may also occur with tumors, multiple sclerosis, encephalitis, seizures, and migraine [460].
Diplopia can sometimes occur without extraocular muscle abnormality. For example, the hemifield slide or slip phenomena is a rare cause of intermittent binocular diplopia noted in some patients with lesions of the optic chiasm [513]. This phenomenon occurs with complete or nearly complete bitemporal hemianopic visual field defects (see Chapter 7) with disruption of ocular fusion and decompensation of a previous phoria. The underlying pathophysiology is loss of binocularity due to lack of cortical representation of corresponding points in the visual field from each eye (i.e., transection of the chiasm creates two independent, free-floating hemifields, with each eye projecting only to the ipsilateral visual cortex). Patients complain of intermittent diplopia and difficulty with near work (e.g., threading a needle or sewing). On examination, no ocular motor palsy is noted despite the patients’ complaints of diplopia. If the eyes intermittently converge (esotropia), a blank space is produced between the vertical meridians as the eyes “slip” inwards and the remaining hemifields drift apart horizontally. Ocular divergence (exotropia) causes overlapping of the vertical meridians resulting in superimposition of images from noncorresponding retinal areas. Hemifield slide diplopia may also occur from altitudinal visual field defects [120].
Testing for Diplopia
Before diplopia testing is undertaken, it is important to ascertain visual acuity in both eyes and to inspect them for lens or retinal displacements. A forced duction test may be done to determine whether the restriction of eye movement is due to muscle weakness or to mechanical restriction in the movement of the globe. A positive forced duction test (i.e., restriction of eye movement on attempted forced ductions) may be seen with thyroid eye disease, extraocular muscle fibrosis, muscle entrapment (e.g., blowout orbital fracture), Duane retraction syndrome, Brown’s superior oblique tendon sheath syndrome, carotid-cavernous sinus or dural fistula, and direct orbital infiltration by inflammatory processes or tumors.
Horizontal binocular diplopia is usually due to disease processes affecting the medial and/or lateral rectus muscles, the innervation of these muscles (including ocular motor cranial nerves and neuromuscular junction), or processes affecting fusion or convergence and divergence mechanisms [141]. By definition, patients with horizontal diplopia complain that the two images are side by side. The separation of images may vary or remain unchanged at far or near fixation. For example, the image separation from a left abducens nerve palsy is typically worse at distance than at near and worse on left gaze. Patients with vertical diplopia complain of seeing two images, one atop or diagonally displaced from the other [140]. If the patient complains of vertical diplopia in primary gaze, often one of the vertically acting extraocular muscles is underacting: the right and/or left inferior rectus, superior rectus, inferior oblique, or superior oblique. Etiologies of horizontal and vertical diplopia are listed in Tables 8.2 and 8.3, respectively.
To identify the muscle or nerve involved, subjective and objective tests should be used. Greater difficulty with near vision suggests impairment of the medial rectus muscle, oculomotor nerve, or convergence system. Abducens weakness results in horizontal diplopia when viewing distant objects. Vertical diplopia becomes worse on near vision when an oblique muscle is weak. Misalignment (deviation) of the visual axis when only one eye is viewing is referred to as a phoria. Misalignment of the visual axis with both eyes viewing is called a tropia. When strabismus (misalignment of the visual axes) exists, it is named by the direction of deviation: if the eyes turn in, esodeviation; if the eyes turn out, exodeviation; if one eye is down, hypodeviation; if one eye is up, hyperdeviation; and if one eye is torted, cyclodeviation. Strabismus may be comitant (the deviation is stable in nonextremes of gaze) or incomitant (the deviation varies in different gaze positions). Eye deviations from childhood strabismus are typically comitant while most of the acquired deviations are incomitant.
A phoria is a latent ocular misalignment that is kept in check by fusion. Fusion is the process of merging images from each eye into a single perception. Sensory fusion is the cortical integration of two images, while motor fusion represents the corrective movements of the eyes required to maintain eye alignment on the target of regard. Breakdown of fusion due to fatigue, stress, illness, etc. may allow a pre-existing phoria to become an intermittent or manifest tropia.
SUBJECTIVE TESTING
Although a peripheral nerve or muscle may be so severely affected that the diagnosis of ocular motor paresis can be made by mere inspection of the position of both eye globes in the orbit, often the objective findings are subtle and subjective diplopia testing is helpful. To ascertain which image belongs to which eye, a red glass may be placed in front of one eye, or each eye may be covered and uncovered alternately. Instead of a red glass, a simple instrument, the Maddox rod, can be used to separate the two images even more conclusively [565]. Two rules are then applied: First, image separation is greatest in the direction of the weak muscle. Second, in the position of greatest image separation, the image seen more peripherally corresponds to the eye with poorer motility. Paresis of the lateral or medial recti can be easily diagnosed using these rules. Vertical gaze palsies can also be diagnosed in this manner, taking into account the actions, described above, of the eight muscles that participate in vertical movement and the fact that with vertical deviation, the hypotropic eye registers the higher image. The following steps are often recommended:
1. Determine which eye is higher. If the right eye is higher (right hypertropia), the right depressors or the left elevators are weak.
2. Have the patient look to both sides. If the images now appear farther apart on left lateral gaze (right hypertropia worse on left gaze), either the right superior oblique or the left superior rectus is weak.
3. Have the patient look up and down toward the side where separation was greater. If this maneuver elicits greater separation on downgaze, the right superior oblique is the weak muscle.
TABLE 8.2 Etiologies of Esotropia/Exotropia and Acquired Horizontal Diplopia
These steps can be supplemented with Bielschowsky head-tilt test, which is usually positive with an oblique muscle palsy. Tilting the head toward the side of a weak superior oblique increases the separation of the images, which become single when the head is tilted opposite the side of the weak muscle. Normally a small counter-rolling of the eyes occurs with a head tilt. This counter-rolling is accomplished by contraction of the superior rectus and superior oblique of one eye and by the inferior rectus and inferior oblique of the other eye. When the action of the superior oblique muscle on one side is lacking, tilting toward that side results only in superior rectus contraction, which elevates and intorts the eye due to the induced ocular counter-rolling. Diplopia caused by a right superior oblique palsy is compensated by a leftward tilt of the head. Thus, a vertical muscle palsy must be suspected in patients with a head tilt.
OBJECTIVE TESTING
Objective diplopia testing is used when the patient is uncooperative or when there is misalignment of the eyes, suggesting ocular motor weakness, but the patient denies diplopia. This situation occurs more often with long-standing ocular motor weakness or with defects of visual perception. It may rarely occur with subacute lesions, however, when the image of the nonfixating eye is suppressed. In this instance, the patient may give a history of transient diplopia.
A tropia is a manifest deviation of the eyes and is diagnosed by the cover–uncover test. The patient fixate on a target and then each eye is covered and uncovered. The patient is then observed for a shift in the noncovered eye. An absence of shift means there is no tropia (the patient is orthotropic) while a shift of the noncovered eye indicates the presence of a tropia (e.g., if the eye shifts inward, there is an exotropia). The cover–uncover test requires good enough vision to fixate on a target. The degree of deviation may be quantified with prisms with apex of prism aimed opposite to the direction of movement (base-out if esotropia)
TABLE 8.3 Etiologies of Binocular Vertical Diplopia and Hypertropia/Hyperphoria
If the cover–uncover test is negative, no tropia is present. A phoria is a latent deviation of the eyes kept in check by visual fusion. The alternate cover test breaks down fusional control and a latent deviation may be uncovered. The alternate cover test is performed by having the patient fixate on a target in each of the nine positions of gaze. While in each position, the eyes are observed for angular deviations from the direction of the target as each eye is alternately covered (Fig. 8.2). In a patient with a right third nerve palsy, both eyes remain parallel on gaze to the right, but on left lateral gaze the uncovered eye is directed toward the target, while the covered eye is at an angle to it. When the right eye is covered and the left eye fixates, the right eye deviates outward (exophoria), and when the cover is placed over the left eye, the right eye moves inward (movement of redress) and the left eye moves outward. The deviation of the paretic eye, termed primary deviation, is smaller than the deviation of the sound eye, called secondary deviation. In the example mentioned above of a right third nerve palsy, it is logical that, when the eyes look to the left, the outward deviation of the covered left eye when the right eye fixates is greater than it is when the cover is switched, because a strong leftward gaze movement has to compensate for the weakened right medial rectus to allow the right eye to fixate. These deviations elicited by the alternate cover test may be measured with prisms, with the prism “pointing” toward the deviation (e.g., with a hypertropia, a base-down prism is placed over the affected eye, whereas with an esodeviation, a base-out prism is placed over the affected eye). The four-stage procedure noted above to investigate vertical deviation may incorporate the alternate cover test to determine the hypertropic eye in the test directions, including head tilt (the Bielschowsky head-tilt test).
FIG. 8.2. Alternate cover test in a right medial rectus palsy. A. When the right (weak) eye fixates, the left is drawn in the direction of gaze (secondary deviation, D2) and is deviated from the orientation to the target (bold line). B. When the right eye is covered, the left eye is oriented to the target, having covered an angle equal to the amount of the secondary deviation (D2). However, the right eye now drifts toward midposition, deviating (primary deviation, D1) from the orientation to the target.
The three-step test can be applied with the cover–uncover examination in the evaluation of vertical diplopia:
1. Determine whether there is a right or left hypertropia or hyperphoria in primary position. For example, if there is a right hypertropia in primary position, there is paresis of the right eye depressors (right inferior rectus or superior oblique) or left eye elevators (left superior rectus or inferior oblique).
2. Compare the amount of vertical deviation in right and left gaze. For example, if the right hypertropia increases in left gaze, either the right superior oblique or left superior rectus is underacting.
3. Compare the vertical deviation in right head tilt and left head tilt (Bielschowsky maneuver). For example, if the vertical deviation increases with right head tilt, the right superior oblique must be weak; if the hyperdeviation increases on left head tilt, the left superior rectus is weak.
Ductions (each eye moving separately) and versions (the eyes moving conjugately) must always be assessed. In assessing normal lateral eye excursion, an imaginary vertical line through the lower lacrimal punctum should coincide with a boundary line between the inner third and outer two-thirds of cornea. If more cornea is hidden, adduction is excessive; if more cornea is visible and if some sclera visible, adduction is limited. If abduction is normal, the corneal limbus should touch the outer canthus. If the limbus passes that point and some of cornea is hidden, abduction is excessive; if some of the sclera remains visible, abduction is limited [972].
Patients with binocular vertical diplopia may adopt a compensatory head, face, or chin position to move their eyes into a gaze angle that achieves binocular single vision. Underaction of the superior or inferior rectus muscles is compensated by chin flexion or extension that seeks to avoid the eye position of maximum image separation. Torsional diplopia is usually caused by underaction of the superior or inferior oblique muscles and may be associated with an angular head tilt. This head tilt is assumed to avoid the vertical and torsional image separation.
Ocular torsion may be measured with the double Maddox rod test that utilizes a red Maddox rod over the right eye and a white Maddox rod over the left eye in a trial frame. A thin base-down prism may be placed before one eye to separate the horizontal lines induced. The tilt of the retinal image is opposite to the tilt of the horizontal line, as seen by the patient. Therefore, when the line is seen slanted toward the nose, an excyclodeviation is present while if the tilt is toward the temple, an incyclodeviation is present. A simple mnemonic rule is that the line is always tilted in the direction in which the offending muscle would rotate the eye if it were acting alone [972]. For example, a patient with right superior oblique muscle palsy will describe the red line to be lower than the white line and relatively intorted or slanted toward the nose. The Maddox rod is then turned until the two lines are parallel and the magnitude of the cyclotropia can be read off the trial frame. Cyclodeviation may also be noted with indirect ophthalmoscopy [972].
Childhood Strabismus
These syndromes (Table 8.4) will be briefly discussed as they may be confused with acquired causes of esotropia (ET) and exotropia (XT) [972]. Most childhood esotropias are comitant and present at an early age with “crossed-eyes” or amblyopia. Childhood comitant esotropias may be due to hyperopia or impaired accommodation or convergence. Noncomitant childhood esotropias include A-pattern and V-pattern esodeviations, in which the esodeviation is worse on upward and downward gaze, respectively, retraction syndromes, and mechanical-restrictive esodeviation due to congenital fibrosis of the medial rectus muscle. Some patients with congenital nystagmus are able to decrease the amplitude or frequency of their nystagmus by convergence (nystagmus blockage syndrome) and thus an esotropia develops.
TABLE 8.4 Classification of Childhood Strabismus Syndromes
Occasionally, adults with a longstanding, essentially asymptomatic, esophoria may present with diplopia due to “decompensation.” This decompensation of a long-standing esophoria may occur after head trauma, with changing refractive needs, after cataract surgery, when the patient receives drugs that depress the central nervous system (e.g., alcohol or sedatives), with systemic illnesses, or for an unclear reason. History and examination often reveal supportive evidence for a long-standing strabismus, including a history of childhood strabismus or patching, the presence of an old head turn, and horizontal comitance. Childhood exotropia is less frequent than childhood ET. The XT may be intermittent or persistent and sometimes adults with exophoria or intermittent XT may present with diplopia due to inability to adequately compensate for the eye misalignment (decompensation of exophoria).
Decompensation of a longstanding phoria may also cause hypertropia and vertical diplopia [158]. Neuro-ophthalmologic history and examination often reveal supportive evidence for a longstanding strabismus including a history of childhood strabismus or patching, the presence of a head tilt or turn (old photos), and large vertical fusional amplitude (6–20 prism diopters). Vertical fusional amplitudes are measured by presenting vertically oriented prisms of gradually increasing strength in front of one eye after first neutralizing any manifest tropia. The amount of prism needed to produce diplopia over that needed to neutralize the tropia (if present) represents the fusional amplitude (normal vertical fusional amplitudes are 2 to 4 prism diopters).
Sensory deviations including ET or XT result from reduced visual acuity in one eye. These patients do not complain of diplopia because of the visual loss. Loss of fusion in cases of visual loss allow a pre-existing phoria to become manifest. Sidikaro and von Noorden reported 121 patients with sensory heterotropias and noted that ET and XT occurred with almost equal frequency when the onset of visual impairment occurred at birth or between birth and 5 years [846]. Sensory XT, however, predominates in older children and adults.
Disease of the Ocular Muscles
Disease of isolated extraocular muscles, particularly when it affects the lateral rectus or the superior oblique or causes patterns of weakness that resemble central involvement, may be difficult to differentiate from neurogenic weakness. Processes that limit the range of motion of the globe by shortening or fibrosis of the muscles, such as old trauma or chronic progressive ophthalmoplegia, can be distinguished from neurogenic weakness because the forced duction test, described above, is normal in neurogenic weakness.
A mechanical restriction to free movement of the superior oblique tendon at the pulley may prevent the upward and inward movement of the globe (Brown’s superior oblique tendon sheath syndrome) [972,993]. Episodic vertical diplopia results due to intermittent trapping of the eye on gaze downward and inward or in the field of action of the superior oblique, thus mimicking paresis of the inferior oblique muscle. This prevents the eye from moving upward while adducted, imitating an isolated inferior oblique muscle palsy. The eye may then release suddenly, occasionally associated with the sensation or actual hearing of a click. This syndrome may be due to swelling of the superior oblique tendon behind the pulley and may be congenital or acquired. Acquired etiologies include superomedial orbital trauma, tenosynovitis or myositis, adhesions, metastasis to the superior oblique muscle, peribulbar anesthesia, blepharoplasty, frontal sinus osteoma, ethmoid sinus mucocele, pansinusitis, psoriasis, peribulbar anesthesia, blepharoplasty, implantation of an Ahmed valve glaucoma implant and maxillo-facial or sinus surgery [23,60,275,810,911]. In congenital cases, MR imaging may show enlargement of the tendon-trochlea complex with this complex being of irregular shape and of intermediate signal intensity [827]. The superior oblique click syndrome is a form of intermittent acquired Brown’s syndrome with a clinical picture that alternates between a Brown’s-type syndrome and a superior oblique muscle palsy [983]. When the patient attempts to look up there is initially restriction but, sometimes after an audible click, the eye does eventually elevate. The click, often audible to the patient and/or the examiner, may signal the release of the restriction. The click is palpable in the superonasal orbit. Subsequently there may be initial limitation of downgaze. Lesions are located within the sheath of the anterior superior oblique tendon, and include schwannoma and giant cell tumor of the tendon.
Orbital blow-out fractures frequently incarcerate the inferior rectus muscle and its surrounding tissue. Characteristics findings include [60,264]:
• Ecchymosis of the involved eye.
• Diplopia often present in all positions of gaze immediately post trauma. This diplopia may persist in upgaze or downgaze.
• Paresthesia of the infraorbital area due to damage to the infraorbital nerve.
• Enophthalmos, either early or late.
• Entrapment of the inferior rectus, inferior oblique, and/or surrounding tissue. This results in restriction of upward gaze with positive forced duction testing. Inferior rectus paresis, resulting in hypertropia in primary position in the involved eye, may also occur due to direct nerve or muscle trauma.
• Hypotropia in primary position that increases in up gaze.
• Frequent intraocular damage.
Canine tooth syndrome is an ocular motility disorder comprising ipsilateral Brown’s syndrome and superior oblique muscle dysfunction. Ocular motility shows ipsilateral deficit of elevation and depression, maximum in an adducted position. Typically this follows a dog bite (hence the name) that damages the trochlea and superior oblique muscle concurrently. Canine tooth syndrome may also rarely occur with a closed head injury with impact to the occipital bone [785].
Thyroid (Graves’) ophthalmopathy is generally preceded by exophthalmos and orbital edema [80–82]. The myopathy of dysthyroid orbitopathy is attributed to inflammation and fibrosis of the muscles, sparing tendinous insertions. The diplopia is usually due to a “tight’ rather than “weak” muscle. The inferior recti are usually most severely affected (causing an esotropia), followed by the medial recti, superior recti, and oblique muscles (the mnemonic “I’M SO glad I do not have thyroid eye disease” is useful in remembering the frequency of muscle involvement with the “I” standing for the inferior rectus, the “M” for the medial rectus, etc.). The lateral rectus is rarely affected; therefore, the presence of an exotopia in a patient with thyroid ophthalmopathy should raise the possibility of concomitant myasthenia gravis. Vertical diplopia caused by asymmetric involvement of the inferior or superior recti muscles is the most common presentation. Diplopia in thyroid ophthalmopathy may be worse in the morning and better as the day progresses [288]. Other components of dysthyroid orbitopathy include orbital congestion, upper lid retraction, lid lag on looking down, proptosis, conjunctival injection, and optic neuropathy due to compression of the optic nerve by enlarged extraocular muscles in the orbital apex [670]. The clinical manifestations of Graves’ ophthalmopathy are outlined in Table 8.5 [547].
TABLE 8.5 Typical Features of Graves’ Ophthalmopathy
Myasthenia gravis should be considered in any case of ocular motor weakness because it can easily mimic neurogenic paresis [981]. Weakness of the extraocular muscles occurs in close to 90% of myasthenics at disease onset, and at least 15% of all myasthenics will manifest only ocular signs. Of the 50%–80% of patients with purely ocular symptoms and signs at onset that go on to develop generalized myasthenia gravis, most, but not all, develop generalized symptoms within 2 to 3 years of onset of the disorder [101]. Myasthenia gravis should be a diagnostic consideration in any patient who presents with painless, pupil-sparing, diplopia or ptosis. Any muscle may be selectively impaired, especially the medial rectus, and weakness characteristically increases with sustained effort. There is often asymmetric ptosis that becomes more pronounced on sustained upgaze. “Enhanced ptosis” may also be demonstrated (i.e., a worsening of ptosis on one side when the opposite eyelid is elevated and held in a fixed position) [356], but this sign is not specific for myasthenia because it may rarely be seen with senile ptosis, ocular myopathy, Lambert–Eaton myasthenic syndrome, Fisher syndrome, or even third nerve palsy [139,883]. During refixation from down to the primary position, the upper eyelid may bare the sclera transiently (Cogan’s lid-twitch sign). Also, a “peek sign” may occur: In an attempt to sustain forceful eye closure, the orbicularis oculi may fatigue, resulting in the patient “peeking” through the partially opened palpebral fissure. Myasthenia can mimic pupil sparing third nerve palsies, superior division third nerve palsies, abducens nerve palsies, or trochlear nerve palsies [230,623,705,866,981]. Eye movement abnormalities due to myasthenia may also mimic internuclear ophthalmoplegia [344,428], gaze palsy, one-and-a-half syndrome [224], complete external ophthalmoplegia, or other central lesions [981]. Also, certain intracranial mass lesions (e.g., parasellar tumors and aneurysms or midbrain gliomas) may mimic the weakness and fatigability of the lids and extraocular muscles seen with myasthenia gravis [14,630,637,748]. For example, isolated, intemittent unilateral ptosis was the presenting sign on a patient with a posterior carotid artery aneurysm [936]. Besides myasthenia gravis and dysthyroid orbitopathy, the differential diagnosis of chronic progressive external ophthalmoplegia (CPEO) includes oculopharyngeal dystrophy, Kearns– Sayre syndrome, myotubular myopathy, myotonic dystrophy, Bassen–Kornzweig syndrome (abetalipoproteinemia), Refsum syndrome, and Stephen syndrome (CPEO, peripheral neuropathy, and ataxia) [565].
Some of the earliest and most sensitive signs of extraocular muscle involvement with myasthenia gravis are abnormalities of saccadic eye movements and quick phases of nystagmus (see below). Large saccades may be hypometric and small saccades may be hypermetric. For large saccades, the eye may start off rapidly but slow in mid-flight and slowly reach the desired final eye position. Often a characteristic “quiver” movement occurs consisting of an initial small saccade followed by a rapid drift backward. During prolonged optokinetic nystagmus with a rotating drum, quick phases may become slow. Injection of edrophonium (Tensilon test) often reverses the extraocular muscle weakness in myasthenia gravis and may cause the saccades to become hypermetric. Sometimes with edrophonium injection the patient will be unable to hold steady fixation because of repetitive hypermetric saccades with overshoot of the target in both directions (macrosaccadic oscillations) [520,565].
Botulism, like myasthenia gravis, affects the neuromuscular junction and can cause similar eye findings, usually associated with blurred vision secondary to accommodative paresis [849]. Varying degrees of internal and external ophthallmoplegia may occur, sometimes with complete ophthalmoplegia. The myasthenic (Lambert–Eaton) syndrome may cause ptosis but seldom causes ophthalmoparesis [699].
Duchenne, Becker, and fascioscapilohumeral muscular dystrophy do not involve the extraocular muscles in most patients. Myotonic muscular dystrophy patients often demonstrate ptosis and mild defects in ocular motility including slow, hypometric saccades with increased latency and impaired smooth pursuit [565]. Oculopharyngeal muscular dystrophy is an autosomal dominant disorder in which ptosis, limitation and slowing of saccades, weakness of the facial muscles and proximal lmnb muscles, and dysphagia begin after age 40 years. The ptosis in these patients is usually more prominent than the impaired ocular motitility.
The syndrome of chronic progressive ophthalmoplegia (CPEO) is characterized by progressive limitation of eye movements and ptosis, usually without diplopia, and occurs in many disease processes. The pupils are spared but the orbicularis oculi are often involved. Saccades in CPEO are characteristically slow throughout the remaining range of movement, unlike those of myasthenia gravis in which initial saccadic velocity is often normal or increased. This impairment of saccades can often help in the differential of CPEO syndromes from myasthenia gravis.
Kearns–Sayre syndrome is a multisystem disorder characterized by progressive ophthalmoparesis starting in childhood or adolescence associated with atypical retinal pigmentary degeneration and heart block. Some patients have subjective diplopia and many have an exotorpia. Pendular nystagmus may occur. Other clinical findings include hearing loss, short stature, cerebellar ataxia, upper motor neuron signs, impaired intellect, peripheral neuropathy, “scrotal” tongue, clouding of the cornea, and endocrine abnormalities.
Autosomal recessive myopathy with external ophthalmoplegia has been described in inbred Arab families in Israel [588]. Onset is usually in childhood or the early teens with ophthalmoparesis, especially affecting upward gaze, without diplopia. Other findings include slow saccades, convergence impairment, facial and neck muscle weakness, nasal speech, mild proximal limb weakness, scoliosis, and scapular winging.
Occasionally, certain disease processes may cause isolated paresis of an individual extraocular muscle. For example, isolated inferior rectus paresis may develop with trauma, multiple sclerosis, myasthenia, or vascular disease and may also occur on a congenital or idiopathic basis [973]. Adduction impairment has been described as a manifestation of injury to medial rectus muscle after nasal septoplasty and radiofrequency ablation of the inferior turbinate [40]. Injection of Botulinu toxin (Botox) into the lateral canthal region may cause a transient lateral rectus paresis while inferior oblique paresis is an uncommon adverse effect of Botox injection into the lower lid [178]. The mechanism is postulated to be diffusion of the medication to the underlying involved muscle.
Orbital pseudotumor (idiopathic orbital inflammation) is a clinicopathologic entity with the following diagnostic criteria: (a) a unilateral orbital mass lesion, clinically presenting with signs of mass effect, inflammation, and/or infiltration; (b) neuroimaging showing a focal or diffuse inflammatory lesion; (c) histopathology demonstrating a fibro-inflammatory lesion; and (d) investigations eliminating identifiable local or systemic causes [11,533,634,635]. When the inflammatory process is confined to one or multiple extraocular muscles, the process is referred to as orbital myositis, although some authors feel that orbital pseudotumor and orbital myositis may be distinct clinicotherapeutic entities [634, 635]. Patients present with acute or subacute orbital pain and diplopia. Findings include conjunctival chemosis and injection, ptosis, and proptosis. The process may be unilateral or bilateral. The illness is often monophasic but recurrent episodes may occur.
Various inflammatory, infectious, and neoplastic processes may present clinically as orbital pseudotumor or myositis. The differential diagnosis of orbital pseudotumor is outlined in Table 8.6. Orbital pseudotumor/myositis may be difficult to differentiate from thyroid orbitopathy. The clinical differential of these two entities is outlined in Table 8.7.
Fraunfelder and Richards have reported the association between 3-hydroxy-3-methyl-glutaryl-CoA (HMG-CoA) reductase inhibitors (statins) and diplopia, blepharoptosis (ptosis), and ophthalmoplegia [306]. Two hundred fifty-six case reports of ptosis, diplopia, and ophthalmoplegia associated with statins were reported. A total of 23 case reports described total ophthalmoplegia. Ptosis was reported alone eight times and in conjunction with diplopia 18 times. There were 62 positive dechallenge and 14 positive rechallenge case reports. The plausible mechanism of the diplopia, ptosis, or ophthalmoplegia may be a myositis of the extraocular muscles, the levator palpebrae superioris muscles, or both.
Retinal Disease Causing Diplopia
Binocular diplopia may occasionally occur with retinal disease. Burgess et al. described 11 patients with subretinal neovascular membranes in one eye who developed binocular diplopia before and after effective photocoagulation therapy (the foveal displacement syndrome) [159]. The diplopia was thought to be due to rivalry between central and peripheral fusional mechanisms. The subretinal neovascular membranes produced shift of the photoreceptor array toward the proliferating neovascular complex; if the lesion was inferior to the fovea, the foveal receptors were shifted toward the membrane and with both eyes open the superior retina relative to the fovea was stimulated in the affected eye. The diplopic image produced by the stimulated superior retinal receptors is projected inferiorly in space. On covering nonaffected eye, the affected eye will have to elevate the fovea, producing a downward motion of the cornea mimicking a true hypertropia. For example, an inferior foveal lesion will mimic a hypertropia in the affected eye. All of the patients demonstrated the following:
TABLE 8.6 Differential Diagnosis of Orbital Pseudotumor
• A deviation (measured tropia) of the affected eye away from the position of the retinal lesion (e.g., a lesion inferior to the fovea produces a superior scotoma).
• The affected eye deviated upward (toward the scotoma).
• The distal diplopic image was downward (toward the retinal image).
TABLE 8.7 Clinical Differential Diagnosis of Orbital Myositis and Thyroid Eye Disease
The diplopia in this condition responds only transiently to prisms. Surgical removal of the subretinal neovascular membrane may correct the diplopia, at least transiently [142]. Foveal displacement syndrome may also occur in patients with preretinal membranes [84,94,299,848].
Ocular Motor Nerves and Localization of Lesions
Three brainstem nuclei contain the lower motor neurons that control the eye muscles: (1) the cranial nerve III (oculomotor) nucleus in the midbrain, (2) the cranial nerve IV (trochlear) nucleus at the level of the midbrain-pontine junction, and (3) the cranial nerve VI (abducens) nucleus in the lower pons. All are paired structures located in the dorsal part of the tegmentum at their respective levels. The sixth nerve innervates the lateral rectus, and the fourth nerve supplies the superior oblique. All the other muscles are innervated by the third cranial nerve. Muscles innervated by neurons on the same side (ipsilateral innervation) include the lateral (sixth nerve) and medial (third nerve) recti, the inferior rectus (third nerve), and the inferior oblique (third nerve). The superior rectus (third nerve) and the superior oblique (fourth nerve) are innervated by neurons located on the contralateral side. However, the fibers to the superior rectus cross at the level of the nucleus, so that nuclear lesions result in bilateral weakness. Similarly, oculomotor nuclear lesions cause bilateral ptosis because the nuclear group for the levator of the lid is located in the midline (a single caudal subnucleus).
OCULOMOTOR NERVE (CRANIAL NERVE III)
Anatomy. The third nerve nuclear complex extends rostrocaudally for about 5 mm near the midline in the midbrain at the level of the superior colliculus (Figs. 8.3 and 8.4). It lies ventral to the Sylvian aqueduct, separated from it by the periaqueductal gray matter, and dorsal to the two medial longitudinal fasciculi. One unpaired and four paired rostrocaudal columns can be distinguished in the oculomotor nuclear complex. The unpaired column, shared by the right and left nuclei, is in the most dorsal location and contains the visceral nuclei (Edinger–Westphal nucleus) rostrally and the subnucleus for the levator palpebrae superioris caudally. The Edinger–Westphal nucleus mediates pupillary constriction. Of the four paired subnuclei, the most medial innervates the superior rectus muscle. This is the only portion of the oculomotor nucleus that sends its axons to the opposite eye. Decussating fibers actually traverse the contralateral subnucleus for the superior rectus. Hence, a destructive lesion in one superior rectus subnucleus results in bilateral denervation of the superior recti. Laterally in each oculomotor complex there are three subnuclei: dorsal (inferior rectus), intermediate (inferior oblique), and ventral (medial rectus). Actually, neurons supplying the medial rectus are distributed into three separate areas of the oculomotor nucleus.
In the substance of the midbrain (fascicular portion), the axons of the oculomotor neurons cross the medial longitudinal fasciculus (MLF) and the decussating fibers of the superior cerebellar peduncle and then diverge widely as they traverse the red nucleus before exiting on the anterior aspect of the midbrain just medial to the cerebral peduncles. Fibers for the elevators of the eye and eyelid are probably located laterally in the fascicular portion of the oculomotor nerve. In the subarachnoid space each third nerve passes between the superior cerebellar and the posterior cerebral arteries, courses forward near the medial aspect of the uncus of the temporal lobe, pierces the dura just lateral to the posterior clinoid process, and enters the lateral wall of the cavernous sinus (Fig. 8.5; see also Fig. 7.4). Here, the nerve runs over the trochlear nerve, lying superior to the abducens nerve and medial to the ophthalmic branch of the trigeminal nerve. Once it reaches the superior orbital fissure, the oculomotor nerve divides into a superior division, that supplies the superior rectus and the levator palpebrae superioris, and an inferior division, which supplies the medial and inferior recti, the inferior oblique, and the presynaptic parasympathetic outflow to ciliary ganglion (sphincter pupillae muscle and ciliary muscles). This division into superior and inferior rami may take place also within the anterior cavernous sinus or posterior orbit, and, indeed, more proximally, even at a fascicular level.
FIG. 8.3. Sagittal section through the brainstem showing structures important in the motor control of eye movements. III = nucleus of cranial nerve III; IV = nucleus of cranial nerve IV; VI = nucleus of cranial nerve VI; PAG = periaqueductal gray; SC = superior colliculus; PC = posterior commissure; iC = interstitial nucleus of Cajal; TR = tractus retroflexus; riMLF = rostral interstitial nucleus of the medial longitudinal fasciculus; MB = mammillary bodies; CN III = cranial nerve III; CN VI = cranial nerve VI; MLF = medial longitudinal fasciculus; IO = inferior olive; MT = mammillothalamic fibers.
FIG 8.4. The oculomotor nerve. Cross-section of upper midbrain with nucleus and course and distribution of axons to the eye. (From JR Daube et al. Medical neurosciences: An approach to anatomy, pathology, and physiology by system and levels, 2nd ed. Boston: Little, Brown, 1986. By permission of Mayo Foundation.)
FIG. 8.5. Coronal diagram of the cavernous sinus. V1 = ophthalmic division of cranial nerve V; V2 = maxillary division of cranial nerve V; V3 = mandibular division of cranial nerve V.
Localization of Lesions. Lesions can affect the third nerve in the brainstem (nucleus or fascicular portion), in the subarachnoid space, in the cavernous sinus, at the superior orbital fissure, or in the orbit [138,547,549] (see Table 8.8). Etiologies of oculomotor nerve palsies, based on localization, are outlined in Table 8.9.
Pure unilateral nuclear lesions are very rare. Paresis of an isolated muscle innervated by the oculomotor nerve almost always results from lesions in the orbit or from muscle disease. However, nuclear lesions may give rise to isolated weakness of one of the muscles innervated by the oculomotor nerve (e.g., the inferior rectus) with the exception of the following muscles: superior rectus, levator palpebrae superioris, and constrictor of the pupil [95,112,191,741,895]. These muscles would be affected bilaterally even with small nuclear lesions. As medial rectus neurons probably lie at three different locations within the oculomotor nucleus, it is unlikely that a medial rectus paralysis (unilateral or bilateral) would be the sole manifestation of a nuclear lesion [943]. More characteristic of nuclear involvement is unilateral third nerve palsy, weakness of the ipsilateral and contralateral superior rectus, and bilateral incomplete ptosis [16,517,738]. Rarely, the ipsilateral superior rectus is spared while the contralateral superior rectus is paretic if the contralateral midbrain lesion selectively involves crossing superior rectus nerve fibers [228,531]. Bilateral third nerve palsies with sparing of the lid levators may also be caused by nuclear lesions (the central caudal levator subnucleus is spared) [157,496]. As corticofugal and colliculofugal (supranuclear) pathways for the control of horizontal saccades travel in the mesencephalic tegmentum near the oculomotor nucleus (see below), unilateral infarction of the midbrain– diencephalic junction may cause an ipsilateral oculomotor nuclear lesion associated with palsy of contralateral horizontal saccades [604]. Rarely, isolated bilateral ptosis with sparing of the extraocular muscles and pupils may occur with lesions involving the levator subnucleus and sparing more rostral oculomotor subnuclei [206,367,603]. After surgery for a fourth ventricle ependymoma, bilateral nuclear oculomotor palsies affecting only the levator and superior recti subnuclei have been described, resulting in third nerve paresis affecting only the levators and superior recti bilaterally [803]. Nuclear lesions affecting the pupil indicate dorsal, rostral damage and are often associated with supranuclear or nuclear vertical gaze palsies.
TABLE 8.8 The Localization of Oculomotor Nerve Lesions
TABLE 8.9 Etiologies of Third Nerve Palsies (TNP) by Topographical Localization
Although isolated inferior rectus paresis may occur due to damage of the inferior rectus subnucleus, a lesion just rostral to the third nerve nucleus selectively damaging the supranuclear descending pathway from the rostral interstitial nucleus of the medial longitudinal fasciculus (riMLF) to the inferior rectus subnucleus may also cause an isolated inferior rectus palsy [895].
Bilateral total ophthalmoplegia, bilateral complete ptosis, and large, unreactive pupils have been described with midbrain hematoma [999]. This constellation of findings was thought due to bilateral third nerve nuclear or fascicular damage or both, bilateral involvement of the interstitial nucleus of Cajal (INC) and the riMLF, and involvement of bilateral horizontal saccadic and smooth pursuit pathways (see below). A similar case was due to bilateral paramedian midbrain–thalamic infarction [925].
Fascicular lesions often accompany nuclear lesions because infarction is a common cause of nuclear third nerve palsy, and the paramedian branches near the top of the basilar artery often feed both structures. For example, infarction of the dorsal, paramedian midbrain may cause bilateral ptosis associated with unilateral paresis of all other muscles innervated by the oculomotor nerve (pupil spared) with sparing of the contralateral superior rectus muscle [580]. These unique findings suggest a lesion of the proximal third nerve fascicles and the central caudal subnucleus. Third nerve fascicular lesions are most often caused by infarction, hemorrhage, or demyelination. Pure fascicular lesions cause a peripheral type of oculomotor palsy that is associated both with ipsilateral involvement of all the muscles innervated by it and with sparing of the other eye. Furthermore, involvement of brainstem structures other than the fascicles of the third nerve helps in identifying the extent and location of the lesion. For example, ipsilateral third nerve palsy and contralateral downbeat nystagmus (see below) may be caused by unilateral paramedian thalmopeduncular infarction [696]. Fascicular involvement and concomitant damage of the red nucleus and superior cerebellar peduncle causes contralateral ataxia and outflow tract cerebellar tremor (Claude syndrome) [35,582]. A more anterior lesion, affecting the peduncle and oculomotor fascicle, gives rise to oculomotor palsy with contralateral hemiparesis (Weber syndrome). Although usually due to infarction, Weber syndrome may rarely be the presenting clinical manifestation of multiples sclerosis [596]. The third nerve palsy with Weber syndrome may affect or spare the pupillary fibers [793,794]. Larger lesions that affect the oculomotor fascicle and the red nucleus-substantia nigra region may produce oculomotor palsy with contralateral choreiform movements or tremor (Benedikt syndrome) [121], sometimes associated with contralateral hemiparesis if the cerebral peduncle is also involved [582]. Although pupillary displacement (corectopia), oval pupils, and irregularity of the pupils are occasionally found with peripheral third nerve lesions and are often due to focal pathology in the iris, they also may result from midbrain lesions (“midbrain corectopia”) [825].
Rarely, a unilateral or bilateral fascicular third nerve lesion may occur in isolation without other ocular motor or neurologic signs or symptoms [2,27,73,112,294,342,483,504,672,773,793,905,942]. Fascicular lesions, even when bilateral, may occasionally spare pupillary function [989] and bilateral preganglionic internal ophthalmoplegia (dilated nonreactive pupils) without motor involvement has been described with bilateral partial oculomotor fascicular lesions [396]. Because of the intra-axial topographic arrangement of fibers, fascicular lesions may cause third nerve palsies limited to specific oculomotor-innervated muscles [528,943]. For example, fascicular lesions have resulted in:
• Isolated inferior oblique paresis [174]
• Isolated paresis of the inferior rectus [550]
• A unilateral fixed, dilated pupil unassociated with other neurologic dysfunction [844]
• Paresis of the superior rectus and inferior oblique (monocular elevation paresis) without other evidence of oculomotor nerve involvement [192,333]
• Paresis of the superior rectus and medial rectus [794]
• Paresis of the levator muscle, superior rectus, and medial rectus [700]
• Paresis of the inferior oblique, superior rectus, medial rectus, and levator muscle with sparing of the inferior rectus muscle and pupil [668,822,843]
• Paresis of the inferior oblique, superior rectus, medial rectus, levator, and inferior rectus with pupillary sparing [145,416,668]
• Paresis of the left inferior rectus, left pupil, right superior rectus, convergence, and left medial rectus [943].
Based on these clinical studies, it has been proposed that individual third nerve fascicles in the ventral mesencephalon are arranged topographically from lateral to medial as follows: inferior oblique, superior rectus, medial rectus and levator palpebrae, inferior rectus, and pupillary fibers (Fig. 8.6) [174]. A rostral-caudal topographic arrangement has also been suggested with pupillary fibers most superior, followed by fibers to the inferior rectus, inferior oblique, medial rectus, superior rectus, and levator, in that order [794,822,943]. This model also serves the description of a “superior division” oculomotor palsies (i.e., paresis of the superior rectus and levator muscles without involvement of other groups) [368,420,527] and an “inferior division” oculomotor palsies (paresis of inferior rectus, inferior oblique, medial rectus, and pupillary fibers with sparing of the superior rectus and levator) [2,270,527] associated with intraaxial midbrain lesions. Thus, although superior and inferior divisional third nerve palsies have classically been localized to anterior cavernous sinus or posterior orbital lesions, such reports suggest that a divisional third nerve pattern may occur from damage at any location along the course of the oculomotor nerve, from the fascicle to the orbit [527].
FIG. 8.6. Schematic diagram of midbrain at level of superior colliculus. Proposed model of oculomotor fascicular organization in ventral midbrain tegmentum from lateral to medial is as follows: inferior oblique (IO) fascicles, superior rectus (SR) fascicles, medial rectus (MR) fascicles, levator palpebrae (lid) fascicles, inferior rectus (IR) fascicles, and, most medially, pupillary fibers (pupil). (From O Castro et al. Isolated inferior oblique paresis from brainstem infarction. Perspective on oculomotor fascicular organization in the ventral midbrain tegmentum. Arch Neurol 1990;47:235–237. Copyright 1990, American Medical Association. Reprinted with permission).
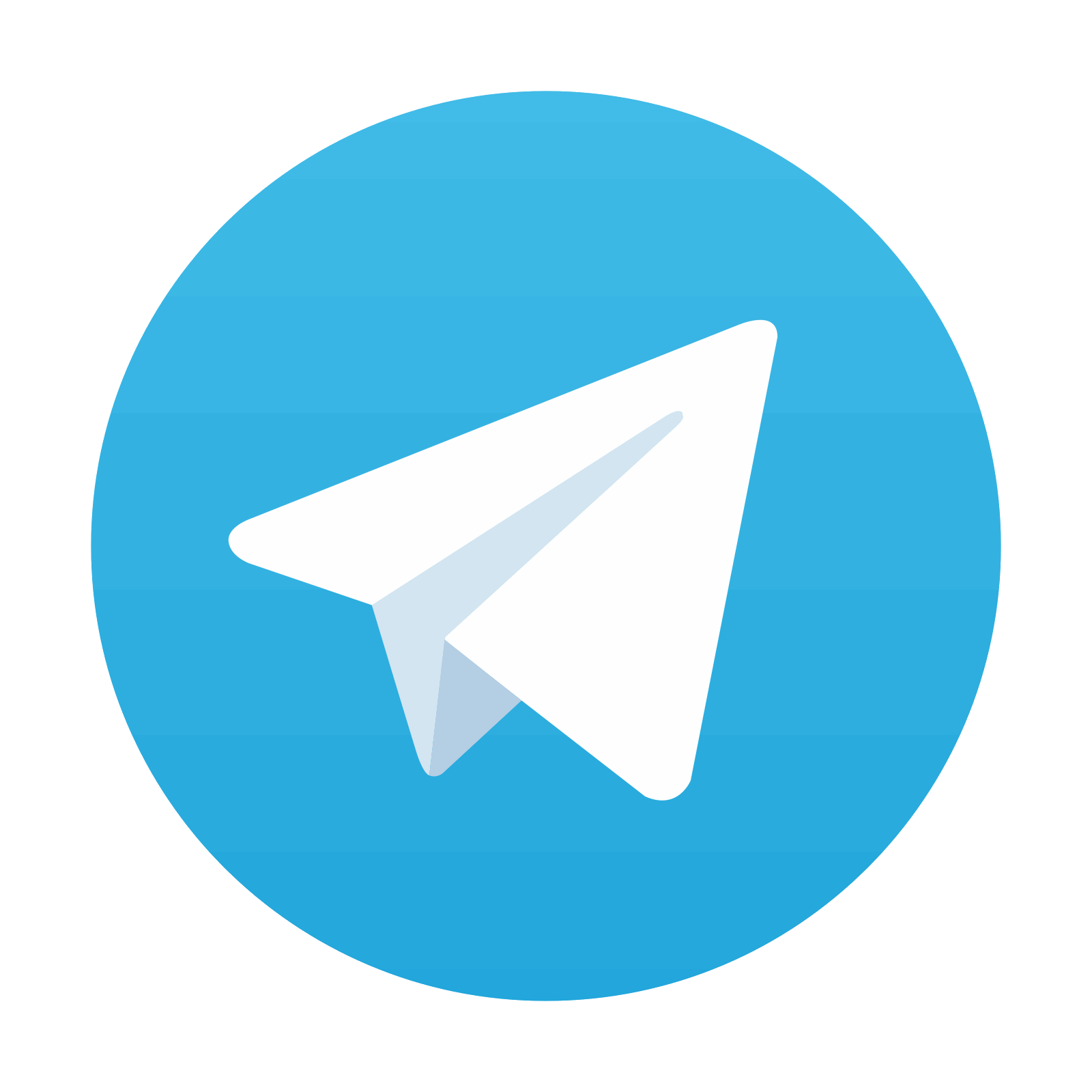