Chapter 5 Acute Sleep Deprivation
Abstract
This chapter will review behavioral, physiologic, and theoretical implications of acute sleep deprivation. Chronic partial sleep deprivation is examined in Chapter 6. Studies of sleep deprivation can suffer from some common methodological problems that require consideration. The most important control issue is that one cannot perform a blinded sleep deprivation study. Both experimenter and subject motivation can have an impact on results, particularly in the behavioral and subjective domains. Motivation effects are frequently apparent near the end of sleep deprivation studies (where performance improvement is sometimes found) and may account for the difficulty in showing early decrements. Animal studies are less susceptible to subject expectation effects, but they may contain additional elements of stress that may interact with sleep loss, so these studies may not be directly comparable with stress control conditions. In addition, almost all sleep loss experiments involve more than simple loss of sleep. Maintenance of wakefulness usually includes upright posture, light, movement, cognition, and all the underlying physiologic processes implied by these activities. The experimental setting itself is usually far from routine. Some studies have attempted to control some of these factors during sleep loss, but trying to control all variables in a single experiment is daunting.
Total Sleep Deprivation
The first published studies of total sleep loss date to 1894 for puppies1 and 1896 for humans.2 The puppy study indicated that prolonged sleep loss in animals could be fatal, an idea reinforced by numerous, more recent animal studies. The human study included a range of physiologic and behavioral measurements and remains a model study.
Behavioral Effects
The most striking effect of sleep loss is sleepiness, and this can be inferred from subjective reports, the multiple sleep latency test (MSLT), electroencephalographic (EEG) change, or simply looking at the face of the participant. The variables that determine the impact of sleep loss can be divided into four categories: sleep/circadian influences, arousal system influences, individual characteristics, and test characteristics (Box 5-1).
Sleep/Circadian Influences
Sleep deprivation, like nutritional status, is a relative concept. How an individual responds to sleep loss depends on the prior sleep amount and distribution. Performance during a period of sleep loss is also directly dependent on the length of time awake and the circadian time, and predictive models support these factors. Experiments usually try to control prior wakefulness and sleep amount by requiring “normal” nights of sleep before initiation of a sleep loss episode. Data from multiple regression analyses of behavioral and EEG data during 64 hours of sleep loss3 suggest that time awake accounts for 25% to 30% of the variance in alertness, and that circadian time accounts for about 6% of the variance. When prophylactic naps of varying length were interjected early in a period of sleep loss, it was found that the prophylactic nap sleep accounted for about 5% of the variance in alertness during the sleep deprivation period. In terms of reducing the effect of sleep loss, the overall effect of increasing the prophylactic nap period was linear for additional sleep amounts ranging up to 8 hours in length. Figure 5-1 displays the effects of time awake and the circadian rhythm on objective alertness as measured by the MSLT and the ability to complete correct symbol substitutions during 64 hours of sleep loss.
Arousal Influences
Activity
A 5-minute walk immediately preceding MSLT evaluations had a large impact (about 6 minutes) on MSLT values, which masked the impact of a 50% reduction of nocturnal sleep (about a 2-minute reduction on MSLT) and continued for at least 90 minutes.4 Exercise before performing tasks provided transient reversal of some psychomotor decrements resulting from sleep loss. However, more ambitious studies comparing high activity and low activity that continued over 40 to 64 hours of sleep deprivation have shown no beneficial effects of exercise on overall performance.5 Perhaps, arousing stimuli act for only a discrete period of time that is decreased by increasing sleep loss.6 There may also be a trade-off between production of arousal and production of physical fatigue.
Bright light
Bright light can shift circadian rhythms. Some controversy exists concerning whether bright light can also act as a source of stimulation during a sleep-loss state to help to maintain alertness. Two of five studies found that periods of bright light immediately before sleep onset significantly increased sleep latencies. Other studies found improved nocturnal performance during bright light conditions, with elevated heart rate as a probable correlate.7
Noise
Noise, despite complex and occasionally negative effects on the performance of well-rested individuals, may produce small beneficial effects during sleep deprivation.8 It is generally assumed that noise increases arousal level, and this may provide maximal benefit during sleep loss.
Temperature
Although temperature variation is commonly used as an acute stimulus to maintain alertness, little research supports ambient temperature as a large modulator of alertness. One study has shown that heat (92° F) was effective in improving performance during the initial minutes of a vigilance task during sleep deprivation.9 However, another study10 showed only a small decrease in subjectively rated sleepiness for about 15 minutes after a car air-conditioner was turned on during simulated driving.
Posture
One study has shown a significant increase in sleep latency, as a measure of alertness, when subjects were asked to fall asleep in the sitting position (60-degree angle) as opposed to lying down.11 Such a difference could be accounted for by increasing sympathetic nervous system activity, which occurs as one moves to the upright posture.
Drugs
Many drugs have been studied in conjunction with sleep loss, and an extensive review by the American Academy of Sleep Medicine has been published.12 Most studies have examined stimulants, including amphetamine, caffeine, methylphenidate, modafinil, armodafinil, nicotine, and cocaine.
Numerous studies have shown that caffeine (dosages of 200 to 600 mg), modafinil (100 to 400 mg), and amphetamine (5 to 20 mg) can improve objective alertness and psychomotor performance for periods of time related to dosage, half-life, and hours of total sleep deprivation. However, head-to-head studies often provide the clearest comparison of compounds. One study13 examined alertness and response speed hourly for 12 hours during the first night of sleep deprivation after administration of modafinil (100, 200, and 400 mg) in comparison with caffeine 600 mg. In that study, modafinil dosages of 200 and 400 mg were shown to be equivalent to caffeine (600 mg) in maintaining response speed consistently above placebo levels for 12 hours. The same group compared modafinil (400 mg), caffeine (600 mg), and dextroamphetamine (20 mg) given just before midnight of the second night of total sleep loss.14 In this study, caffeine and dextroamphetamine significantly improved response speed only at midnight, 2:00 AM, and 4:00 AM, whereas modafinil improved response speed through 10 AM. The decreased sensitivity to caffeine probably reflects both the half-life of caffeine and the increased sleep pressure from the second night of deprivation. Side effects were fewest after modafinil at 400 mg. However, the authors concluded that (1) these stimulants all provided some benefits and had some associated costs, (2) caffeine, with efficacy, availability, and low cost, can be a first choice for alertness, and (3) modafinil, with good efficacy and few side effects, would be a good substitute if caffeine were ineffective (related to time course of available administration, tolerance, side effects, or degree of sleep loss).
Effects of stimulants can be enhanced by the use of naps or other sources of arousal. The beneficial effect of caffeine (300 mg) during periods of sleep loss was approximately equivalent to that seen after a 3- to 4-hour prophylactic nap before the sleep loss period.15 The combination of a 4-hour prophylactic nap followed by 200 mg of caffeine at 1:30 AM and 7:30 AM resulted in significantly improved performance (remaining at baseline levels) compared with the nap alone, for 24 hours.16 The combination of naps and caffeine was additive16 and superior to the provision of 4 hours of nocturnal naps. The combination of 200 mg of caffeine administered at 10:00 PM and 2:00 AM and exposure to 2500-lux bright light had no impact beyond the effect of caffeine alone on the maintenance of wakefulness test but did provide significant benefit above caffeine alone on a vigilance task.17
Recent work has shown that armodafinil (the levorotatory R-enantiomer of modafinil), which has a 10- to 14-hour half-life, is effective in maintaining alertness and performance throughout 1 night of sleep deprivation.18 Results with armodafinil (200 mg) appeared to be similar to those with modafinil (200 mg) but may have provided some additional benefit 11 to 13 hours after administration.18
Nicotine, infused intravenously at dosages of 0.25, 0.37 and 0.5 mg after 48 hours of wakefulness, had no significant impact on MSLT or psychomotor performance.19 Cocaine (96 mg), like amphetamine, did not improve performance in subjects before sleep loss. However, reaction time and alertness, as measured by the Profile of Mood States, was improved after 24 and 48 hours of sleep loss.20
Alcohol use has been found to consistently reduce alertness.21 Subjects were tested with the MSLT and simulated driving after 0.6 g/kg alcohol or placebo following normal sleep or 4 hours of sleep. There was a significant main effect for condition, and MSLT latencies were 10.7, 6.3, 6.1, and 4.7 minutes after placebo (normal sleep), placebo (4 hours sleep), ethanol (normal sleep), and ethanol (4 hours sleep), respectively. Similar results were found in the driving simulator, where there were three “crashes”—all in the reduced-sleep-with-ethanol condition. One difficulty in assessing the magnitude of performance effects associated with sleep loss is the lack of a clear standard of pathology for most measures. The fact that society has established very specific rules for blood alcohol content with respect to driving has led to the use of impairment associated with blood alcohol level as a standard reference for sleep deprivation as well. Several studies of alcohol use in direct comparison with sleep deprivation have shown decrements on different tasks. Response speed on the Mackworth task was reduced by approximately half a second by 3:45 AM (i.e., with sleep loss) and to a similar extent by a blood alcohol content (BAC) of 0.1%. Also, hand–eye coordination (in a visual tracking task) declined in a linear fashion during sleep loss and with increasing BAC, such that performance was equivalent at 3:00 AM to a blood alcohol level of 0.05% and equivalent at 8:00 AM (after a full night of sleep loss) to a blood alcohol level of 0.1%. In a third study,22 performance was measured in a driving simulator after alcohol use or sleep deprivation. After a night of sleep deprivation (at 7:30 AM), subjects averaged one off-road (i.e., vehicle driving off the road) incident every 5 minutes. This same level of off-road driving was reached with a BAC of 0.08%. These studies suggest that the changes in response speed, visual tracking, and driving commonly found during the first night of total sleep deprivation are equivalent to changes associated with legal intoxication. Such metrics provide useful understanding of the consequences associated with short periods of sleep loss.
Motivation or Interest
Motivation is relatively easy to vary by paying subjects and has therefore received attention. In one study, monetary rewards for “hits” on a vigilance task and “fines” for false alarms23 resulted in performance being maintained at baseline levels for the first 36 hours of sleep loss in the high-incentive group. Performance began to decline during the following 24 hours but remained significantly better than in the “no incentive” group. However, the incentive was ineffective in maintaining performance at a higher level during the third day of sleep loss. Knowledge of results—for example, the publication of daily test results—was sufficient to remove the effects of 1 night of sleep loss. In another variation, simple knowledge that a prolonged episode of sleep deprivation was going to end in a few hours was sufficient for performance to improve by 30% in a group of soldiers.24
Group Effects
There are few studies, but interest is growing in how groups perform during sleep deprivation. Such studies are difficult because groups of individuals interact in many ways. One early study suggested that, if all group members were working at a similar task, greater deficits were seen as sleep deprivation progressed, compared with individual work. However, a more recent study that distributed work so that each individual added a unique component to task completion found that the deficits that accumulated during sleep deprivation were less pronounced in the group task.25
Repeated Periods of Sleep Loss
Studies of repeated episodes of sleep loss have agreed that the magnitude of performance loss increases as a function of the number of exposures to sleep loss.26 Increasingly poor performance may be secondary to decreased motivation or to familiarity with the sleep deprivation paradigm (resulting in decreased arousal).
Individual Characteristics
Age
Tests of performance and alertness in older subjects undergoing sleep loss reveal a decrease in performance and alertness similar to that seen in younger individuals. If anything, older men had a smaller decrease in psychomotor performance ability at nocturnal times during sleep loss27,28 than younger men. Older individuals perform more poorly than young adults on a broad range of tasks, but, because of decreased amplitude of the circadian body temperature rhythm, this relationship may not be maintained across the night or during sleep loss.27 The same flattened curve associated with lower temperatures and decreased performance during the day also produces relatively elevated temperatures that could be related to improved performance at night.
Sensitivity to Sleep Loss
A number of studies have now demonstrated consistent individual differences in both alertness and performance during sleep deprivation.29 The studies have also shown that the reliable changes in subjective alertness, objective alertness, and performance are not related to one another. Performance consistently declined in the same subjects when sleep loss was repeated, but decrements did not generalize across performance tasks. This has led some to speculate that different brain areas could be responsible for different tasks. Some studies have begun to look for central predictors of performance loss. Early functional magnetic resonance imaging (fMRI) studies showed that subjects with higher levels of global brain activation (consistent at both baseline and during sleep loss) maintained better performance on a working memory task,30 and a more recent study linked working memory during sleep loss with left frontal and parietal brain areas.31 Other studies have shown that extroverts and caffeine-sensitive individuals were more sensitive to sleep loss.32 Such findings imply individual trait ability to maintain higher levels of arousal in specific brain areas or systems to help to maintain performance during sleep loss.
In another approach, over 400 potential subjects were screened genetically, and groups were formed on the basis of the PER3 polymorphism (PER3[5/5] versus PER3[4/4]) before a 40-hour constant routine. The PER3(5/5) group appeared sleepier by all measures (significantly shorter sleep latency, more slow-wave sleep (SWS), greater slow eye movements during sleep loss, and significantly worse performance during sleep loss, particularly on executive tasks done early in the morning [0600 to 0800]).33,34
Personality and Psychopathology
Mood changes, including increased sleepiness, fatigue, irritability, difficulty in concentrating, and disorientation, are commonly reported during periods of sleep loss. Perceptual distortions and hallucinations, primarily of a visual nature, occur in up to 80% of normal individuals, depending on work load, visual demands, and length of deprivation.35 Such misperceptions are normally quite easy to differentiate from the primarily auditory hallucinations of a schizophrenic patient, but normal individuals undergoing sleep loss may express paranoid thoughts. Two percent of 350 individuals sleep deprived for 112 hours experienced temporary states resembling acute paranoid schizophrenia. Some predisposition toward psychotic behavior existed in individuals who experienced significant paranoia during sleep loss, and the paranoid behavior tended to become more pronounced during the night, with partial recovery during the day and disappearance after recovery sleep. In a review of the area, Johnson concluded, “Each subject’s response to sleep loss will depend on his age, physical condition, the stability of his mental health, expectations of those around him, and the support he receives.”36, p. 208 At a more general level, normal adults undergoing sleep deprivation typically express some increase in somatic complaints, anxiety, depression, and paranoia that do not reach clinical levels.37
In view of the commonly reported effects of sleep deprivation, it seems quite unusual that one would seek to treat depression by sleep deprivation. However, sleep deprivation has been used as a successful treatment for depression in 40% to 60% of cases for over 30 years. Imaging studies have shown that depressed patients have elevated metabolism in the prefrontal cortex and ventral anterior cingulate cortex (possibly related to reduced transmission of dopamine and serotonin) that is normalized by sleep deprivation.38 One theory proposes that sleep deprivation is more effective in depressed patients with high levels of activation or high central noradrenergic activity because it limits the effects of chronic hyperarousal. As a result, patients felt tired but also had improved mood and energy.
Test Characteristics and Types
Two meta-analyses of subtopics of sleep deprivation have been published.39 Both analyses indicated that sleep deprivation has a significant impact on psychomotor performance. In general, longer periods of sleep loss had greater impact on performance, and decrements in speed of performance were greater than decreases in accuracy. Also, mood measures were more sensitive than cognitive tasks, which were more sensitive than motor tasks,39 during sleep loss. Therefore, the measured response to sleep deprivation is critically dependent on the characteristics of the test used. To some extent, the type of test has also been used to infer specific brain area dysfunction. Sleep-deprived individuals appear most sensitive to the following dimensions.
Length of Test
Individuals undergoing sleep loss can usually rally momentarily to perform at their non–sleep-deprived levels, but their ability to maintain that performance decreases as the length of the task increases. For example, subjects attempted significantly fewer addition problems than baseline after 10 minutes of testing following 1 night of sleep loss but reached the same criterion after 6 minutes of testing following the second night of sleep loss. It took 50 minutes of testing to show a significant decrease in percentage of correct problems after 1 night of sleep loss, and 10 minutes of testing to reach that criterion after the second night.40 It is frequently difficult to show reliable differences during short-term sleep loss from almost any test that is shorter than 10 minutes in duration. Momentary arousal, even as minor as an indication that 5 minutes remained on a task, was sufficient to reverse 75% of the decrement accumulated over 30 minutes of testing.
Knowledge of Results
Immediate performance feedback, possibly acting through motivation, has been shown to improve performance during sleep deprivation.41 Simply not giving knowledge of results to subjects with normal sleep doubled their number of very long responses (“gaps”) on a serial-reaction time test. One night of total sleep loss increased the number of gaps by 9.3 times the baseline level, but provision of immediate knowledge of results decreased the number of gaps back to baseline levels.41
Proficiency Level
Sleep loss is likely to affect newly learned skills more than well-known activities, as long as arousal level remains constant. For example, in a study of the effects of sleep loss on doctors in training, significant performance decrements were found in postgraduate year (PGY)-1 surgical residents but not in PGY-2 to -5 surgical residents.42
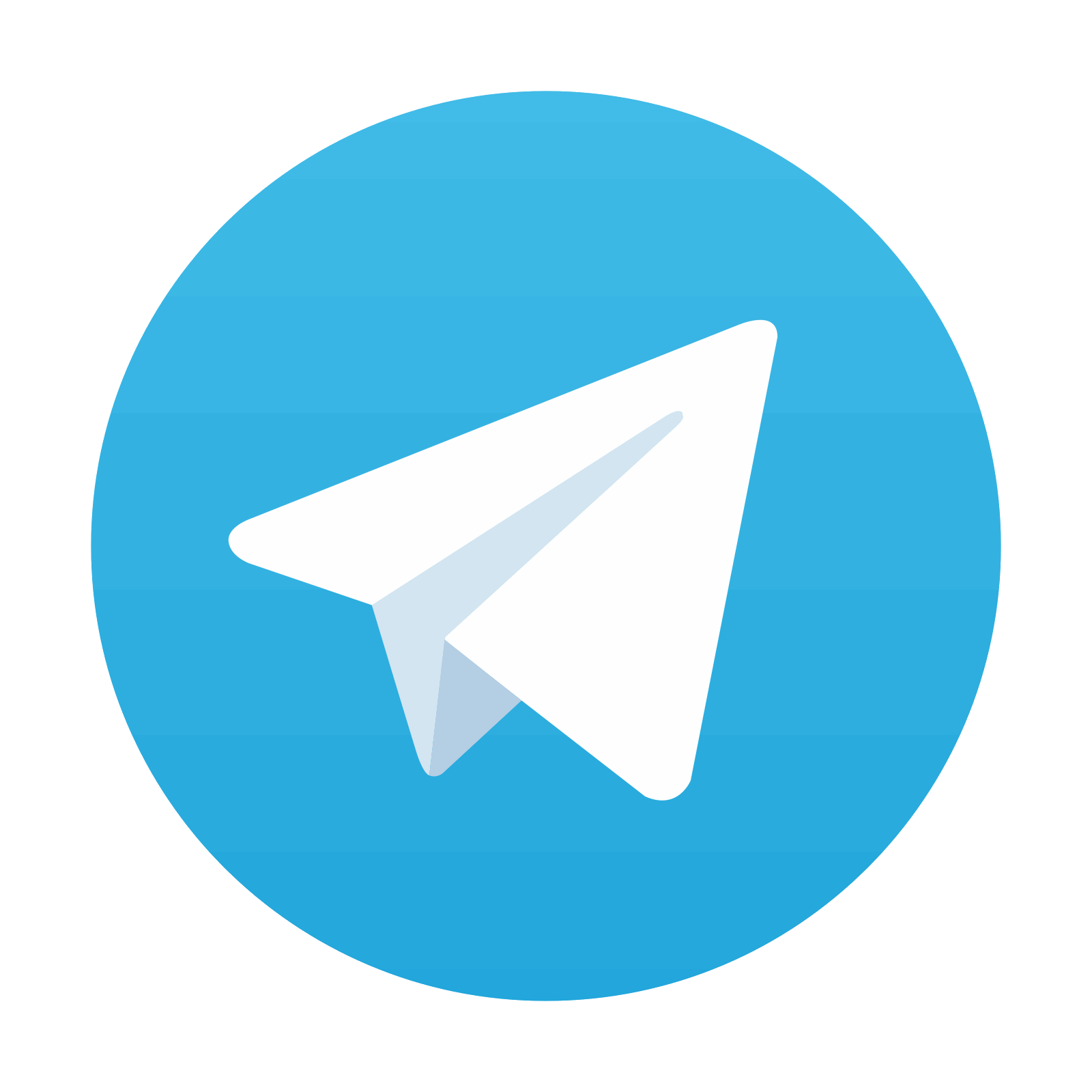
Stay updated, free articles. Join our Telegram channel

Full access? Get Clinical Tree
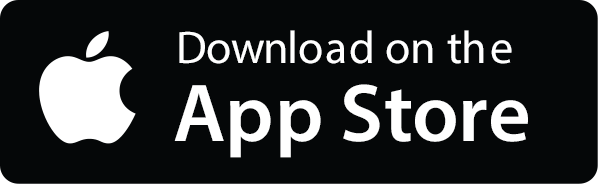
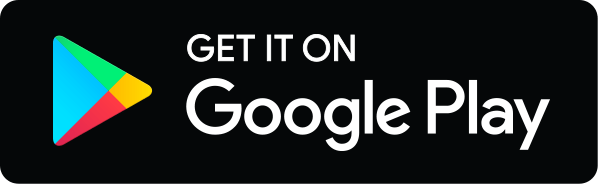