Introduction
At the turn of the twentieth century, Dr. Alois Alzheimer described a form of progressive presenile dementia in a female patient named Auguste Deter, who developed memory loss and died at the age of 56 [1]. Postmortem neuropathology revealed extensive brain shrinkage and accumulation of senile plaques (SPs) and neurofibrillary tangles (NFTs) [2], now considered the defining lesions of the disease bearing his name. SPs accumulate in the extracellular matrix and contain insoluble fibrils of amyloid beta (Aβ) protein fragments, which are derived from the larger transmembrane amyloid precursor protein (APP) through serial cleavage by beta-site APP cleaving enzyme 1 (BACE1) and the γ-secretase complex [3–5]. NFTs are composed of argyrophilic aggregates of several hyperphosphorylated epitopes of the protein tau [6, 7]. These pathological protein aggregates display a β-pleated sheet conformation and are thought to interfere with cytoskeletal integrity, which disrupts synaptic and neuronal function. In over 99% of individuals, the onset of Alzheimer’s disease (AD) occurs in late adulthood, usually after the age of 65 [8]. In a small portion of people (< 1%), the disease displays an autosomal dominant pattern of inheritance (“familial AD,” FAD), resulting from one of three different gene mutations, APP, presenilin 1 (PS1), or presenilin 2 (PS2) and manifests much earlier [8]. Interestingly, genetic analysis of tissue from the brain of Auguste Deter revealed a PSEN1 mutation [1, 9], which was consistent with her early age of disease onset. Genotyping for APOE ɛ4, a major risk factor for AD, revealed that Augusta Deter was an ɛ3/ɛ3 carrier [1].
Although late onset sporadic AD is the leading cause of dementia in the United States, affecting an estimated 5.4 million people and is predicted to afflict 13 million people in the USA by 2050 [8], the field lacks a true animal model that fully recapitulates the pathobiology underlying the disease. Currently, premortem clinically and postmortem neuropathologically well-characterized human brain tissue provides the gold standard for defining the pathophysiology of AD. Although similar to AD, individuals with Down syndrome (DS) develop SP and NFT pathology and, dementia as they age, there is a discrepancy between the prevalence of AD pathology (about 100% by age 40) and the prevalence of dementia (about 2%–5% by age 40 and 70% by age 70) in adults with DS [10–12] (see Chapters 1, 13, and 15). This population provides a unique resource for the investigation of the location, temporal course, and clinical association of the cellular and molecular neuropathology related to the development of AD.
As described in Chapter 1, in 1866, the Cornish physician John L. Down published an article entitled “Mental affections of childhood and youth,” which described the external and innate characteristics of people referred to as a “mongoloid idiot.” Down’s grandson was born with this condition and displayed a phenotype (described by Dr. Down) that included a round face, oblique eyes, flat nape, thin eyebrows, a small pug nose, and thick cleaved tongue [13]. In 1965 the World Health Organization officially confirmed the eponym for this disorder as Down syndrome (DS). Although Down assumed that parental tuberculosis was the cause of this disorder [14], genetic analysis revealed that DS was due to an extra copy of chromosome 21 (Ch21) [15]. This chromosome harbors the gene encoding APP, that results in an overproduction of the Aβ peptide and is associated with the onset of FAD (< 50 years of age) [16–18]. Several groups linked the gene for the APP to a locus on the proximal portion of the long arm of Ch21 [19–21], which then narrowed the location of APP to Ch21 [19, 20]. Behaviorally, DS is characterized by intellectual disability attributed to a full or partial extra copy of human Ch21 (HSA21) [15] that accounts for 95% of the chromosomal anomalies in this disorder, or lesser frequent genotypes associated with a translocation of Ch21 onto another chromosome (4%) and mosaicism where some cells exhibit an extra copy of Ch21 (1%). DS occurs in 1 in 800 births in the United States and affects approximately 6 million people worldwide. The extra copy of Ch21 results in developmental alterations that distinguishes DS from the neurotypical brain.
Prenatal brain pathology in DS
During gestation, the DS brain appears smaller than its neurotypical counterpart (Fig. 1). In the neurotypical cortex the lateral sulcus (Sylvian fissure), which first appears on the superolateral surface as a minor indentation, is observed as early as gestational weeks 13.5 in DS (Fig. 1E). At these early developmental ages, the corpus callosum and callosal sulcus appear on the medial surface of the hemisphere, although these structures are more evident in the neurotypical brain (Fig. 1A′ and E′). During normal brain embryology, the lateral sulcus gradually becomes deeper, at the same time as the frontal, parietal, and temporal opercula are rapidly expanding (Fig. 1C and D) compared to a less well-developed narrow lateral fissure seen in DS gestational weeks 20 and 26 (Fig. 1F and G). At about week 21 of gestation, the central sulcus appears as a small groove in the dorsal region of the normal cortex (Fig. 1C), which was not evident in DS (Fig. 1F). However, the central sulcus was clearly visible in the dorsolateral cortex at gestational week 26 in DS (Fig. 1G). At 24 weeks’ gestation, the neurotypical cortex displays a pre- and postcentral sulci as well as a developing superior temporal sulcus (Fig. 1D) that are not clearly visible at embryonic week 26 in DS (Fig. 1G). At fetal week 17 the medial surface of the neurotypical brain displayed cortical invagination indicative of the callosal fissure and the developing corpus callosum (Fig. 1A′), which was less well defined at gestational week 13.5 in DS (Fig. 1F′). The medial surface of the neurotypical cortex also revealed indentations indicative of a developing calcarine and parietooccipital sulcus (Fig. 1B) similar to that seen at 26 weeks in the DS brain (Fig. 1G′) [22]. We were able to identify the cerebellum at DS fetal week 13.5 (Fig. 1E, E’) and 26 (Fig. 1G, G’). The developmentally altered trisomy brain indicated by delayed central nervous system maturation associated with prenatal arrest of neuro and synaptogenesis [23, 24] may be partially a consequence of delayed cortical development. It is important to note these developmental differences in the brains of people with DS, since these regions are similar to those later affected by aging and AD.

Adult brain pathology in DS
Gross evaluation of the adult DS brain revealed abnormalities that include a reduction in brain weight, altered configuration, less numbers of gyri and depth of cerebral sulci, stumpy/shortened appearance of the frontal and temporal lobes, hypoplasia of the brainstem and cerebellum (Fig. 2). Coronal sections of the DS brain revealed ventricular enlargement (Fig. 2), cortical and hippocampal shrinkage (Fig. 2) [25–28], as well as alterations of cortical lamination. Increased cortical thickness has been reported in sensory (Brodmann areas in 1, 3b), middle frontal, and orbital cortex [29] in DS. Although these changes may play a role in defects in somatic, memory, and olfactory responses in people with DS, there is variability in these alterations across cortical regions. In 1948 George Jervis reported that individuals with DS displayed dementia premortem and a postmortem neuropathological phenotype that included SP and NFT similar to that described 60 years earlier by Dr. Alzheimer [2, 30]. Other studies confirmed these pathological findings and showed a similar topography of these lesions between DS and euploid AD cases [31]. Individuals with DS also exhibit characteristics of premature aging and are at a higher risk for developing dementia of the AD type several decades earlier than patients with sporadic AD [10, 32–34] (see Chapters 1, 13, and 15). Despite parallels between AD and DS, current transgenic mouse models do not truly replicate these disorders and the consequence of trisomy in nonhuman species is not consistent with the genotype or phenotype of DS [35]. Therefore this chapter will provide an overview of the similarities and differences in the cellular and molecular pathobiology of the human DS brain from gestation to older ages in contrast to AD.

Cortical amyloid in AD and DS
Although all cases of DS display AD pathology, only about two-thirds develop dementia [10] (see Chapters 1, 13, and 15). The biology underlying this disconnect between the presence of AD neuropathology and presence of dementia is an underinvestigated area, primarily due to the lack of autopsy cases that are clinically well characterized. In AD and DS, an increase in soluble Aβ species precedes plaque deposition [34, 36, 37], and occurs as early as 21 gestational weeks in DS [36, 37]. In a recent study, amyloid plaques were not seen at 0.01, 1.6, and 3 months of age in the frontal and temporal cortex or brainstem in DS [38]. In our ongoing developmental investigations, we were not able to immunohistochemically visualize Aβ40, Aβ42, or Aβ plaques-like structures in the frontal cortex at 6 months, 1 year (Fig. 1H–K), and in a 3-year-old DS case. However, an occasional diffuse starburst deposit displaying immunoreactivity against the 6E10 antibody, which recognizes amyloid precursor protein (APP) and Aβ was found in these same cases (Fig. 1H). Interestingly, there is one other case report showing a similar “starburst” pattern [39]. By contrast, no amyloid-like staining was seen in a 5-year-old neurotypical case. These observations suggest that APP and not Aβ is an early event in the developing DS cortex, which may be related to altered axonal pruning and neuronal culling [40]. Although diffuse deposits of Aβ42 have been reported in people with DS between 8 and 27 years of age [38, 41, 42], an earlier time point than seen in AD [41–43], they appear to have negligible effects upon neurons and their deposition is not associated with clinical symptoms [44]. In DS cases aged 40–50 years, levels of cortical Aβ deposition are similar to those observed in sporadic, late onset AD [11, 42, 45–49]. In addition to diffuse plaques, a few cored plaques associated with dystrophic neurites (neuritic plaques) have been reported at age 19 and in a 30-year-old DS case [38], which are of neuropathological diagnostic significance in AD. By age 40, people with DS exhibit Aβ plaque density and morphology similar to that seen in AD [38].
Cerebellar amyloid in AD and DS
Although neuropathological reports suggest that the reduced size of the DS cerebellum leads to a delay in fine motor development [25, 50], studies of AD neuropathology in this structure during gestation are minimal. We found scattered diffuse amyloid-like plaques, some containing a dense central core immunoreactive for APP/Aβ1–16 (6E10) in the white matter and granular cell layer of the cerebellum at postnatal day 10 and between 4 and 20 weeks of age in DS. Another study reported virtually no amyloid plaque pathology between 0 and 53 years of age using the 4G8 antibody (APP/Aβ17–24), but beyond this age all cases displayed various levels of Aβ pathology in DS [38]. The difference between earlier and a more current study [38] may be related to the sequence specificity of the 4G8 antibody [51]. However, in both the adult AD and DS cerebellum, amyloid deposition visualized using an antibody against the Aβ peptide (Aβ4) appears as amorphous patches, which often occur perpendicular or parallel to the pial surface within the molecular layer (ML) [52]. We recently investigated the deposition of amyloid using antibodies that detect different epitopes of the Aβ sequence in cerebellar cortex obtained from demented (average age 51.2; range 45–59) and nondemented (average age 50.3; range 44–60) individuals with DS (average age 81.7; range 71–98) and healthy aged controls (average age 70.9; 51–85). Cerebellar tissue reacted with an antibody that recognizes both APP and Aβ (6E10), revealed patches of APP/Aβ in the ML that was greater in both DS groups compared to AD and nondemented healthy subjects (Fig. 3A–D). Since the elevation of levels of the long form of Aβ, Aβ42, compared to the short form, Aβ40, plays a role in the early events underlying the pathogenesis of AD, we evaluated Aβ42 and Aβ40 immunoreactivity within cerebellar tissue from these same adult cases. Aβ42, but not Aβ40 immunoreactivity, was found in the cerebellum of both DS groups but to a lesser extent in AD (Fig. 3E–H). In DS, Aβ42 appeared as bands perpendicular to the pial surface within the ML (Fig. 3F and G). Interestingly, only scattered patches of APP/Aβ and Aβ42 were seen in the cerebellar ML in AD (Fig. 3D and H). Both demented and nondemented individuals with DS had significantly higher Aβ42 plaque loads in the ML compared to nondemented controls. Cerebellar Aβ42 loads in demented individuals with DS were significantly increased compared to AD [53].

Striatal amyloid pathology in AD and DS
Although striatal plaques mainly exhibit a diffuse morphology, there are reports of cored/neuritic plaques in this region in AD [54, 55]. Neuropathological studies revealed that Aβ42 positive plaques first develop in the striatum in DS and in select FAD types of PS1 mutation carriers, as early as the second decade of life [42, 56]. Since APP cleavage products appear more resistant to degradation, it is possible that they act as early seeds for the eventual formation of amyloid plaques in the adult DS brain. Postmortem reports indicate that amyloid in the striatum appears 20–30 years later in life compared to the neocortex in DS [45, 46]. Data derived from various studies of individuals with DS ranging in age from newborn to 76 years [45, 46] revealed an absence of striatal amyloid in all cases aged 50 years or less. Studies suggest that only 7% of cases over 55 years had a greater striatal amyloid load than found in the temporal cortex, while 36% had a higher amyloid load in temporal cortex than the striatum and 29% had similar amyloid loads in both regions [38]. These finding suggest that amyloid pathology displays a regional development in people with DS similar to the Thal and Braak phases seen in AD [57, 58], with neocortical regions including temporal neocortex affected earlier than the striatum and other subcortical regions. By contrast, amyloid positron emission tomography (PET) Pittsburgh Compound B (PiB) imaging studies reported that striatal amyloid deposition precedes neocortical amyloid [59–62], which was related to cognitive decline in DS [59] (see Chapter 9). When prevalence of amyloid positivity was assessed using PiB, both the striatum and precuneus cortex showed the highest values in the youngest participants (age range 36–40 years), suggesting that these brain regions are affected early by amyloid in DS [63]. It has been suggested that striatal amyloid labeling may be a measure for pathology-based clinical staging of AD, as increased sensitivity of [F-18]Flutemetamol PET was associated with higher histological density thresholds of striatal amyloid deposits [64]. It remains to be determined whether PET ligand imaging would reveal differences in brain amyloid load between individuals with and without DS [59–61, 65]. Amyloid immunocytochemistry performed by our group failed to reveal any significant differences in caudate or putamen amyloid plaque load and number between demented and nondemented adults (40–60 year old) with DS [34]. Striatal plaque loads determined using either the 6E10 (APP/Aβ) antibody or X-34 histochemistry, which reveals β-pleated sheet structures, displayed higher mean values than Aβ and 6-CN-PiB (tissue stain for the PiB compound) in nondemented DS cases [34]. Recently, elevated striatal [C-11]PiB binding was found in 13 of 16 people with DS with cognitive impairment or dementia, whereas similar labeling was observed in only 7 of 33 cognitively stable individuals [59]. Further clinical pathological studies are needed to develop a striatal amyloid signature that distinguishes demented from nondemented people with DS [55].
It is widely accepted that amyloid-related peptides are toxic resulting in the formation of neuropil threads, dystrophic dendrites, and axons in the AD brain [66, 67]. Interestingly, we did not observe tau, choline acetyltransferase (ChAT), and tyrosine hydroxylase dystrophic neurites and neuropil threads (NTs) associated with striatal amyloid plaques in demented or nondemented individuals with DS compared to frontal cortex (FC) plaques [34]. Not all striatal ChAT positive interneurons located adjacent to Aβ42 plaques or containing NFTs displayed an abnormal morphology in either DS phenotype (see Fig. 5E and F) compared to the shrunken appearance of striatal cholinergic neurons in AD (see Fig. 5G). Striatal amyloid is an area that requires further investigation using both imaging and histopathological methods to determine its role in the pathobiology and onset of dementia in DS and AD [55].
Tau deposition in AD and DS
In addition to amyloid pathology, both the AD and DS brain develop age-related tau-containing NFT and neuritic thread (NT) pathology (see Fig. 5) [12]. Tau, a microtubule protein associated with normal cytoskeletal function, transitions from a relatively soluble state into filamentous aggregates in AD and DS [68–71]. NFT pathology occurs at a later age than Aβ in DS [11, 72–74], exhibits an age-related pattern similar to AD [75], and NFT burden is tightly correlated with cognitive decline and dementia in both AD and DS [34, 76–79].
Fetal tau in DS
Despite the age-associated onset of tau pathology in the adult AD and DS brain, tau deposition in the fetal DS brain remains underinvestigated. However, different abnormal tau phosphorylation events have been demonstrated during fetal development (14–28 gestation weeks) using tau epitope-specific antibodies that revealed tau within white matter tracts (e.g., cerebellar peduncles and internal capsule) [80, 81] suggesting early axonal transport defects, a common theme in tauopathies [82]. Interestingly, we observed a band of phosphorylated CP13 (Ser202) and PHF-1 (Ser396) tau immunoreactivity situated between the external granular (or germinal) and molecular layers of the cerebellum during early postnatal development (Fig. 4). Although this tau band is similar to that reported in the outer two-thirds of the molecular layer of the hippocampus in individuals with DS over the age of 31 years, these profiles were not positive for PHF-1 [48] as seen in the fetal cerebellum. Although the functional significance of these bands of tau remains unclear, this fine dust-like immunoreactivity may represent synaptic terminals related to neuronal sprouting or pruning during both development and aging [83]. Recently, using neuron-derived extracellular vesicles (NDEs) isolated from blood [84, 85], increased levels of p-Tau and Aβ were found in NDE cargo obtained from children with DS as early as 8 years of age, lending support to the suggestion that putative pathologic tau is initiated in brain decades prior to symptom [86] and lesion onset.

Tau pathology in the adult AD and DS brain
Tangle bearing neurons appear within neocortical layer III, V, and VI pyramidal, entorhinal cortex layers II and IV neurons. Cortical NFTs display a flame-like shape compared to a more globose morphology associated with subcortical neurons such as those seen in the cholinergic neurons of the nucleus basalis in both the adult DS and AD brain [87–89] (Fig. 6M, N, Q). Recently, we reported that neuritic pathology, found in these regions, is an excellent correlate of cognitive decline during the progression of AD suggesting that axonal and dendritic pathology precedes neuron dysfunction [90]. Furthermore, we reported greater neuritic pathology in the frontal cortex of demented compared to nondemented DS cases suggesting that axonal integrity also plays a key role in dementia onset in DS [34]. These observations suggest that despite the appearance of Aβ at an early age, NFT pathology is more closely linked to cognitive decline and dementia in DS similar to AD [76–78]. Braak and colleagues have staged the progression of NFT pathology in AD using the tau AT8 antibody. Braak staging revealed a progression of NFT pathology that begins within the entorhinal and transentorhinal cortex (stage I) spreading to the hippocampus (stage II), temporal cortex (stage III), then to other neocortical regions (stage IV), and finally reaching visual association cortex (stage V–VI). Recently, this schema was modified and stages prior to entorhinal cortex involving subcortical neurons containing tau located within noradrenergic locus coeruleus (LC), serotonergic dorsal raphe (DRN), and cholinergic nucleus basalis neurons [91, 92] were described as pretangle or prodromal stages “a” and “b” [93, 94], independent of cortical tau pathology and these areas are implicated in cognitive decline in AD [87, 95]. Recently, tau (AT8) positive NFTs or NTs were not found within the entorhinal cortex, hippocampus, or neocortex in a subset of DS cases 35 years and under plus a single 39, 50, and 60-year-old subject, which were considered Braak stage II–IV [38] indicative of mild to moderate tangle pathology within the medial temporal lobe (MTL) [96, 97]. Remaining cases over the age of 50 showed moderate to numerous NFTs in all brain regions examined similar in appearance, distribution, and degree to AD, which were scored as Braak stage V or VI [45] indicative of advanced MTL and neocortical NFT pathology [96] that is associated with dementia in AD. Davidson and colleagues [38] reported tau pathology in LC and DNR nuclei only after 35 years of age in DS. These investigators also evaluated the substantia nigra, which contains dopaminergic neurons, and found no tau pathology in any case less than 50 years of age. After this age NFTs and neuropil threads increased similar to that described in older people with AD [98]. A detail application of Braak staging needs to be applied to demented and nondemented individuals with DS.
Evolution of tau pathology in AD and DS
To better understand the development of NFTs in adults with AD, a linear model has been proposed that can be tracked by antibodies that mark tau epitopes during the early, intermediate, and late stages of NFT formation [71, 90, 99, 100]. This model has been applied to the evolution of NFTs in the cortex, hippocampal complex, and cholinergic basal forebrain (CBF) in AD [71, 90, 100, 101]. Recently, this paradigm was used to determine whether changes in posttranslational tau epitopes in NFTs differ in the frontal cortex (FC) and striatum between DS without (DSD −) and with (DSD +) dementia. This study used antibodies against early tau phosphorylation epitopes (pS422 and AT8), structural/conformational tau changes (Alz50 and MC1), and tau truncation (Tau C3 and MN423) applied to FC layer V and VI pyramidal neurons in DSD − and DSD + cases (Fig. 5A–D). NFT and NT profiles positive for phosphorylation (pS422) and truncation (TauC3) were more abundant compared to conformational (Alz50 or MC1) NFTs in layer V and VI neurons in both DS groups. However, significant differences in pS422 and TauC3 compared to Alz50 positive NFT densities were found in the DSD + group. In addition, pS422 positive NT numbers were significantly higher than MC1 and Alz50 positive NTs and the number of AT8 reactive NTs was greater than those containing MC1 only in DSD + cases. Furthermore, NFT counts revealed that only the number of NFTs and NTs containing AT8 was significantly higher in DSD + compared to DSD −. By contrast, MN423-positive NFTs were seen in 2/6 DSD − compared to 6/8 DSD + cases mainly in layer II and III, with the exception of two female DSD + cases (59 and 45 years old) where NFTs were found in layer V and VI. Triple-labeled NFTs (AT8 + pS422 + Alz50, TauC3 + pS422 + Alz50) and dual reacted (TauC3 + pS422) were more frequent in DSD + compared to DSD − cases (Fig. 5A–D). The number of dual-labeled FC NFTs (Alz50 + pS422 and Alz50 + TauC3) compared to triple-labeled (AT8 + pS422 + Alz50 and TauC3 + pS422 + Alz50) positive neurons was lower in DSD − and DSD +, respectively, suggesting that tau phosphorylation and truncation events precede conformational events in FC neurons in DS.

Striatal NFTs containing tau phosphorylation, conformational and truncated (pS422, AT8, Alz50, MC1, and TauC3) epitopes were detected in all DSD + cases, whereas conformational (Alz50 and MC1) NFTs were found in a subset of DSD − cases. MN423-positive profiles were not detected in the striatum of either DS group. Since there were no significant differences in pS422, AT8, TauC3, MC1, and Alz50 positive NFTs in the striatum between DS groups, conformational alterations may be an early event in DS that is discontinuous as the amino terminus is eliminated during the evolution of tau pathology [34]. In contrast, TauC3 NFT density was significantly reduced compared to pS422, but comparable to Alz50 in the putamen in both DS groups, revealing a less advanced stage of NFT pathology. In this study, several striatal cholinergic interneurons displayed intracellular aggregates of tau (AT8) resembling skeins of yarn (Fig. 5F) in both DS groups but the cellular morphology remained unchanged compared to shrunken NFTs in DS and AD (Fig. 5G). These observations suggest that cholinergic NFT containing interneurons were at an early pathological stage or were resistant to tau toxicity.
Recent evidence suggests that prefibrillar amyloid oligomeric forms are a more likely neurotoxic species of tau [101, 102]. In this regard, we examined the accumulation of tau oligomeric species in CBF neurons during the progression of AD and found that tau oligomer formation is an early event in tangle evolution [101, 102] suggesting that aberrant phosphorylation primes the tau protein for additional phosphorylation and conformational changes [90, 95] that facilitate oligomerization [96]. Whether similar events occur in striatal neurons in individuals with DS requires investigation. In the future, the accumulation of tau burden using PET imaging will assist in defining the role that tau plays in the onset of dementia in both AD and DS. Rafii and colleagues [79] have shown a similar tau binding pattern and progression in non-DS AD cases, which were related to cognitive decline (see also Chapter 9). Further longitudinal studies are needed to assess the interaction of tau with cognitive impairment in DS.
Other pathologies in AD and DS
Transactive response DNA-binding protein 43 (TDP-43) inclusions in DS
TDP-43 was identified as a 414 amino acid (molecular mass 43 kDa) binding partner to the TAR regulatory element in HIV virus type 1. TDP-43 is a nuclear versatile RNA/DNA binding protein involved in RNA-related metabolism, particularly in RNA splicing. Phosphorylated TDP-43 proteins, in the form of neuronal cytoplasmic inclusions, a pathological hallmark of amyotrophic lateral sclerosis (ALS) are also found in other neurodegenerative diseases including in some, but not all AD (30%–40%) and FAD [103–105] cases. In both AD phenotypes, phosphorylated TDP-43 inclusions appear mainly in temporal cortex, hippocampus (e.g., dentate granule cells and CA pyramidal fields), subiculum, entorhinal cortex, amygdala, and frontal cortex in more advanced AD [45, 104–107]. By contrast, a few reports have described a small number of older DS cases with AD pathology (age range 54–62) that contained TDP-43 inclusions in the hippocampus, amygdala, and temporal cortex similar to AD [45, 105]. We observed nuclear nonphosphorylated TDP-43 immunostaining in layers V and VI of the orbital cortex in a 1-year-old female with DS (Fig. 5H), a 46-year-old male DS with dementia (Fig. 5I), and an 83-year-old AD (Fig. 5J) case. TDP-43 reactivity was also seen in hippocampal subfields in a 46-year-old male DS case with dementia (Fig. 5K) including granule (Fig. 5L) and CA1 pyramidal (Fig. 5M) neurons, while cytoplasmic nonphosphorylated TDP-43 positive inclusions were not observed in these DS cases. Recently, a staging schema was applied to TDP-43 distribution in AD [107] but has not yet been undertaken in DS. The functional significance of cytoplasmic TDP-43 inclusions remains a highly debated question. Some have argued that TDP-43 intracellular deposits in AD and DS are more related to an aging process than to AD alone [105]. Although there is a strong relationship between these inclusions and cognition in AD, only 30%–40% of AD and 7% of DS cases examined display TDP-43 immunoreactivity [105], arguing against a primary role in the onset of dementia in most people with these disorders.
α-Synuclein inclusions in DS
Lewy bodies (LB), which contain ubiquitin and a-synuclein, are classic lesions of Parkinson’s disease but are also found in AD and dementia with LBs (DLB) [108]. Tissue from DS immunostained for α-synuclein revealed the greatest number of LBs and Lewy neurites (LN) in the amygdala [108]. We found LBs to be concentrated in the basal medial, lateral, and accessory basal amygdaloid nuclei as well as scattered Lewy Neurites (LNs) with a heavy concentration in white matter tracts (Fig. 5O and Q). LBs have been reported, to a lesser degree, in orbital frontal, entorhinal, and temporal cortex as well as in the substantia nigra, nucleus basalis of Meynert and hypothalamus (Fig. 5N and P), and an occasional LB in DS cases 50 years or older but not in very young cases [45, 108–110]. Although LBs occur mainly in neurons lacking NFTs, an occasional dual-labeled neuron was reported in the amygdala and adjacent periamygdaloid cortex in DS [108]. LBs were found in 50% of DS cases with AD pathology but not in DS without AD and to a lesser degree in FAD suggesting a role for the APP gene as well as trisomy in the biological processes driving the development of this pathology [108]. We found LB pathology in both demented and nondemented adults with DS aged 40–60 years (Fig. 5N–Q). However, the factors that determine the formation of synuclein containing LB pathology and their role in the behavioral/functional decline seen in DS remain unknown.
Cholinergic basal forebrain dysfunction in DS and AD
Of the many subcortical brain regions implicated in cognitive impairment the neurons located within the nucleus basalis of Meynert (nbM), which innervate the entire cortical mantle [111, 112], display NFT pathology even prior to that seen in the transentorhinal and entorhinal cortex in AD [94] have received extensive investigation in AD but to a lesser degree in DS [31, 113–121]. Outcomes from brain imaging studies have strengthened the importance of this region in AD including altered basal forebrain signaling in relation to cognitive decline [122], propagation of cortical atrophy early in the evolution of AD [123], as a presymptomatic biomarker for AD [124, 125] and a predictor of memory impairment in AD [123]. The increase in [F-18]FDG PET signal within the nucleus basalis in MCI compared to control and AD [126] suggests a transient cholinergic upregulation, similar to the neuroplastic increase in ChAT activity found in the frontal cortex [127] and hippocampus [128] in MCI. This renewed interest in the CBF projection system demonstrates a critical need to better understand the mechanistic factors driving the selective vulnerability of the CBF connectome and its association to dementia in DS. Since there are numerous reviews that discuss the structural and functional correlates of CBF degeneration in AD [87, 120, 129–133], here we will concentrate on the pathobiology of this system in DS.
Prenatal cholinergic basal forebrain connectome in DS
The neurotypical human newborn brain displays an intact CBF system [134, 135]. However, whether individuals with DS are also born with an intact CBF system remains an intriguing area of research. To investigate the CBF system during gestation we examined several neurotypical and DS embryonic brains [134, 136]. Due to methodological caveats related to the use of ChAT antibodies to visualize the CBF system in paraformaldehyde fixed fetal tissue, we employed antibodies that recognize the low affinity nerve growth factor receptor (p75NTR) and the high affinity (TrkA) cognate receptor for the trophic substance nerve growth factor (NGF), both are excellent markers for the CBF connectome in fetal [134–136], young, aged, and diseased human brain [114, 137]. The neurotypical fetal human brain displayed extensive p75NTR immunostaining at least at embryonic weeks 21 and 27 (Fig. 6A and B) [134, 136] and TrkA reactivity at least to embryonic week 34 [134]. At these fetal ages, CBF p75NTR immunoreactive neurons appear multipolar and display varicose beaded axons, dendrites (Fig. 6D), as well as straight fibers (Fig. 6E). However, trisomy results in an altered cholinergic/p75NTR phenotype during gestation including reduced reactivity within subregions of the CBF at DS embryonic week 18 (Fig. 6C) suggesting a defect in the cellular machinery producing p75NTR. Although many CBF neurons exhibit a similar morphology in DS to the neurotypical brain at fetal week 18 (Fig. 6F), others appear dystrophic with blunted dendrites (Fig. 6G) and fibers appear broken with swollen processes (Fig. 6I). At both prenatal neurotypical (20 weeks) and DS (18 weeks), p75NTR immunoreactive fibers coursed within the external capsule to reach the subplate zone of the developing cortex (Fig. 6J and K). Although a similar distribution of p75NTR immunoreactivity was seen in the subplate zone in the neurotypical and DS cortex (Fig. 6J and K) the later displayed less neurons and swollen and blunted neurites (Fig. 6K). Recently, we immunostained tissue containing the nbM from a 6-month-old female individual with DS with an antibody against p75NTR and observed large multipolar and elongated bipolar cholinergic neurons (Fig. 6L) similar to that seen in normal (Fig. 6O) and aged (Fig. 6P) adults as well as children with DS [26]. Although it remains to be determined whether numerical differences exist in CBF neuron number and cellular phenotype between prenatal, newborn, young, and aged neurotypical and DS cases, the current findings suggest that the embryonic DS CBF projection system already displays structural alterations similar to the degenerative phenotype seen in AD and older individuals with DS [115, 138].

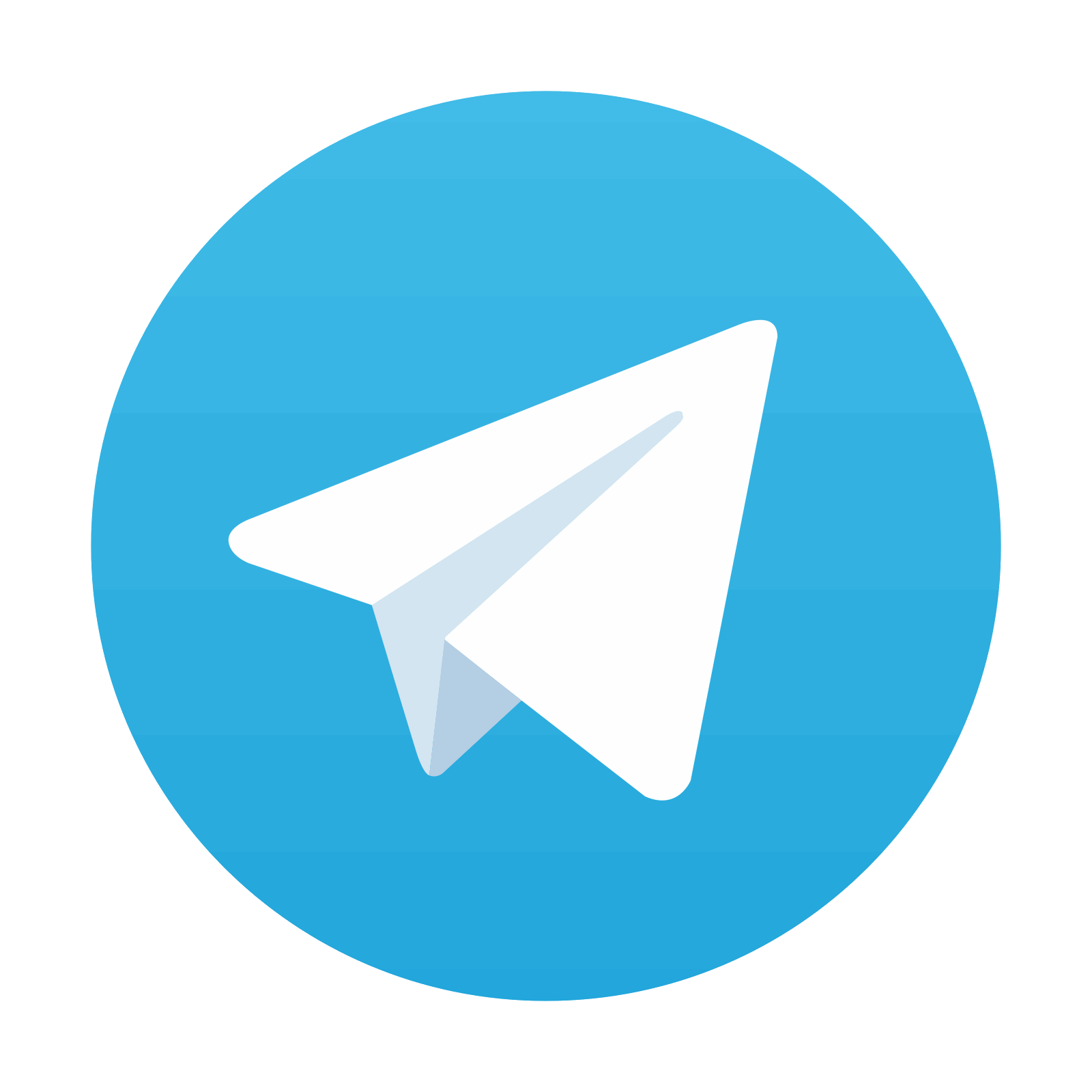
Stay updated, free articles. Join our Telegram channel

Full access? Get Clinical Tree
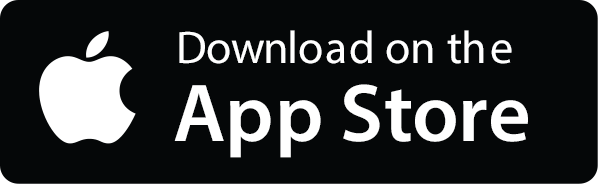
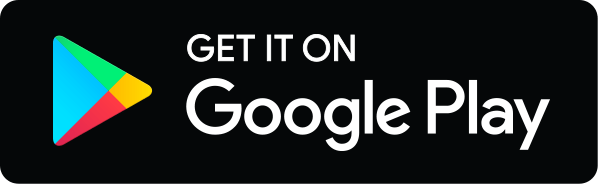