Chapter 101 Anatomy and Physiology of Upper Airway Obstruction
Normal Upper Airway Anatomy
The upper airway has been separated into three regions: the nasopharynx, which includes the posterior margin of the nasal turbinates to the posterior margin of the hard palate; the oropharynx, subdivided into the retropalatal region (the posterior margin of the hard palate to the caudal margin of the soft palate) and the retroglossal region (the caudal margin of the soft palate to the base of the epiglottis); and the hypopharynx, which includes the base of the tongue and epiglottis to the larynx (Fig. 101-1). The majority of patients with OSA manifest upper airway narrowing and closure during sleep in the retropalatal region, the retroglossal region, or both.1,2
In order to evaluate airway closure in patients with OSA it is important to understand how pharyngeal wall structures mediate changes in airway size. The anterior wall of the oropharynx is formed primarily by the soft palate and tongue, and the posterior wall of the oropharynx is composed primarily of the superior, middle, and inferior constrictor muscles. The lateral oropharyngeal walls are formed by several different structures including oropharyngeal muscles (hyoglossus, styloglossus, stylohyoid, stylopharyngeus, palatopharyngeus, palatoglossus, and the superior, middle, and inferior pharyngeal constrictors), lymphoid tissue (palatine tonsils), and adipose tissue (parapharyngeal fat pads). The mandibular rami bound all the structures that form the lateral pharyngeal walls (Figs. 101-2 and 101-3).
Static and Dynamic Properties of the Normal Pharyngeal Airway
The mechanical behavior of the pharyngeal airway under passive conditions—that is, in the absence of pharyngeal muscle activity—can be described in terms of the relationship between cross-sectional airway area (A) and transmural pressure (Ptm).3 As depicted in Figure 101-4A, transmural pressure is the difference between intraluminal pressure (PL) and tissue pressure (Pti):
An increase in transmural pressure, caused either by more-positive intraluminal pressure or more-negative tissue pressure, distends and enlarges the airway area. Conversely, a decrease in transmural pressure, caused either by more-negative intraluminal pressure or more-positive tissue pressure, narrows the airway.
The mechanical characteristics at a particular region of the passive pharynx are revealed in a plot demonstrating the relation between transmural pressure and intraluminal cross-sectional area (see Figure 101-4B). This relationship is referred to as the tube law and describes the dependence of cross-sectional area on transmural pressure. The closing pressure (Pclose) is the transmural pressure when cross-sectional airway area reaches zero (i.e., complete obstruction), and the point at which the curve reaches a plateau is the maximum airway area. The slope of the line at any point in the relationship (ΔA/ΔPtm) is the effective compliance at that particular transmural pressure. A number of mechanical factors (Box 101-1) influence the upper airway to cause it to be fully open, narrowed, or closed. These factors, classified as static and dynamic, interact with the tube law of the pharynx to determine, at any time, the cross-sectional area of the airway.
Static Factors Influencing Behavior of the Normal Pharyngeal Airway
Surface Adhesive Forces
Clinical observations and studies in anesthetized animals indicate that surface adhesive forces between opposed luminal surfaces can contribute to airway patency and closure.4–6 During nasal breathing with the mouth closed, surface adhesive forces help maintain the soft palate in apposition to the base of the tongue and promote contact of the tongue with the mucosa of the oral cavity. Mouth opening potentially destabilizes the airway by freeing the mucosal attachments of the tongue and soft palate and allowing these now freely moving structures to relocate posteriorly and compromise the pharyngeal airway. Surface adhesive forces can also make restoration of airway patency more difficult and might explain why the pressure needed to open an already closed airway (opening pressure) is greater than the closing pressure. Although surface adhesive forces are an important determinant of airway patency, reduction in these forces by administering surfactant to the upper airway mucosa results in only a relatively modest reduction in airway collapsibility during sleep.4
Neck and Jaw Position
Studies indicate that neck flexion under passive conditions tends to close the airway, and neck extension acts to open it.7 The retropalatal and retroglossal regions of the pharyngeal airway are narrowed as the neck is flexed. Jaw position has also been documented to alter the size of the upper airway. Opening the jaw slightly can actually increase the size of the pharynx by providing more room in the oral cavity for the tongue. This may be particularly important if the tongue is large relative to the oral cavity. However, progressive opening of the jaw leads to posterior movement of the genial tubercle of the mandible: The genial tubercle moves closer to the posterior pharyngeal wall because the mandibular condyle of the temporomandibular joint is considerably rostral to the plane of the mandible (Fig. 101-5). This posterior movement of the genial tubercle of the mandible with mouth opening causes the tongue and hyoid apparatus to move posteriorly, and thereby narrows the pharyngeal airway.
Tracheal Tug
Increases in lung volume are thought to increase pharyngeal cross-sectional area, reduce closing pressure, and stiffen the upper airway.8,9 This action may be exerted through axial forces in the trachea, called tracheal tug. Increasing lung volume causes a caudal displacement of the intrathoracic trachea that, in turn, exerts caudally directed forces on the upper airway. The resulting passive axial tension in the pharyngeal wall tends to open the pharynx. There are at least four mechanisms by which caudal traction on the upper airway may improve airway patency (Fig. 101-6).10 As the upper airway is pulled toward the thorax, folding may be reduced in the walls of both the larynx and oropharynx. Second, stretching should stiffen the upper airway and make it more resistant to collapse. Caudal displacement of fat and other structures surrounding the pharynx can reduce extrinsic compression of the airway. Finally, caudal traction can improve airway patency through its mechanical effect on the hyoid apparatus.
Gravity
Gravity also has an important influence on pharyngeal airway patency and it is common for patients with OSA to have a higher apnea-hypopnea index in the supine than in the nonsupine position. When the patient is supine, gravity can help to narrow the pharyngeal airway by pulling the tongue and soft palate in a posterior direction.11
Dynamic Properties of the Normal Pharyngeal Airway
The upper airway has also been modeled as behaving like a Starling resistor.12 Starling resistor describes a highly collapsible tube having infinite compliance (e.g., more floppy) at one transmural pressure and low compliance (e.g., more stiff) at transmural pressures that are above and below this pressure. The tube is completely closed at one luminal pressure and completely open at a higher luminal pressure. The luminal pressure at which the airway shifts from fully open to fully closed (i.e., the point of infinite compliance) is determined by the extramural pressure and is referred to as the critical pressure (Pcrit).
Factors Influencing the Dynamic Properties of the Passive Pharyngeal Airway
Dynamic Compliance
During inspiration, the decrease in intraluminal pressure at any point in the upper airway interacts with the dynamic compliance of that segment of the upper airway.13 If intraluminal pressure at the beginning of inspiration is a value that lies on the steep portion of the pressure-area relationship, the upper airway narrows as intraluminal pressure decreases during inspiration. The degree to which pharyngeal cross-sectional area decreases depends on the dynamic compliance of the upper airway. This mechanical property also influences the likelihood of further airway collapse. Specifically, narrowing during inspiration due to a decrease in intraluminal pressure might decrease the area significantly which, in turn, increases the velocity of gas flowing through that segment. This results in further reductions in intraluminal pressure because of the conversion of static to kinetic energy with decreased distending pressure. Such a decline in luminal pressure, in turn, tends to further decrease airway area. This sequence describes dynamic narrowing of the upper airway, which is typically observed under normal conditions if the pharyngeal muscles are hypotonic. In early expiration (positive airway pressure due to chest wall recoil), airway caliber increases, and toward the end of expiration (reduction in positive airway pressure and the upper airway dilator muscles remain inactive), the airway narrows.1 Patients with OSA are at risk for airway closure at the end of expiration and in inspiration.
Ventilatory Control
Alterations in ventilatory control can also influence the upper airway and promote upper airway obstruction. In humans, arterial oxygen and carbon dioxide levels are tightly controlled by various feedback systems involving chemoreceptors, intrapulmonary receptors, and respiratory muscle afferents. Because ventilatory control is regulated by feedback systems, these systems can become unstable (waxing and waning ventilation). Disturbances in these respiratory feedback systems are generally characterized by an increase in loop gain, which can augment periodicities in breathing patterns and in some persons produces associated periodic upper airway obstruction. (See Chapter 100 for a complete description of loop gain.)
Pharyngeal Muscles
Activation of the Pharyngeal Muscles
Many of the 20 or more skeletal muscles surrounding the pharyngeal airway are phasically activated during inspiration, which helps to dilate the airway and stiffen the airway walls.14,15 As shown in Figure 101-7, the pharyngeal muscles have complex anatomic relationships that help regulate the position of the soft palate, tongue, hyoid apparatus, and posterolateral pharyngeal walls. Contraction of specific muscles within these groups can have antagonistic effects on the pharyngeal airway. For example, contraction of the palatal levator palatini muscle, along with the superior pharyngeal constrictor, closes the retropalatal airway, but contraction of other palatal muscles, the palatopharyngeus and glossopharyngeus, opens the airway in the retropalatal region. Similarly, the extrinsic tongue muscles can have antagonistic effects; namely, the genioglossus and geniohyoid protrude the tongue and the hyoglossus and styloglossus retract it.
In addition, pharyngeal muscles can have different effects when activated in concert as opposed to when activated individually. Co-activation of the hyoid muscles is a particularly good example of this phenomenon (Fig. 101-8; see Fig. 101-7B). The hyoid bone in humans, unlike that in other mammals, does not articulate with any other bony or cartilaginous structure. The position of the hyoid bone is determined by the muscle attachments to this floating bony structure. Muscles inserting on the hyoid include the geniohyoid and genioglossus. Contraction of these muscles pulls the hyoid in a rostral and anterior direction. Strap muscles originating from the sternum (sternohyoid) and thyroid cartilage (thyrohyoid) also insert on the hyoid and pull it in a caudal direction. With simultaneous contraction of all four muscles, the resultant force vector acting on the hyoid is directed caudally and anteriorly. This combined effect moves the anterior pharyngeal wall outward, can stiffen the lateral pharyngeal walls, and promotes upper airway patency. Another example of coactivation of muscles and upper airway patency involves the tongue. Evidence indicates that simultaneous activation of the antagonistic protrudor and retractor tongue muscles, as occurs under hypercapnic and hypoxic conditions, has a synergistic effect in promoting upper airway patency.16
The action of an upper airway muscle depends not only on whether other muscles are simultaneously active but also on its length–tension and force–velocity characteristics at the time of activation. For instance, opening the mouth decreases genioglossus and geniohyoid muscle length, which can affect upper airway caliber. Similarly, neck flexion changes the position of the hyoid bone; altering the anatomic relationships of a variety of muscles acting on this structure and shifting the vector of their forces in a more caudal direction. Other evidence suggests that a particular pharyngeal muscle can have different mechanical effects on the airway depending on the size of the airway at the time of muscle activation.17,18 The ability of a given muscle to produce different mechanical effects may be due to changes in muscle fiber orientation with concomitant changes in airway size and shape. In addition, the timing of muscle activation relative to the phase of respiration may play a role in determining the mechanical effects of such activation.19
Factors Modulating Pharyngeal Muscle Activation
Activation of pharyngeal muscles can alter the mechanical characteristics of the upper airway. The effect of pharyngeal dilator muscle activation on the tube law of the pharynx is shown in Figure 101-8. Under active conditions, the pressure–area relationship is shifted upward and to the left. At any given transmural pressure, muscle activation increases airway area and stiffens the airway, that is, decreases effective compliance. The effect of muscle activation on the tube law is quantified by the term Pmus, the effective pressure exerted by muscle activation, equivalent to the change in transmural pressure required to yield the equivalent change in area on the passive curve (see Fig. 101-8). Under certain conditions, pharyngeal dilator muscles display bursts of inspiratory activity superimposed on tonic activity. Alcohol, sleep deprivation, anesthesia, and sedative-hypnotics suppress respiratory-related pharyngeal muscle activation.20 Additional factors that modulate respiratory-related activity of pharyngeal airway motoneurons include changes in state, proprioceptive feedback, and chemical drive (Fig. 101-9).
Changes in State
Perhaps the most convincing evidence supporting the overall importance of neuromuscular activity on airway patency is that apneas and hypopneas occur during sleep. That modification of neuromuscular factors (e.g., decrease in genioglossus activity) by sleep is a normal physiologic phenomenon can be inferred from measurements of supraglottic resistance, the airflow resistance extending from the nares to the region above the glottis in normal subjects in whom supraglottic resistance increases fourfold to fivefold with sleep onset (e.g., from 1 to 2 cm H2O/L/sec during wakefulness to 5 to 10 cm H2O/L/sec during sleep).21,22 Supraglottic airway resistance is abnormally high in OSA patients during wakefulness and increases even further with sleep onset. The decrease in upper airway caliber that occurs with sleep onset is explained by a state-dependent reduction in neural output to upper airway muscles, which causes a decrease in Pmus at one or more sites within the pharyngeal airway. EMG recordings of pharyngeal muscles, such as the genioglossus and tensor palatini, confirm this decrease in pharyngeal muscle activity during the transition from wakefulness to sleep.23,24 An even more pronounced reduction in motor output to pharyngeal muscles occurs in rapid eye movement (REM) sleep, particularly in phasic REM.25,26 Thus, there is compelling evidence that the neural output to pharyngeal dilator muscles is decreased during sleep.
Sensory Modulation of Pharyngeal Muscles
Neurosensory feedback from thoracic and upper airway receptors can modulate motor output to pharyngeal muscles.27 During NREM sleep and general anesthesia in animals, withdrawal of vagally mediated phasic volume feedback by tracheal occlusion during inspiration results in an immediate large augmentation in motor output to many upper airway and chest wall inspiratory pump muscles.28–30 Introduction of subatmospheric pressure into an isolated, sealed upper airway in spontaneously breathing tracheostomized animals precipitates neurally mediated muscle activation.31,32 It is believed that upper airway receptors located superficially in the airway wall mediate this reflex activation because it is not present following administration of topical anesthesia.33
The majority of upper airway respiratory-related afferents are located in the upper trachea and larynx and carried in the internal branch of the superior laryngeal nerve. Sensory information from the upper airway is also transmitted in the glossopharyngeal and trigeminal nerves.34 Both intrathoracic and upper airway sensory afferents can also reduce motor output to the thoracic inspiratory muscles, thereby increasing intraluminal pressure below the site of airway obstruction.35 The effects of this reflex on upper airway and respiratory pump muscles could represent a powerful defense mechanism for maintaining upper airway patency during sleep. Presumably, neural reflex activation of pharyngeal muscles by upper airway and thoracic receptors would be initiated by upper airway obstruction and would tend to compensate for airway obstruction by dilating and stiffening the pharynx. However, evidence indicates that these neurally mediated reflexes to protect upper airway patency are blunted in sleeping humans.36
Repetitive mechanical stress on pharyngeal airway structures from recurrent episodes of apnea and snoring on the upper airway appear to have neurosensory consequences. Evidence indicates that there is a sensory-neural abnormality in patients with sleep apnea with impaired upper airway sensation, which is partially reversible with continuous positive airway pressure (CPAP).37–39 In addition, studies have shown the importance of upper airway reflexes that increase upper airway caliber.36 These reflexes are activated by negative inspiratory pressure and may be attenuated by the sensory impairment of the upper airway. Repeated episodes of snoring and vibration at night have also been thought to lead to progressive local neuropathy.40,41 Both the upper airway sensory abnormalities and neuropathy could explain the progressive worsening of sleep apnea over time.
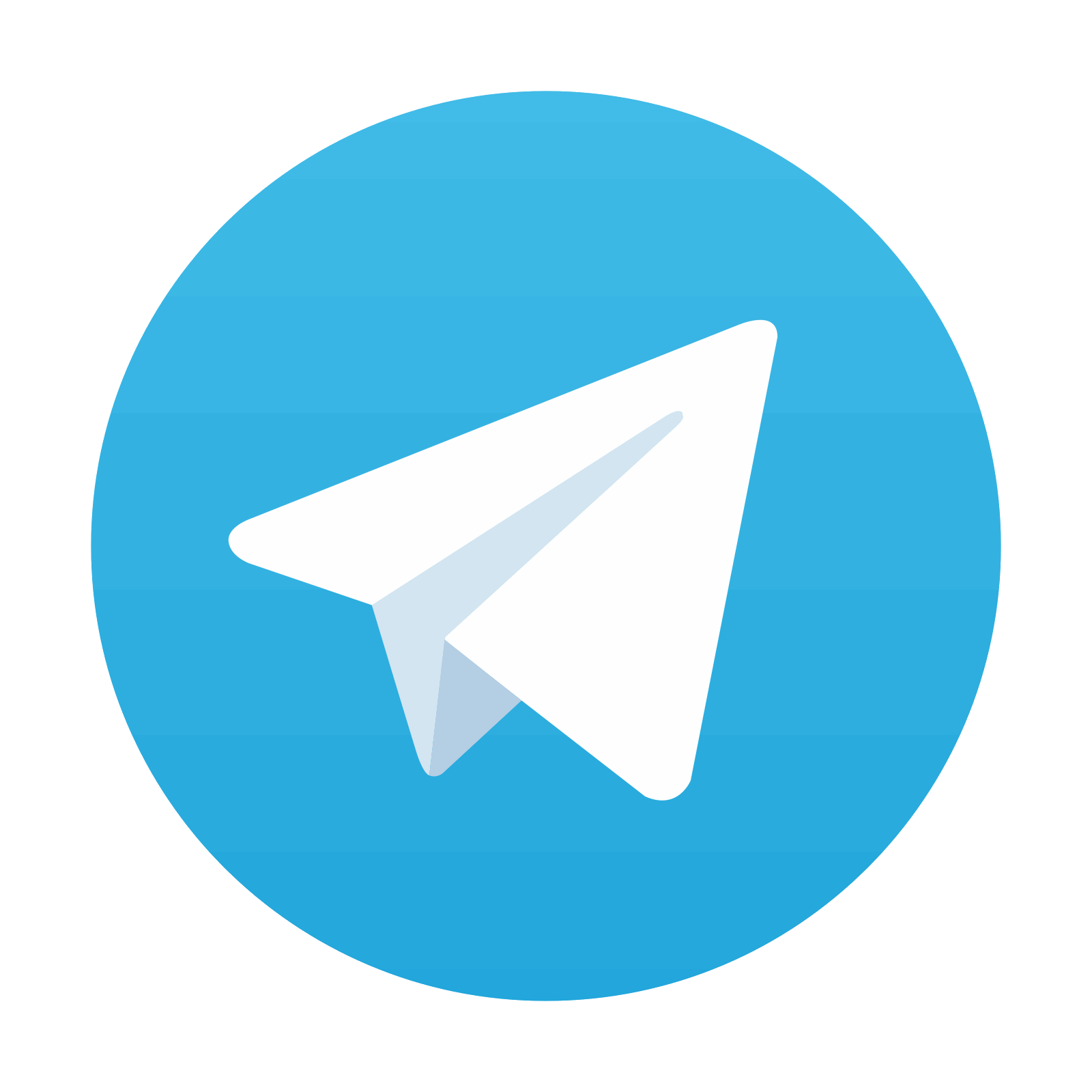
Stay updated, free articles. Join our Telegram channel

Full access? Get Clinical Tree
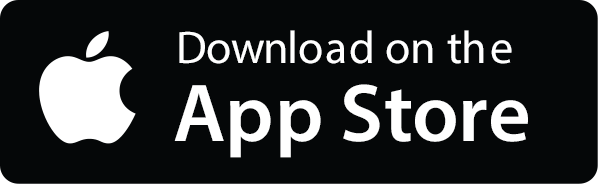
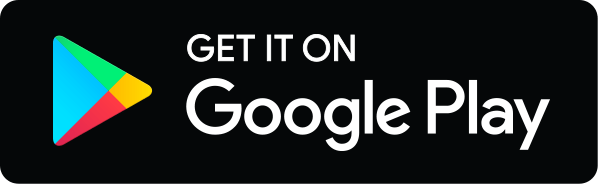