CHAPTER 104 Angiogenesis and Brain Tumors
Molecular Targets and Molecular Scalpels
The treatment of brain tumors, especially glioblastoma, is being transformed by the recent introduction of a new class of therapeutic agents: molecular-targeted, antiangiogenic drugs. The prototype, bevacizumab (Avastin, Genentech, South San Francisco, CA), a humanized monoclonal antibody directed against vascular endothelial growth factor (VEGF-A), received U.S. Food and Drug Administration (FDA) approval in 2004 for the treatment of metastatic colorectal carcinoma,1–3 followed by approvals for non–small cell cancer of the lung and metastatic breast cancer. In view of compelling data of efficacy (Table 104-1),4–6 accelerated FDA-approval is anticipated for recurrent glioblastoma. The National Comprehensive Cancer Network (NCCN) has endorsed the bevacizumab-irinotecan combination for recurrent glioblastoma7 and, more recently, bevacizumab as a single agent for recurrent malignant glioma.8,9 In light of these groundbreaking events, this chapter examines (1) the scientific foundation of antiangiogenesis therapy,1,10–25 (2) practical aspects of using antiangiogenics,21,25 (3) advances in neuroimaging and biomarkers to monitor therapeutic responses,21,25 (4) potential new applications of antiangiogenics in neurosurgery and neuro-oncology (e.g., the treatment of radiation-induced necrosis),26 and (5) antiangiogenic agents in phase II and phase III clinical trials (Table 104-2).
Historical Perspective
More than 100 years ago, Paul Ehrlich introduced the concept of targeted therapy in the form of a “magic bullet”—a drug that would go straight to its intended target. He coined the term chemotherapy and described (1) specific cellular receptors, (2) the blood-brain barrier, (3) neurotoxicity of drugs, (4) rationally “designed” drugs, and (5) animal models as part of a systematic approach to drug discovery—thereby creating the foundations of experimental therapeutics in oncology.27–29
A century later, the pioneering work of Judah Folkman (Fig. 104-1) developed the field of angiogenesis research that is revolutionizing ophthalmology, oncology, and other angiogenesis-dependent diseases.30 More than 500 million people worldwide are predicted to benefit eventually from the pharmacologic control of angiogenesis.30–32 In 2004, the FDA commissioner announced that in addition to the traditional three modes of cancer therapy—surgery, radiotherapy, and chemotherapy—“antiangiogenic therapy can now be considered the fourth modality of cancer treatment” (Fig. 104-2).30–33 We use the terms antiangiogenic therapy, angiosuppression,14,34 and angiotherapy35 interchangeably.
In Folkman’s seminal paper,10 he hypothesized that solid tumor growth is dependent on angiogenesis, tumors secrete a diffusible angiogenic factor, and blockade of the factor would render tumors harmless, existing in a persistent state of dormancy.10,30 These concepts were supported by observing tumors, including brain tumors, transplanted into the avascular vitreous body.13,36 Once tumors acquired a blood supply, there was a 19,000-fold burst in tumor volume, supporting the concept of an angiogenic switch (Fig. 104-3).13,36 The first quantitative, translational study of angiogenesis11 compared microvascular density in a range of human neoplasms and concluded that glioblastomas would be uniquely susceptible to angiosuppression because of the extremely high degree of neovascularization.37
Proof-of-principle that angiogenesis could be inhibited, and tumor growth controlled, was demonstrated by Judah Folkman and Henry Brem,12 accelerating the discovery of a series of biochemical stimulators and inhibitors of angiogenesis.30,33 The decisive discovery of a protein, termed vascular permeability factor by Harold Dvorak,38 and vascular endothelial growth factor by Napoleone Ferrara,2 led to the development of a monoclonal antibody that decreases angiogenesis, capillary permeability, and growth of tumors, including glioblastoma.39 Inhibition of VEGF signaling by a variety of knockout techniques halts the growth of experimental gliomas.39–43 In patients with gliomas, VEGF is highly overexpressed in the tumor tissue44 and the cerebrospinal fluid (CSF),45 making VEGF an attractive therapeutic target.
Phase I trials showed that bevacizumab was well tolerated and could be added to standard chemotherapy without exacerbating chemotherapy-induced toxicity.2 In a phase II trial in patients with metastatic colorectal cancer, bevacizumab added to chemotherapy (irinotecan, 5-fluorouracil, and leucovorin [IFL]) resulted in a significant improvement of overall survival, response rate, and time to disease progression.2 These findings were validated in a randomized, prospective trial that provided class I evidence of increased survival using an antiangiogenic drug (Fig. 104-4).2,3 Bevacizumab was confirmed to be an effective agent, extending progression-free survival, when used in combination with cytotoxic chemotherapy for patients with cancer of the breast46 or lung.47 The success of bevacizumab preceded FDA approval of other antiangiogenic compounds, notably sunitinib (Sutent, Pfizer, New York); sorafenib (Nexavar Onyx, South San Francisco, CA), and lenalidomide (Revlimid, Celgene Summit, NJ), for a spectrum of cancer types.32
How was bevacizumab extended to glioblastoma? The success of bevacizumab, combined with irinotecan (IFL), led paradoxically to its translation to glioblastoma, what could be termed the “irinotecan irony” or the “perseverance of patient caregivers.” Initially, bevacizumab, itself, was proscribed for use in brain tumors because of the presumed risk for intracranial hemorrhage, based on anecdotal reports.48 However, Virginia Stark-Vance, a neuro-oncologist, placed a patient (Dorothy B.) with a recurrent glioblastoma on irinotecan as “salvage therapy”: Irinotecan as a single agent has minimal efficacy.9,49–52 The patient’s husband learned of the FDA approval of bevacizumab, in combination with irinotecan, and insisted that bevacizumab be added.48 With some trepidation, the regimen of irinotecan with bevacizumab was tried on a compassionate care basis (Fig. 104-5).
Dorothy B. experienced an immediate clinical improvement that correlated with a volumetric reduction on magnetic resonance imaging (MRI). This success was repeated by clinical improvements in 26 of the next 29 patients with recurrent glioblastoma.4,48 There was no intracranial hemorrhage; however, despite a prolonged progression-free interval, many patients developed VEGF resistance and succumbed to tumor recurrence. There also appeared to be a few patients who had complete responses.48 One patient with a radiographic complete response had a necropsy after death for an unrelated comorbidity, without histologic evidence of residual glioblastoma.48 There are no data currently on whether other cytotoxic agents are superior to irinotecan because nearly the entire reported experience (see Table 104-1) is based on the bevacizumab-irinotecan combination.6,52–65
Angiotherapy: An Effective, Novel Approach to Glioma Therapy
The efficacy of the bevacizumab-irinotecan combination is readily apparent in terms of tumor response (see Fig. 104-4). We performed a meta-analysis of 605 patients with malignant gliomas from 16 reports (see Table 104-1) and found radiographic response rates of 30% to 73% (median, 50%; mean, 53%)—striking results compared with the historical controls of 9% response to cytotoxic chemotherapy for recurrent malignant gliomas.66 Wong and colleagues reported a 6-month progression-free survival (PFS) of 21% achieved with several chemotherapeutic regimens66; by contrast, the average 6-month PFS of bevacizumab-irinotecan is 50% or more (range, 25% to 78%). PFS is regarded as a key end point, used by the FDA and the North American Brain Tumor Consortium (NABTC), for the evaluation of therapy for recurrent high-grade gliomas.67 The NABTC experience of 6-month PFS for patients with recurrent malignant glioma, not taking temozolomide, is 17% for patients with anaplastic gliomas and 9% for those with glioblastoma. By contrast, the recent reports confirm that bevacizumab-irinotecan represents an unprecedented advance in the treatment of recurrent anaplastic gliomas and glioblastomas (see Table 104-1).
Whether the dramatic increase in 6-month PFS will translate to significant increases in overall survival is uncertain. Based on metabolic imaging using [18F]fluorothymidine positron-emission tomography, responders had a median survival of 10.8 months; nonresponders, only 3.4 months, suggesting a 7-month gain in the patients who had a metabolic response to bevacizumab.54 A partial response, measured by classic Macdonald criteria, also tended to correlate with overall survival,54 but metabolic scanning was more sensitive and rapid. Others found no difference in overall survival between MRI responders and nonresponders.55 Drug resistance while on bevacizumab, as with other targeted agents,68 remains a therapeutic challenge and can reduce overall survival. In non–central nervous system (CNS) cancers, such as breast cancer,46 FDA-approval was based on improvement in 6-month PFS and not the time of overall survival.
Most patients will respond to bevacizumab, clinically and radiographically (see Fig. 104-5). In certain series, radiographic response rates are as high as 70% when partial and complete responses are added. When stable disease is also taken into account, tumor control rates higher than 90% are achievable.6,48,59 Treated tumors that radiographically showed minimal, partial response (minPR) also showed improved outcome,59 similar to non-CNS cancers, treated with targeted therapy, where data is evaluated by a “waterfall plot.”69 Small volumetric reduction (<50% but >10%) can be associated with significant clinical improvements in patients with recurrent glioblastoma treated with bevacizumab.9,70
Responses to bevacizumab, or failures, are noticeable early, usually after four doses (two cycles) and the first follow-up MRI.52 Responses occur even in patients heavily pretreated with chemotherapy and irrespective of age, gender, location of the tumor, or extent of surgical resection.53,58 Performance status is correlated with survival in certain reports,58 but not in others.53 Angiotherapy is effective for patients with anaplastic glioma.55,57,65 Paradoxically, the radiographic response to bevacizumab may be more pronounced for patients with glioblastoma than anaplastic glioma, possibly because of the higher VEGF levels in glioblastomas.55,65
Taken together, the International Brain Tumor Association concluded, “Bevacizumab in combination with irinotecan is already regarded as the de facto standard treatment for recurrent or refractory glioblastoma. In addition, some neuro-oncologists in the United States, are routinely prescribing bevacizumab as monotherapy for recurrent glioblastoma.”71
Mechanisms of Efficacy
Five mechanisms account for the observed responses to anti-VEGF angiotherapy.
Angiogenic Switch
Angiogenesis is recognized as a fundamental hallmark of cancer.72 During the development of malignancy from preneoplasia, the angiogenic switch is expressed (see Fig. 104-3),73,74 —a key event in multistage carcinogenesis with therapeutic implications.75 Angiogenesis is essential in the stepwise progression of low-grade gliomas to anaplastic gliomas and glioblastoma.76 The objective of angiosuppression is to suppress the logarithmic growth associated with the onset of the angiogenic switch77 and, ultimately, to convert large vascularized tumors such as glioblastoma into tiny, innocuous, nodules, analogous to in situ cancer, that is, “cancer without disease.”78 Angiotherapy, by reducing tumor size, can theoretically enhance other modes of therapy, including chemotherapy, radiation therapy, and immunotherapy.77
Angiogenesis not only affects the growth potential of the malignant cell by affecting the microenvironment and permitting logarithmic growth—that is, affecting the “soil”—but also affects the “seed” of cancer. The transformed cancer cell undergoes oncogene activation, dependence, or “addiction.”1 For example, tumorigenicity of myc or ras oncogenes is angiogenesis dependent.79,80 Mutations relevant to glioma formation—including the PTEN, EGFR, or c-MYC genes—can act as an angiogenic switch by stabilizing hypoxia-inducible transcription factor-1α (HIF-1α) or one of its downstream targets, such as VEGF.76 Human glioblastomas contain clones that vary in their angiogenic capacity.15 Those that display the angiogenic switch grow rapidly, produce vascularized tumors in immunodeficient mice, and are rapidly fatal; by contrast, those glioblastoma cells that are nonangiogenic are slow growing and harmless to the host.15
Key steps of the angiogenic switch (Fig. 104-6) in human glioblastoma are as follows: (1) glioma cells, in response to gene mutations or hypoxia, release growth factors, such as VEGF (or basic fibroblast growth factor [bFGF]), that activate brain endothelial cells during the switch to the angiogenic phenotype; (2) growth factors, such as VEGF, bind to endothelial cell receptors and activate signal transduction pathways (Fig. 104-7), leading to endothelial cell proliferation; (3) sprouting vessels secrete urokinase and metalloproteinases and migrate toward the tumor using specific αβ-integrins; (4) glioma cells surround the host vessels by co-option and migrate along these vessels away from the main mass of the tumor to form a protected environment, or a “vascular niche”; and (5) the glioma cluster reaches a critical size, about 5 × 105 cells,77 then the glioma cells recruit their own, additional vessels, perpetuating the process.81
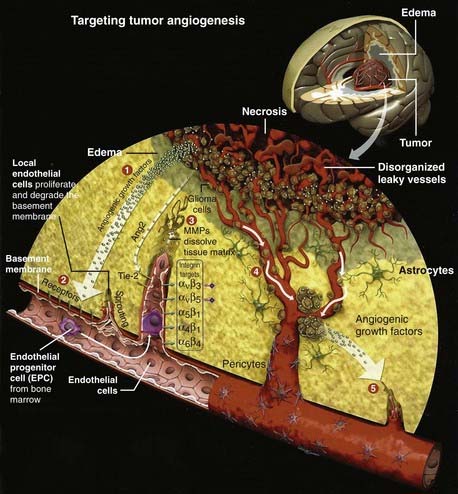
(From Batchelor T, Brem S, Sorensen AG, eds. Targeting tumor angiogenesis: antiangiogenic therapy for advanced malignant glioma. The Angiogenesis Foundation, 2008. Available at: http://www.angio.org.)
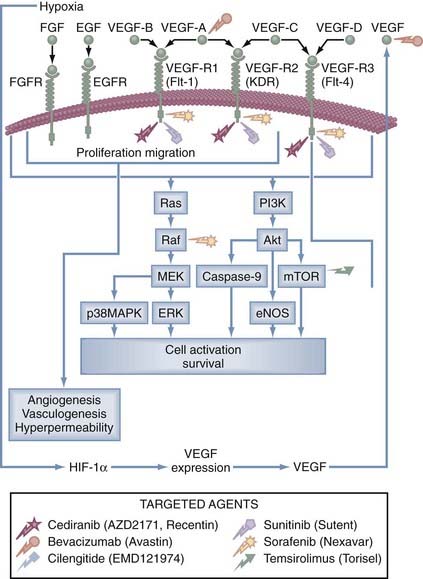
FIGURE 104-7 Signal transduction pathways activated by vascular endothelial growth factor (VEGF) and its receptors (VEGF-R1, VEGF-R2, VEGF-R3) stimulate angiogenesis and provide targets (see Table 104-2) for novel agents (bevacizumab, cediranib, cilengitide, sunitinib, sorafenib, and temsirolimus). The following are genes or oncogenes that do not have words to describe them but are used as three letter symbols: Akt, MEK, Raf, and Ras. EGF, epidermal growth factor; EGFR, epidermal growth factor receptor; eNOS, endothelial nitric oxide synthase; ERK, extracellular signal-related kinase; FGF, fibroblast growth factor; FGFR, fibroblast growth factor receptor; HIF-1α, hypoxia-inducible growth factor-1α; p38MAPK, p38 MAP kinase; mTOR, mammalian target of repamycin; PI3K, phosphatidylinositol 3-kinase.
(From Batchelor T, Brem S, Sorensen AG, eds. Targeting tumor angiogenesis: antiangiogenic therapy for advanced malignant glioma. The Angiogenesis Foundation, 2008. Available at: http://www.angio.org.)
An important stimulus for the angiogenic switch in glioblastoma is hypoxia. Within hours of exposure to hypoxia, C6 glioma cells upregulate the expression of VEGF messenger RNA and other proangiogenic peptides.82 The expression of VEGF is tightly regulated by the HIF complex, including HIF-1α and HIF-2α.83,84 A subset of tumors initiate growth by co-opting existing host vessels, which then regress, leading to an avascular tumor that depends on angiogenesis for further growth.85 After treatment with angiogenesis inhibitors, tumor cell apoptosis occurs near the endothelium.75
The endothelial cells that form the vasculature of gliomas can arise from adjacent mature blood vessels or circulating endothelial precursor cells derived from the bone marrow.19,21,85,86 Because the endothelial cells arise from nonmalignant cells, it was once thought that tumor endothelium would be genomically stable and resistant to the multidrug resistance87,88 that limits the efficacy of cytotoxic chemotherapy. Analysis of the endothelium derived from gliomas, however, indicates that these cells are genetically distinct from the normal quiescent endothelium, with mutations found that resemble those characteristic of gliomas.89–91 Dynamic time-lapse analysis of endothelial cell–glioma cell interactions shows that not only does the microvasculature provide a physical scaffold on which the glioma cells migrate but also that glioma cellular mitosis occurs preferentially at microvascular branch points, suggesting that both proliferation and migration of glioma cells are guided by the microvasculature.92 The anatomic bifurcations are not only branch points but also mitotic control points.
Numerous proangiogenic factors are implicated in glioma biology,22,23,93 but the key factors include VEGF, bFGF, CXCL12 (stromal cell–derived factor-1α [SDF-1α]), hepatocyte growth factor–scatter factor (HGF-SF), NG2, platelet-derived growth factor, and tenascin C.93,94 bFGF is overexpressed in glioblastoma94 and has an autocrine, proliferative effect because glioblastoma cells have an elevated expression of FGF receptor 1.93 The binding of VEGF or bFGF to its cognate receptors activates the existing endothelial cells.93 Binding of VEGF to its receptors on the endothelial cell typically results in signaling through the phosphatidylinositol 3-kinase (PI3K)/protein kinase B (Akt) pathway (see Fig. 104-7), whereas the bFGF receptors signal predominantly through the protein kinase Cα pathway.93 Other stimulators are angiopoietin-1, angiotropin, angiogenin, epidermal growth factor, endothelin-1, transforming growth factor-β1 (TGF-β1), granulocyte colony-stimulating factor, interleukin-1, interleukin-6, and interleukin-8.22,23,93–96
Angiogenesis is physiologically suppressed by one or more of the known endogenous inhibitors, including angiopoietin-2, angiostatin, brain angiogenesis inhibitor-1 (BAI-1), endorepellin, endostatin, interferon-α, kringle-5, pigment epithelium–derived factor, platelet factor-4, prolactin (16-kD fragment), thrombospondin, tissue inhibitors of metalloproteinase (TIMP-1, TIMP-2, and TIMP-3), troponin I, tumstatin, and thrombospondin-1.15,93,97,98
The VEGF signaling pathway is the dominant pathway in tumor angiogenesis, the “master regulator of the angiogenic cascade,”99 and can be blocked in one of three main ways1,100–119: (1) blocking production of VEGF by the tumor cell, for example, temsirolimus inhibits mTOR activation of the tumor cell (see Fig. 104-7); (2) blocking the ligand, VEGF, after it is secreted by the tumor but before it attaches to its receptor—for example, bevacizumab neutralizes circulating VEGF, and aflibercept, a soluble decoy receptor, traps VEGF100,101; and (3) using one or more of several drugs (see Table 104-2) that target one or more VEGF receptors.102,103,110,112,117,119
Normalization Hypothesis and the Normalization Window
The detailed physiologic studies of Jain and colleagues16,21,25,120 on tumor-microvascular interactions, including measurements of oxygenation, blood flow, and interstitial pressure of brain tumors, led to the normalization hypothesis (Fig. 104-8). This model provides the basis for restoring a physiologic balance of angiogenic stimulators and inhibitors. The optimal normalization window varies with each specific drug, dose intensity, duration of treatment, and duration of response.16 The normalization concept helps interpret findings in preclinical models of angiogenic inhibitors used for drug discovery.14,121–125 In Figure 104-8C, the tumor vascular bed has been exposed to an angioinhibitor, such as bevacizumab or penicillamine, thereby normalizing the vessels (compare with Fig. 104-9B), restoring blood flow, enhancing oxygenation, improving delivery of chemotherapeutic drugs, and increasing radiosensitivity.123
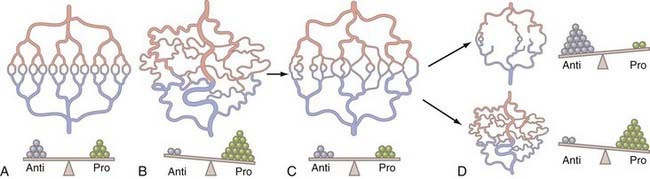
FIGURE 104-8 Diagram of the normalization hypothesis and implications for angiotherapy. A, The vascular system normally shows a physiologic branching with arteries and arterioles (light red) each being drained by a matching system of venules and veins (light blue), adequately perfusing the zone of tissue, through a well-formed capillary bed (red and blue, center); there is a balance of endogenous proangiogenic factors (green circles) and endogenous antiangiogenic molecules (gray circles). B, Disruption of the microvasculature is induced by a tumor producing angiogenic factors (e.g., vascular endothelial growth factor or basic fibroblast growth factor), the molecular balance (bottom) is tilted to angiogenesis and structurally marked by aberrant vascularization (tumor-induced angiogenesis) with hypertrophied, tortuous, dilated arterioles and venules (as visualized in Fig. 104-9A), similar to a human glioblastoma when newly diagnosed. Note that the vessels are dysfunctional with significant shunting of blood to the venous system, leading to hypoxia in the tissue core. C, The tumor vascular bed has been treated with an effective inhibitor (e.g., bevacizumab), thereby normalizing the vessels, with restoration of physiologic flow, decrease in edema, restoring oxygenation, allowing delivery of chemotherapeutic agents, and increasing radiosensitivity. There is a balance again of inhibitors and proangiogenic factors during this period, called the normalization window. Compare the pattern of blood vessels to that in A and in Fig. 104-9B. D, The therapeutic window closes; resistance emerges in two different patterns. On top, there could be an excess angiosuppression resulting in reduced blood flow and impaired access of chemotherapeutic molecules, hypoxia, and radioresistance. The angiosuppressed tumor receives nutrients from preexisting vessels (co-option) or by diffusion from cerebrospinal fluid channels (compare with Fig. 104-14M, gliomatosis). On bottom right, angioinhibition could be resisted by the secretion of additional angiogenic factors and renewed angiogenesis (compare with Fig. 104-14N, new vascular nodule).
(From Jain RK: Lessons learned from multidisciplinary translational trials on anti-angiogenic therapy of cancer. Nat Rev Cancer. 2008;8:309-316.)
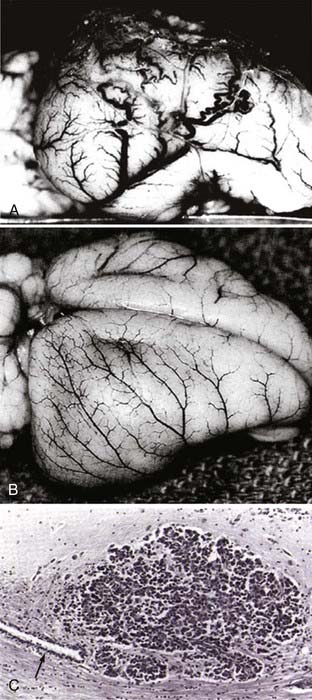
FIGURE 104-9 Brain tumor angiogenesis, anti–vascular endothelial growth factor (VEGF) normalization, and vascular co-option in an animal model. In the first initial demonstration of angiosuppression in an orthotopic brain tumor model,14 we used penicillamine, a chelator of copper, a necessary cofactor of VEGF, as well as other angiogenic peptides and cytokines (e.g., basic fibroblast growth factor, hepatocyte growth factor–scatter factor, and interleukin-1β.126 In a proof-of-principle experiment that angiosuppression could inhibit malignant growth in the brain, we implanted tumors that recapitulated the angiogenic response with numerous, dilated, serpiginous vessels that resemble the chaotic vessels of a human glioblastoma (A). By contrast, in a treated animal, in which VEGF and other factors are inactivated, the cerebral cortical vasculature is normalized, showing the normal, branching pattern (B). In tumors growing in the brain, when angiogenesis is blocked pharmacologically, the neoplastic cells adapt by attaching to preexisting blood vessels (arrow), an example of co-option and a mechanisms of VEGF escape (C). The normalization hypothesis explains the efficacy of antiangiogenics, such as bevacizumab, when given in combination with cytotoxic chemotherapy: improved perfusion and oxygenation enhance drug delivery and chemosensitivity of cytotoxic drugs.
(From Brem S, Zagzag D, Tsanaclis A, et al. Inhibition of angiogenesis and tumor growth in the brain: suppression of endothelial cell turnover by penicillamine and depletion of copper, an angiogenic cofactor. Am J Pathol. 1990;137:1121-1142.)
Patients with recurrent glioblastoma who were given the pan-VEGF receptor tyrosine kinase inhibitor, cediranib, showed normalization of perfusion and permeability within 24 hours as revealed by dynamic susceptibility contrast (DSC) MRI.25 The normalization window varies from patient to patient; in some patients, normalization persists for months.25,124 Ultimately, the normalization window disappears, although the exact timing is not always apparent because of the time interval between scheduled MRI tests.124 In preliminary studies of patients treated with bevacizumab, there is histologic evidence of normalization because the vessels within the treated tumors are thin walled, evenly distributed, and have a decrease in microvascular density.126 Exploiting vessel normalization to improve chemotherapy might be best in situations involving tumors such as glioblastomas,127 confined to a single organ, or when using localized radiation therapy.
Vascular Niche and Glioma Progenitor and Stem Cells
Another target for bevacizumab and angiotherapy is the perivascular niche where glioma progenitor cells resembling stem cells (SCLGCs) reside (Fig. 104-10).17,128 The stem cell hypothesis proposes that cancers arise from a small fraction of cancer cells that constitute a reservoir of self-sustaining cells that maintain the tumor.17 The SCLGCs share characteristics of neural stem cells.128,129
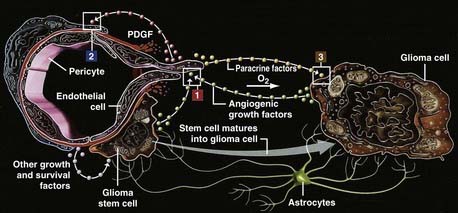
FIGURE 104-10 The vascular niche and cellular interactions in brain tumor angiogenesis. During tumor angiogenesis (1), endothelial cells in turn recruit pericytes (2) through platelet-derived growth factor, which stabilizes blood vessels. Stem cell–like glioma cells (SCLGCs) associate directly with endothelial cells (3), forming a perivascular stem cell niche,17 a renewable source of glioma cells and a sanctuary to evade chemotherapy. The SCLGCs are selectively vulnerable to bevacizumab.129
(From Batchelor T, Brem S, Sorensen AG, eds: Targeting tumor angiogenesis: antiangiogenic therapy for advanced malignant glioma. The Angiogenesis Foundation, 2008. Available at: http://www.angio.org.)
The intersection of the stem cell hypothesis and angiogenesis research has been one of the most exciting new avenues in neuro-oncology, offering new insights into the pathogenesis and identification of therapeutic targets.17,128–133 The availability of trophic factors from endothelial cells, such as brain-derived neurotrophin (BDNF), chondroitin proteoglycans, tenascin C, VEGF-C, bone morphogenetic proteins, and pigment epithelium–derived growth factor, make the perivascular niche a microenvironment that supports the growth of the glioma.17 Expanding the number of blood vessels results in an increase in the fraction of SCLGCs.131,133 By contrast, depletion of blood vessels from gliomas ablates self-renewing cells from tumors and arrests tumor growth.131
When compared with other glioma cells, the SCLGCs consistently secrete elevated levels of VEGF, further amplified by hypoxia.129 The injection of SCLGCs, modified to overexpress VEGF, leads to the massive growth of the vascular-rich glioblastomas, tumor-associated hemorrhages, and a high morbidity, suggesting that VEGF promotes tumorigenesis through angiogenesis.132 The proangiogenic effects of glioma SCLGCs on endothelial cells are specifically abolished by bevacizumab. Furthermore, bevacizumab suppresses growth of xenografts derived from SCLGCs but shows limited efficacy against xenografts derived from a matched non-SCLGC population.129
The disruption of such a vascular niche could result in the loss of “stem” qualities, such as intrinsic drug and radiation resistance,130 providing another mechanism by which angiosuppression would be a chemosensitizer131 or radiosensitizer. In a rat glioma model, the combination of targeted antiangiogenic therapy and cytotoxic chemotherapy significantly reduced the fraction of tumor sphere–forming units (a measure of stem cell activity), whereas neither modality tested alone was effective.131 These findings explain why bevacizumab, in combination with chemotherapy, could be more effective than antiangiogenic monotherapy for many types of human cancers; also, it explains, in part, why it may not be necessary to have a significant reduction in tumor volume to achieve an increase in survival, if the stem cell vascular niche is selectively vulnerable.131 If indeed the stem-like glioma cells are the cellular target of bevacizumab, the existence of angiogenesis-independent but invasive clones of glioma cells134 can explain the shrinkage of vascularized tumors and the emergence of highly invasive glioma cells.135
Enhanced Immune Surveillance and Tumor Immunity
An important mechanism, often overlooked, is the potentially beneficial effect of anti-VEGF angiotherapy on the immune response to glioblastoma. Cell-mediated immunity is a key host response to human brain tumors136,137; however, the normal antitumor response is insufficient to eradicate malignant gliomas, which could be achieved if sufficient effector cells reached the tumor bed.138 Immune escape is a critical gateway to malignancy that affects tumor dormancy, progression, invasion, and ultimately, clinical outcomes.139 The connections between signaling pathways that control immune tolerance and those controlling proliferation, invasion, and angiogenesis remain largely unexplored, offering a fertile area for scientific investigation.
The immune response to brain tumors is normally suppressed, mediated by cytokines such as TGF-β, STAT3, and a profound suppression of TH4 lymphocytes.137,140 Standard therapies, such as corticosteroids, radiation therapy, and chemotherapy further suppress the immune response, lowering the CD4 counts.141 Normally, the endothelium-astrocyte complex abets immune escape by sequestration of antigens; VEGF blockade, however, normalizes vascular permeability and enhances perfusion, thereby exposing the antigens to blood-borne immune effector cells.142
VEGF also contributes to immune escape of tumors by blocking dendritic cell (DC) differentiation.124,143–147 An antibody to VEGF, combined with DC-based immunotherapy, results in a prolonged and enhanced antitumor effect.144 The inhibitory function of VEGF on DC function is most likely mediated by the VEGF receptor, Flt-1.145,146 Bevacizumab, given to cancer patients, has the beneficial effects of increasing the DC population of peripheral blood, enhancing the allostimulatory capacity of DC and T-cell proliferation against recall antigens, and decreasing the accumulation of immature myeloid cell progenitor cells.147 Because of the promise of current dendritic cell vaccines for patients with glioblastoma,140,148 combining immunotherapy with anti-VEGF angiotherapy has merit.
Reduction of Vasogenic Edema, Radioprotection, and Radiosensitization
A major cause of morbidity and mortality from brain tumors is the peritumoral, vasogenic edema that can cause mass effect and midline shift and is responsible for much of the morbidity and mortality of brain tumors.149 The discovery of high-dose corticosteroids, nearly 50 years ago, has been hailed as a revolution in brain tumor management and one of the greatest contributions in neurosurgery.150 Although corticosteroids truly are invaluable to control peritumoral edema, the long-term toxicities are problematic and include Cushing’s syndrome, myopathy, immunosuppression with increased risk for infections such as Pneumocystis species pneumonitis, hyperglycemia and insulin resistance, osteoporosis and pathologic fractures, capillary fragility, and bowel perforation.151,152 In addition, corticosteroids counteract in vitro the effect of temozolomide153 and in vivo increase the expression and activity of multidrug resistance transporter proteins, thereby adding to the general resistance of the blood-brain barrier to cytotoxic chemotherapy.154 Consequently, there has been a search for less toxic alternatives to corticosteroids that could be used for long-term control of vasogenic edema. For example, corticotropin-releasing factor is currently being tested in a phase III trial.21 Because VEGF is a potent inducer of hyperpermeability,21,38 anti-VEGF therapy could normalize the blood-brain barrier, alleviate peritumoral, vasogenic edema, and lessen the need for corticosteroids.21,152
In experimental systems, VEGF induces fenestrations and other structural abnormalities that comprise the anatomic substrate for vasogenic edema.155 Elevated levels of VEGF or messenger RNA for VEGF156 are found in tumors that have significant amounts of vasogenic edema, such as meningiomas,157,158 cerebral metastases,159 and malignant gliomas.160 In animal models, bevacizumab produces a rapid decrease in peritumoral edema, detected by dynamic MRI,161 and is more effective than dexamethasone.162
The reduced requirement for dexamethasone in patients treated with bevacizumab or other anti-VEGF agents (cediranib)25 has been a consistent finding in patients with recurrent glioblastoma, even in the presence of chemotherapy (irinotecan). Most patients treated with bevacizumab had at least a 50% reduction in corticosteroid dose.5,52,163 Vascular normalization efficiently decreases vasogenic edema. The reduction in vasogenic edema is apparent on fluid-attenuated inversion recovery (FLAIR) MRI after one or two doses of bevacizumab8,163 and after the first dose of cediranib21,25 when sophisticated neuroimaging techniques are used (Fig. 104-11). The steroid-sparing effect of cediranib persists until the drug is discontinued because of tumor progression.25
The clinical toxicity of radiation therapy of brain tumors can be radiation-induced necrosis, with peritumoral vasogenic edema. Heretofore, the mainstay of therapy has been corticosteroids, with surgical decompression used if the patient became steroid dependent or steroid toxic, or if there were symptoms and mass effect despite corticosteroids. Treatment with hyperbaric oxygen, vitamin E, and other antioxidants yield only modest benefits. The molecular response of malignant cells to ionizing radiation is the secretion of VEGF and metalloproteinases, which then promote angiogenesis and tumor invasion.164,165 Bevacizumab has been discovered recently to provide a consistent benefit in patients with radiation-induced necrosis,26,166,167 with a striking 50% to 60% reduction in vasogenic edema (detected by FLAIR images) and a 50% to 80% reduction in the size of the necrotic area (detected by contrast enhancement) (Fig. 104-12), leading to a substantial reduction in the daily dexamethasone requirement and neurocognitive improvement.166,167 The optimal duration for treatment of radiation necrosis with bevacizumab is unknown, but it is recommended to be discontinued after 6 months, followed by surveillance MRI every 3 months.167
Angiotherapy, by normalizing the vasculature, can serve not only as a radioprotectant but also as a radiosensitizer. Because of numerous preclinical studies that show an enhanced, synergistic response to radiation therapy when angiogenesis, particularly VEGF signaling, is inhibited,101,168–172 Gutin and associates evaluated the safety and efficacy of bevacizumab with hypofractionated stereotactic irradiation in patients with recurrent malignant glioma.173 These patients had already received standard radiation therapy (about 60 Gy) and were given an additional 30 Gy in five fractions while receiving concurrent bevacizumab. The additional irradiation was well tolerated without any instances of radiation-induced necrosis, possibly a protective anti-VEGF effect of bevacizumab. The 6-month PFS was 65%, the median overall survival was 12.5 months, and the 1-year survival was 54%. Bevacizumab combined with hypofractionated stereotactic irradiation proved safe and well tolerated with evidence of activity.173
Further extensions of combining anti-VEGF angiotherapy with radiation therapy and radiosurgery are anticipated. For example, the response to a single, high dose of radiation (10 Gy) in a laboratory model is an upregulation of VEGF by the normal brain as a response to radiation injury.174 In patients with metastatic cancer to the brain, stereotactic radiosurgery effectively achieves local control, but the peritumoral edema adversely affects quality of life.175 For this large patient population, we propose that the addition of bevacizumab or another antiangiogenic compound, such as an agent that targets integrins176 or cyclooxygenase-2,177 would increase the efficacy and safety of radiosurgery and potentially enable dose reduction at the isocenter or increase tumor responses, while improving quality of life.
Because of the radiosensitizing properties of anti-VEGF therapy, as well as to control vascular leakage—an independent predictor of the time to recurrence of gliomas178—a pivotal phase III trial combining standard, external-beam radiation therapy (60 Gy) with temozolomide plus bevacizumab is being organized for patients with newly diagnosed glioblastoma. A single-institution feasibility study reports promising results with a 1-year PFS of 59.3% and a 1-year overall survival rate of 86.7%.179 Temozolomide is effective in the treatment of newly diagnosed glioblastoma,152 in part because it acts as a radiosensitizer.180 Temozolomide also suppresses angiogenic pathways; in animal models, bevacizumab increases the antitumor efficacy of temozolomide.181 Increasingly, targeted agents will be used in the standard upfront setting of newly diagnosed cancers. An example is the success of cetuximab, an epidermal growth factor receptor (EGFR)-targeted agent, when combined with radiotherapy for carcinoma of the head and neck.182 Cetuximab blocks the production of VEGF and is an indirect inhibitor of angiogenesis.183
Clinical Experience with the Use of Bevacizumab
Bevacizumab is a novel agent for brain tumor therapy, with a preliminary, yet extensive, clinical experience listed in Table 104-1. Practical guidelines and caveats are summarized in Table 104-3. Bevacizumab is generally given at a dosage of 10 mg/kg every 14 days on a 28-day cycle; two infusions constitute one cycle. The maximal tolerable dose is about 15 to 20 mg/kg; the optimal biologic dose is unknown. Favorable results are reported with dosages as low as 4 to 5 mg/kg.52 Bevacizumab is diluted in 100 mL of 0.9% sodium chloride; the intravenous infusion is given slowly, initially, over 90 minutes, with the second dose given over 60 minutes, and if tolerated well, subsequent treatments given over 30 minutes.184 The lower (5 mg/kg) dose can be given safely as a 10 minute infusion.185
TABLE 104-3 Pearls and Caveats in the Use of Bevacizumab for the Management of Malignant Glioma
Bevacizumab, for recurrent glioblastoma, is often given with irinotecan (dosage of irinotecan, 340 mg/m2 or 125 mg/m2, depending on the concurrent use of antiepileptic drugs the higher dosage for patients taking anticonvulsants that activate hepatic cytochromes). In the initial study of bevacizumab, given in combination with irinotecan (the bevacizumab plus IFL arm; see Fig. 104-4), the irinotecan active metabolite, SN-38, was 33% higher in patients receiving bevacizumab plus chemotherapy, compared with chemotherapy alone, perhaps explaining the higher incidence of grades 3 and 4 diarrhea and neutropenia in the combination arm.3 Higher levels of SN-38 could contribute to the angiosuppressive activity because SN-38 is antiangiogenic.186 Standard cytotoxic chemotherapy, including temozolomide, when given in a low-dose, continuous (metronomic) fashion is also angiosuppressive.19,187–189 Metronomic chemotherapy acts directly on the mature endothelial cells within the tumor neovasculature and inhibits the mobilization of circulating, endothelial progenitor cells.19,190
What toxicities are linked to anti-VEGF therapy in general (e.g., cediranib, sunitinib, sorafenib) and bevacizumab specifically?191 Mild epistaxis (grade 1) is the most common form of bleeding linked to bevacizumab and usually resolves without medical intervention.191 The risk for bleeding with bevacizumab is not substantially altered by the concomitant use of low-dose aspirin or warfarin.192 A major concern, especially for neurosurgeons, is the risk for spontaneous intracranial hemorrhage during bevacizumab therapy or in the perioperative period. Highly vascular tumors, such as glioblastoma or metastatic melanoma, sporadically present with spontaneous intracerebral hemorrhage.193,194 The risk for bevacizumab-induced intracranial hemorrhage is minimal in patients with glioblastomas,9 similar to the natural history of glioblastoma. Indeed, patients with glioblastoma who develop venous thromboembolism can safely undergo anticoagulation while receiving treatment with bevacizumab.195 An extensive meta-analysis examining the safety of anti-VEGF therapy in more than 10,000 patients found the rate of intracranial bleeding to be negligible, even in the presence of CNS metastases.196
Hypertension is one of the most frequently observed side effects of anti-VEGF therapy and can usually be managed with oral antihypertensive agents without dose reduction of the angiosuppressive drug.191 Because hypertension is the consequence of interference with VEGF signaling, its manifestation can be viewed as a surrogate biomarker of VEGF efficacy.197 VEGF normally increases the synthesis of nitrous oxide (NO), a vasodilator, but VEGF blockade downregulates endothelial NO synthase, leading to increased peripheral resistance and hypertension.191 In patients with systemic cancer, the overall rate of bevacizumab-induced hypertension is about 32% with severe (mostly grade 3, rarely grade 4) hypertension occurring in about 15% of patients.3,191,198 In a recent National Institutes of Health (NIH) study, only 8% of patients with recurrent glioblastoma treated with bevacizumab had grade 2 hypertension, 4% had grade 3 hypertension, and there were no patients with grade 4 hypertension; in all patients, blood pressure could be controlled medically, without discontinuing bevacizumab.9 Calcium channel blockers (e.g., nifedipine) rapidly reverse the hypertensive effect, without altering the antitumor benefit of anti-VEGF drugs such as cediranib.199 Hypertension emerges over weeks and months, so blood pressure should be monitored every 2 weeks before administration of bevacizumab,184,200 or more frequently in patients with preexisting hypertension, or when starting antihypertensive medication.200 The Seventh Report of the Joint National Committee on Prevention, Detection, Evaluation, and Treatment of High Blood Pressure (JNC-7) hypertension guidelines serve as the cornerstone of medical management.201
The key role of VEGF in normal renal physiology is reflected in the common (about 33%) occurrence of proteinuria with anti-VEGF drugs.191,202 In general, proteinuria is asymptomatic and harmless, a laboratory abnormality that can be viewed as a biomarker,197 but it still bears monitoring. At the start of anti-VEGF therapy, a baseline urinalysis should be evaluated for protein, and regularly during therapy: if the urine dipstick is 2+, a 24-hour urine collection should be performed, and bevacizumab should held if urine protein is greater than 2 g.200
The role of bevacizumab in the pathogenesis of arterial or venous thromboembolism (VTE), one of the leading causes of morbidity and mortality in cancer patients, is unclear.192,203 Patients with malignant gliomas are already are at risk for thromboembolism, estimated between 7% and 30%, related to multiple risk factors such as age, proximity to surgery, dehydration, immobilization, paralysis, and comorbidities.204–207 In the 605 patients summarized in Table 104-1, discontinuation of bevacizumab because of VTE was rare. In one report, 5 of 25 patients (20%) developed deep venous thrombosis, but at a frequency expected in view of the normally high incidence of VTE in patients with malignant glioma.56 In a large meta-analysis of VTE in cancer patients (n = 7956), bevacizumab was significantly associated with an increased risk for developing VTE,203 depending on the tumor type (colorectal cancer, 19%; non–small cell lung cancer, 15%; breast cancer, 7%; and renal cancer, 3%). Patients who develop VTE during bevacizumab therapy should be managed according to the best standard of care, including anticoagulation (warfarin can be safely administered).192 Scappaticci and coworkers192 reported an increased risk for arterial thromboembolism, especially in patients with a prior history of an arterial thromboembolism and in patients older than 65 years, but no increase in risk for VTE in patients with metastatic carcinoma treated with chemotherapy and bevacizumab, compared with chemotherapy alone.
Because of the key role of VEGF in wound healing208,209 and the prolonged half-life of bevacizumab of 20 days (range, 11 to 50 days), bevacizumab should not be started until at least 28 days after major surgery, or until the wound is fully healed.200 Of patients with colorectal cancer, those who had received bevacizumab when surgery was performed within 60 days of the last dose experienced a complication rate of 13%, compared with 3.4% of those patients treated with chemotherapy alone.191 Others reported no significant increase in postoperative complications in patients receiving preoperative bevacizumab 58 days (range, 31 to 117 days) before hepatic surgery for metastatic colorectal cancer.210 Despite a delay of 4 to 6 weeks after surgery and visual inspection confirming wound closure, dehiscence developed in four cases after craniotomy for recurrent glioblastoma, requiring discontinuation of bevacizumab and reoperation, followed by rapid recurrence of the tumor and a poor overall outcome.211 Persistent CSF leak in patients treated with bevacizumab after carmustine (Gliadel) wafer placement is reported,212 reinforcing the need for watertight dural and meticulous wound closures. Wound healing might be less problematic using antiangiogenics with a shorter half-life than bevacizumab, such as sorafenib or sunitinib.191
The incidence of bowel perforation among 244 patients with malignant glioma treated with bevacizumab, in combination with corticosteroids and chemotherapy, is 2.5%.213 Two of the patients (<1%) succumbed to this complication (one patient refused salvage surgery), the others recovered, and a few required sigmoid colectomy or colostomy. Because perforation of the colon can be life-threatening, caregivers need to have a high index of suspicion if a patient develops abdominal pain or other gastrointestinal symptoms.213 The incidence of gastrointestinal perforation appears higher in patients with abdominal tumors (e.g., metastatic colorectal cancer) taking bevacizumab than in patients with malignancy at other sites.213 In patients with a variety of non-CNS cancers who are not taking corticosteroids, the incidence of bowel perforation is less than 2%.3,214 Most (79%) of these patients can be managed medically without surgical repair.214 The higher rate of bowel perforation reported for patients with intracranial gliomas might be related to the concomitant use of corticosteroids, which by itself is associated with a risk for gastrointestinal perforation as high as 2.7%,215 especially at higher dosages (dexamethasone, 16 mg/day) combined with chemotherapy. Because anti-VEGF therapy, by controlling vasogenic edema, can reduce the need for corticosteroids, it seems advisable to wean patients off steroids rapidly to minimize the risk for bowel perforation.
A rare (<0.1%) serious complication of bevacizumab is reversible posterior leukoencephalopathy syndrome (RPLS) marked by loss of vision due to vasogenic edema in the occipital lobes.216,217 The pathogenesis of RPLS is believed to be a drop in circulating VEGF leading to endothelial dysfunction. The reason for its rare occurrence and specific location in the posterior cerebral circulation is unknown.135 Optic neuropathy is a rare cause of visual loss in patients with malignant glioma treated with bevacizumab.218 Other toxicities that are “class effects” of antiangiogenics include teratogenicity and infertility because angiogenesis is essential for follicular and embryonic development. Hypothyroidism, diarrhea, fatigue, and neutropenia are also reported.184,200
Because VEGF has a dual function in both angiogenesis and neurogenesis,219–222 as well as neuroprotection223 and normal behavior,224 there is the possibility that anti-VEGF therapy could have off-target effects and adversely affect neurocognition, mental well-being, or quality of life. The self-renewal, differentiation, and function of neural stem cells require, in part, trophic factors such as VEGF and BDNF from endothelial cells in the perivascular niche.222 Most clinical reports, however, suggest these theoretical concerns of neurobehavioral toxicity are offset by the immediate and substantial reduction in vasogenic edema, mass effect, and tumor size, thereby improving neurological function, including neurocognition. Prospective, quantitative studies of neurocognition and quality of life in patients with brain tumors treated with anti-VEGF therapy are needed. For metastatic renal carcinoma, there are objective data that angiotherapy improves quality of life compared with standard treatment with cytokines (interferon-α).225
Another concern is the potential for rapid vascular regrowth, increased vasogenic edema, or rebound after discontinuing or reversing VEGF inhibition.135,226 In the cediranib trial, after a 14-day drug holiday, tumor enhancement volume and permeability increased substantially.25 The reversibility of vascular normalization, once antiangiogenic drugs are discontinued, suggests that rebound cerebral edema could occur, a phenomenon noted in the eye with rebound macular edema following cessation of bevacizumab.227 Thus, bevacizumab can be continued indefinitely as tolerated and deemed effective, unless there is clear evidence of drug toxicity or tumor progression.
One of the most pressing and practical dilemmas for patients is the high cost of bevacizumab and other targeted agents, potentially in excess of $100,000 per year.228 The efficacy of these agents makes the antiangiogenics cost-effective229 but also a focal point in the national debate of health care and cost-containment. An economic analysis, using the yardstick of quality-adjusted life-years (QALYs), found targeted agents such as bevacizumab and cetuximab at variance with the European “willingness-to-pay” thresholds,230,231 requiring further research concerning the impact of these novel therapies on health-related quality of life.231,232 The QALY analysis uses overall survival, rather than PFS, as a yardstick.232 Although bevacizumab has remarkable benefits that could “change oncology forever,”233 tumor shrinkage and an increase in 6-month PFS do not necessarily translate into major improvements in overall survival.234 Fortunately, Medicare recently expanded coverage of targeted drugs, including bevacizumab, for patients with malignant brain tumors, based on the many reports of efficacy.235
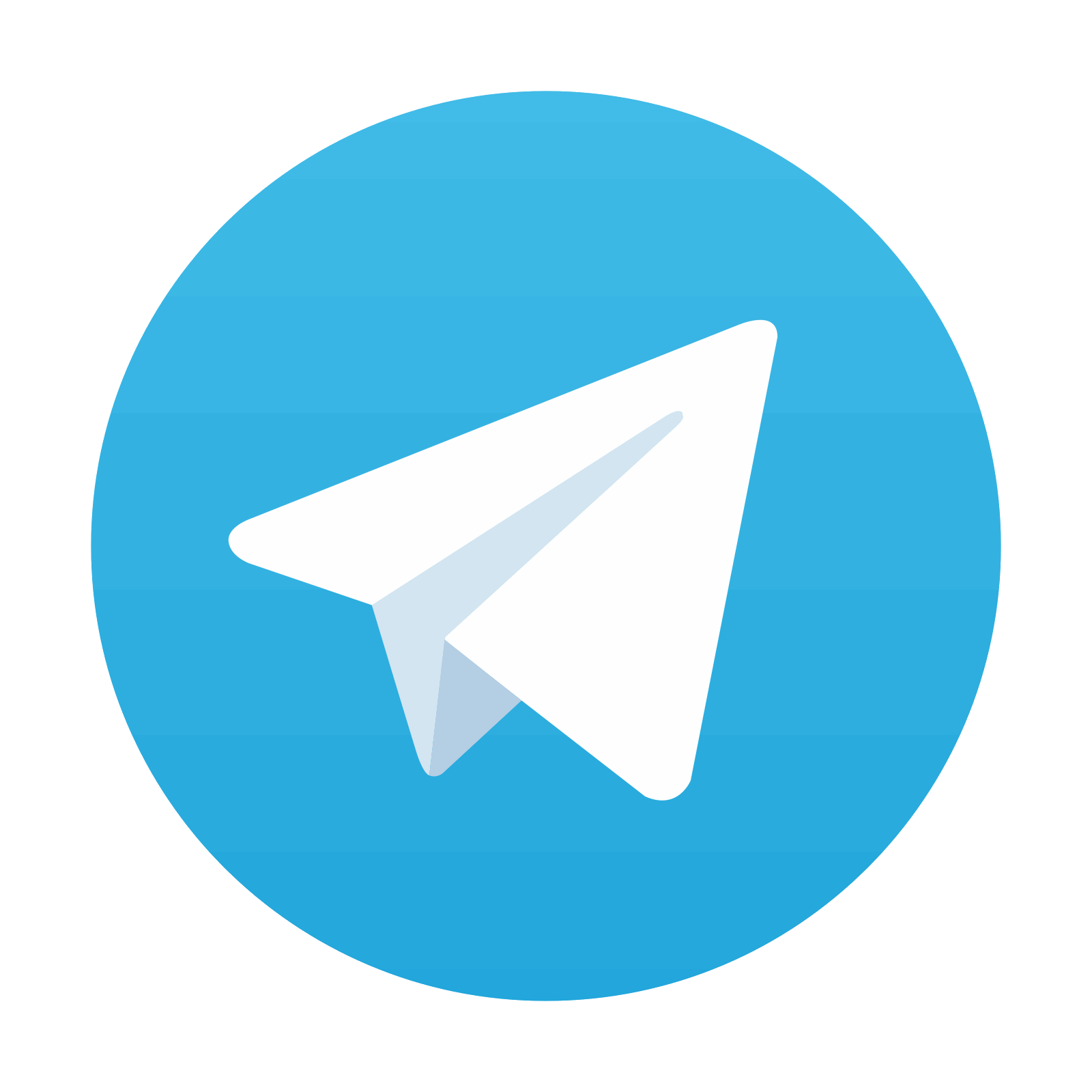
Stay updated, free articles. Join our Telegram channel

Full access? Get Clinical Tree
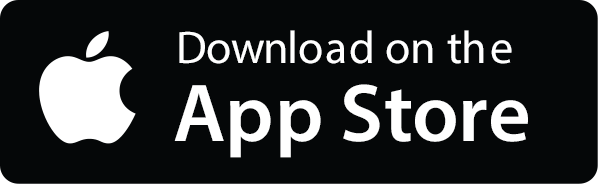
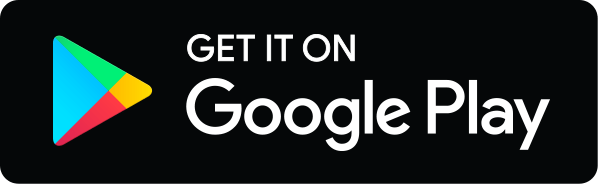