8 Artifacts and Technical Factors
Understanding and recognizing artifacts and technical factors play a central role in every nerve conduction and electromyography (EMG) study (Box 8–1). The value of the information gained during an electrodiagnostic (EDX) study relies on two important and complementary processes: (1) collecting the data correctly and (2) interpreting the data correctly. If the collected data are not technically accurate, then correct interpretation of the data can never occur, either at the time of the study or later by the referring physician.
Box 8–1
Important Technical Factors Influencing Nerve Conduction Studies and Electromyography
Non-physiologic Factors
Electrode impedance mismatch and 60 Hz interference
Cathode position: reversing stimulator polarity
Co-stimulation of adjacent nerves
Electrode placement for motor studies
Antidromic versus orthodromic recording
Distance between recording electrodes and nerve
Distance between active and reference recording electrodes
Limb position and distance measurements
Limb position and waveform morphology
Physiologic Factors
Temperature
Temperature is the most important of all the physiologic factors. It affects nearly every parameter measured in a nerve conduction study, including conduction velocity, distal latency, and waveform morphology. Temperature also can affect motor unit action potential (MUAP) morphology during the needle EMG examination. Physiologically, cooler temperatures result in delayed inactivation of sodium channels and subsequently prolong the time of depolarization (see Chapter 2). For myelinated fibers, conduction velocity is primarily determined by the time delay of depolarization that occurs at the nodes of Ranvier. Hence, prolonged depolarization times result in slowed conduction velocities for the nerve being studied. Conduction velocity slows in a fairly linear manner within the normal physiologic range of limb temperature (approximately 21–34°C). For motor and sensory conduction velocities, conduction velocity slows between 1.5 and 2.5 m/s for every 1°C drop in temperature, and distal latency prolongs by approximately 0.2 ms per degree.
In addition, longer channel opening time results in a larger influx of sodium. Subsequently, each nerve fiber depolarization is larger and longer. For both compound muscle action potentials (CMAPs) and sensory nerve action potentials (SNAPs), cooling results in a higher amplitude and longer duration as a consequence of larger and longer individual muscle and sensory fiber action potentials, respectively (Figure 8–1). This effect is more pronounced in sensory fibers, because the duration of individual sensory nerve fiber action potentials normally is shorter than that of individual muscle fibers. The normal process of phase cancellation is more prominent when individual fiber action potential durations are shorter (see Chapter 3). Thus, when cooling occurs, individual sensory nerve fiber action potentials prolong, resulting in less phase cancellation and a higher compound nerve action potential. Accordingly, any sensory study that yields a high-amplitude, long-duration potential along with a slow conduction velocity should alert the electromyographer to a possible cooling effect.
There are several ways to reduce the influence of cooling on the EDX study. First, the electromyographer must recognize the importance of temperature in every nerve conduction and EMG study (Box 8–2). Distal limb temperatures should be routinely recorded and monitored in all patients and ideally maintained between 32 and 34°C. A normal temperature at the beginning of a study does not ensure that the limb will not cool down as the study progresses; in fact, it often does cool down.
Box 8–2
Temperature and Nerve Conduction Studies and Electromyography
Maintenance of Temperature
Measure distal limb temperature in all patients
Maintain temperature at 32–34°C with heating lamp, warm packs, or hydrocollator
Remember there may be a delay when heating between when the skin and the underlying nerve reach the desired temperature
If limb is profoundly cool (>10°C cooler than desired), immerse the limb in warm water and then maintain temperature with a heating lamp
If limb cannot be warmed, use conversion factors of 1.5–2.5 m/s/°C for conduction velocity and 0.2 m/s/°C for distal latency
Limbs can be heated with heating lamps, warming packs or hydrocollators. The ideal way to warm and maintain proper limb temperature is to use a heating lamp device that has a feedback control mechanism from a temperature sensor placed on the distal hand or foot. Unfortunately, these devices are now very difficult to obtain because most manufacturers have stopped producing them due to concerns over litigation; there were incidents wherein patients were burned when they grabbed the heating element and did not realize it was a heating device. It is important to be aware, however, that regardless of the method used to warm the limb, there may be a significant delay between the time that the skin reaches the desired temperature and when the underlying nerve and muscle warm up. When a limb is warmed, skin temperature usually reaches the desired temperature several minutes before the underlying nerve and muscle do so. For limbs that are profoundly cool, it may require 20 to 40 minutes for the underlying nerve temperature to equilibrate (Figure 8–2). If this fact is not recognized, the conduction velocity may increase through the EDX study as more time passes and the nerve warms up, despite a constant skin temperature. Nerves studied earlier in the examination will conduct more slowly than those studied later in the examination, after the underlying nerve has warmed up. This can result in confusing and difficult to interpret results.
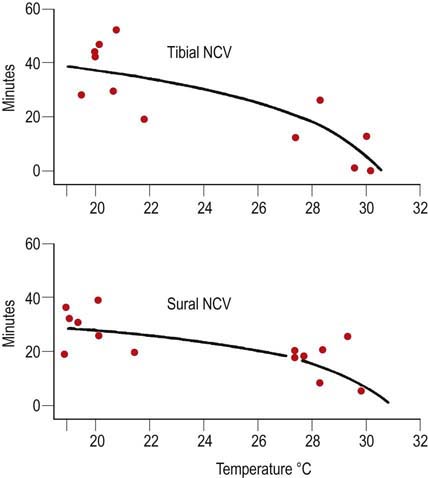
FIGURE 8–2 Warming time and conduction velocity.
(From Franssen, H., Wieneke, G.H., 1994. Nerve conduction and temperature: necessary warming time. Muscle Nerve 17, 336–344. Reprinted by permission of Wiley.)
Age
Age also affects many parameters on the needle EMG study. The most prominent effect is on MUAP duration. It is well known that as an individual ages, MUAP duration increases. In the years from birth through childhood, the duration increases due to the physiologic increase of muscle fiber and motor unit size as an individual grows. In later life, the normal aging process results in a slow dropout of motor units. Some normal reinnervation occurs to compensate, which results in slight prolongation of motor unit duration as the individual ages. For these reasons, it is important to compare MUAP durations on needle EMG with normal values based on age (see Chapter 15).
Height
The effect of height is especially relevant to the interpretation of late responses (F responses and H reflexes). The circuitry of these responses extends twice the length of the limb for the F response and twice the length of the proximal lower limb for the H reflex. Normal values of absolute latency for these potentials must be based on limb length or height (see Chapter 4). Failure to do so will result in erroneously labeling of taller individuals as having “abnormal” late responses. In some situations, however, the effect of height is not relevant, as when latencies are compared between a symptomatic and a contralateral asymptomatic limb.
Nonphysiologic Factors
Electrode Impedance and Noise
Electrical noise is present in every EDX laboratory. The most common cause of electrical noise is 60 Hz interference generated by other electrical devices (e.g., lights, fans, heaters, computers). Outside of the EDX laboratory, particularly in the intensive care unit, there may be many other sources of electrical noise such as ventilators, monitors, and other electrical devices. This noise can create a host of problems, especially when recording very small potentials such as SNAPs or fibrillation potentials (Figure 8–3). However, the examiner usually can reduce electrical noise to an acceptable level by paying close attention to technical details.
All signals recorded during the nerve conduction study and needle EMG are the result of differential amplification (Figure 8–4). With differential amplification, the difference between the signals at the active (G1) and reference (G2) electrodes is amplified and then displayed. Thus, if the same electrical noise is present at both the active and reference electrodes, it will be subtracted out, and only the signal of interest will be amplified (this is known as common mode rejection).
The best way to eliminate 60 Hz interference is to ensure that each electrode appears identical to the amplifier (Box 8–3). This can be accomplished by several steps. First, ensure that the electrodes are intact, without any frayed or broken connections. Next, the skin preparation should be thorough, using either alcohol or acetone to remove dirt and oil. Conducting electrode jelly is then applied to the electrode before it is attached to the skin. The recording electrodes should be held firmly against the skin with tape or a Velcro band. Finally, the closer the electrodes are to each other, the more likely any associated electrical noise will appear identical to a differential amplifier.
Box 8–3
Methods to Reduce Electrode Impedance Mismatch and 60 Hz Interference
Active and reference recording electrodes should be the same type.
Ensure all contacts are intact without any frayed or broken connections.
Clean all dirt and oil from the skin using alcohol or acetone.
Apply conducting electrode jelly between the skin and electrodes.
Secure electrodes firmly to the skin with tape or Velcro straps.
Place ground between stimulator and recording electrodes.
Filters
By allowing the signal to pass through a certain “pass band,” some unwanted electrical noise can be excluded. The pass band varies for different EDX studies. For motor conduction studies, the low- and high-frequency filters typically are set at 10 and 10 kHz, respectively. For sensory conduction studies, the low- and high-frequency filters typically are set at 20 and 2 kHz, respectively. Note that the high-frequency filter is set lower for sensory than for motor nerve conduction studies. This is done in order to reduce high-frequency noise, which more easily interferes with the recording of sensory nerve action potentials, which contain higher-frequency components compared with motor potentials (Figure 8–5).
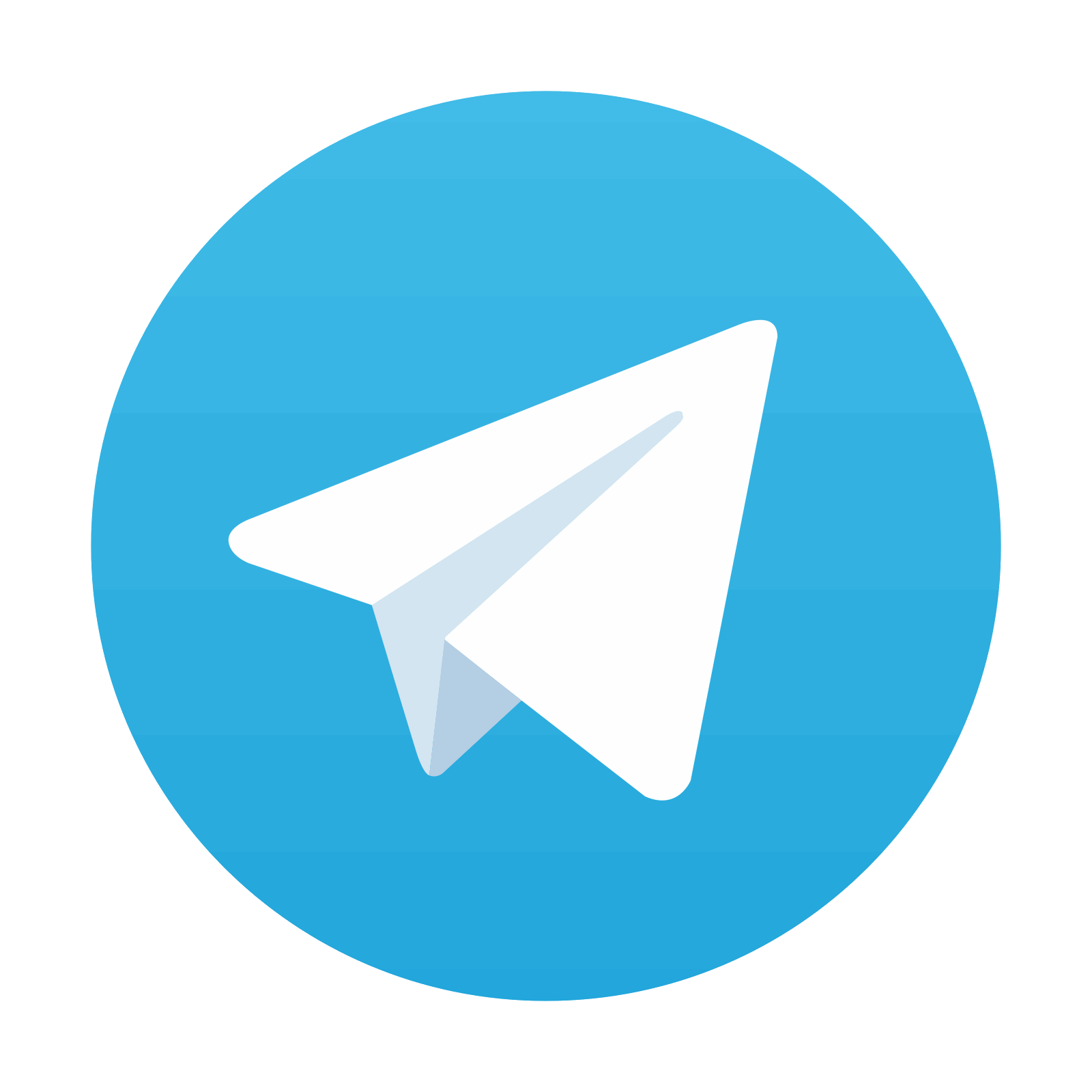
Stay updated, free articles. Join our Telegram channel

Full access? Get Clinical Tree
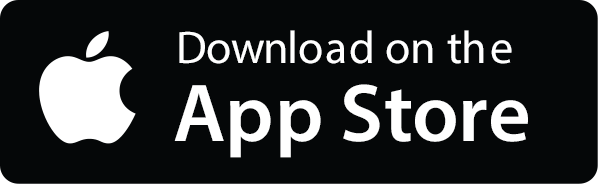
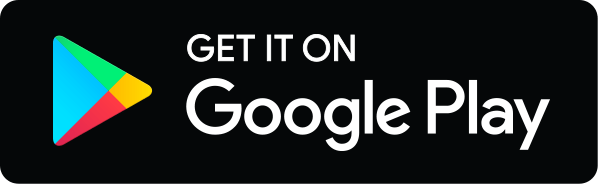