Astrocytes in the Mammalian Brain
Abstract
In this chapter, we provide a more modern overview of the concept of astrocytes. We summarize the classification of neuroglia, explore astrocyte types in the central nervous system (CNS), delve into the phylogeny of astrocytes, emphasize astrocyte heterogeneity, and then enumerate currently known functions of astrocytes. Many studies have indicated a critical contribution of CNS astrocytes to neural development, structural support, barrier function, homeostatic function, metabolic support, synaptic transmission, regulation of blood flow, higher/integrative brain functions, brain defense, neuroprotection, and response to injury. The concept of the “tripartite” synapse and “astroglial cradle” is discussed, highlighting the intimate relationship of astrocytes and CNS synapses. Finally, the emerging concept of neurological diseases as “gliopathies” is considered. Subsequent chapters will more specifically evaluate glial changes in the context of epilepsy, in both human tissue and animal models.
Keywords
Neuroglia; protoplasmic; fibrous; heterogeneity; phylogeny; tripartite; cradle; gliopathy
Overview
In contrast to Chapter 1: History of Astrocytes, in which a comprehensive history of the concept of glia was developed, in this chapter we will provide a brief overview of the modern concept of glia. We will summarize the classification of neuroglia, astrocyte types in the CNS, phylogeny of astrocytes, astrocyte heterogeneity, and then mention many of the currently known functions of astrocytes. Each of these subsections is not meant to be comprehensive as there are many excellent review articles and textbooks [1] that address each of these issues in more detail. Finally, the emerging concept of neurological diseases as “gliopathies” is considered. Subsequent chapters will more specifically enumerate glial changes in the context of epilepsy, in both human tissue and animal models.
Classification of Neuroglia and Astrocyte Types in the CNS
Neuroglia are classified into peripheral nervous system (PNS) and central nervous system (CNS) glia (Fig. 2.1). There are various forms of specialized PNS glia including Schwann cells (responsible for myelinating and surrounding peripheral nerve axons), olfactory ensheathing cells (OECs, found in the olfactory system), satellite glia (found in peripheral ganglia), and enteric glia (in the enteric nervous system). CNS glia includes macroglia (astrocytes, NG2-glia, and oligodendrocytes, derived from ectoderm) and microglia (derived from mesoderm).

Astrocytes (“star-like cells”) are quite diverse, and in fact the term astrocyte or astroglia encompasses multiple types of structurally and functionally distinct cells. The more that is being learned about astrocytes, the more it is clear that there is great structural and functional heterogeneity among astrocytes, both within and between distinct brain regions [2–4]. Nevertheless, it is useful to briefly review the main features of astrocytes. The largest morphological subdivision has been between protoplasmic astrocytes and fibrous astrocytes. Protoplasmic astrocytes, found in the gray matter of the brain and spinal cord, have several (5–10 in rodent) primary processes which then expand into a complex arborization. Interestingly, this arborization delineates a “domain” and in the normal state there is little overlap between astrocyte domains [5,6] (Fig. 2.2). Each protoplasmic astrocyte extends processes to invest synapses (perisynaptic processes) [7], blood vessels (forming a specialized structure called a perivascular endfoot), and sometimes the pial surface (subpial endfeet, collectively forming the glia limitans) (Fig. 2.3). The processes from a single rodent protoplasmic astrocyte contact approximately 100,000 synapses [5,8].

Left: Each single astrocyte occupies a well-defined territory; astroglial contacts occur only through distal processes and, overall, the overlap between astrocyte territories does not exceed 3–5%. Images show neighboring hippocampal astrocytes stained with different fluorescent dyes. Right: Schematic representation of astrocytic domains, which are organized in rows along the vessels, the latter typically being positioned in the narrow interface between astrocytes. Source: Reproduced with permission from Verkhratsky A, Butt AM. Glial physiology and pathophysiology. Oxford, UK: Wiley-Blackwell; 2013 (Figure 4.33).

In the adult central nervous system (CNS), astrocytes interact with multiple cell types. As depicted in this schematic, they form endfeet on capillaries that induce the blood–brain barrier properties of the cerebral microvasculature. They interact with the cells along the pial surface, as well as at the ventricular lumen, and their processes interdigitate among the neurons, synapses, nodes of Ranvier, and oligodendrocytes. Additionally, they form gap junctions with other astrocytes. Source: Reproduced with permission from Levinson et al. Astrocyte development. In: Rao MS, Jacobson M, editors. Developmental neurobiology. New York: Kluwer Academic/Plenum Publishers; 2005. p. 197–222.
Fibrous astrocytes, found in the white matter of brain and spinal cord and in the optic nerve and nerve fiber layer of the retina, are less arborized than protoplasmic astrocytes, and their cell bodies are located in a regular pattern between the axon bundles. In addition to perivascular and/or subpial endfeet, they also extend perinodal processes to contact the axons at nodes of Ranvier [9]. While fibrous astrocytes exhibit diverse morphology, in general they lack domain organization [10].
Originally, it was thought that astrocytes could be segregated by molecular markers, notably by expression of the intermediate filament protein glial fibrillary acidic protein (GFAP). However, GFAP expression is heterogeneous among astrocyte subtypes. For example, fibrous astrocytes express GFAP to a greater extent than protoplasmic astrocytes, and there is heterogeneity of GFAP expression among protoplasmic astrocytes of distinct brain regions. Astrocyte heterogeneity of form and function in the rule rather than the exception (Fig. 2.4).

Ia, pial tanycyte; Ib, vascular tanycyte; II, radial astrocyte (Bergmann glial cell); III, marginal astrocyte; IV, protoplasmic astrocyte; V, velate astrocyte; VI, fibrous astrocyte; VII, perivascular astrocyte; VIII, interlaminar astrocyte; IX, immature astrocyte; X, ependymocyte; XI, choroid plexus cell. Source: Reproduced with permission from Reichenbach A, Wolburg H. Astrocytes and ependymal glia, In: Kettenmann H, Ransom BR, editors. Neuroglia. New York, NY: Oxford University Press; 2005. p. 20.
A specialized group of astroglia is called radial glia. These cells are elongated astroglia that are prominent during development. They are bipolar cells with ovoid cell bodies and processes that span from the ventricular to the pial surface. Radial glia serve as a scaffold for neuronal migration (Fig. 2.5) [11]. Radial glia-like cells may persist into adulthood, such as in the retina (Müller glia) and cerebellum (Bergmann glia). Radial glia also have a neurogenic role [12,13].

Radial glial cells extend their processes from the ventricular zone (VZ) and subventricular zone (SVZ), where neural progenitors reside, toward the pia. Neuronal precursors attach to the radial glial cells and migrate along their processes toward their final destination. Numerous reciprocal factors released by both neurons and glia regulate the processes of mutual recognition, attraction, adhesion, migration, and final repulsion. Source: Reproduced with permission from Verkhratsky A, Butt AM. Glial physiology and pathophysiology. Oxford, UK: Wiley-Blackwell; 2013 (Figure 4.31).
Given the morphological and functional heterogeneity of astrocytes, identification of “classical” astrocytes requires careful consideration. Kimelberg put forth the following criteria [14,15]:
1. Absence of electrical excitability
2. Very negative membrane potential (−80 to −90 mV)
3. Expression of neurotransmitter transporters (GABA, glutamate)
4. Expression of intermediate filaments (particularly GFAP)
However, while these criteria have heuristic value in categorization, there are many exceptions to these criteria leading to heterogeneity, such as the existence of: (1) GFAP-negative protoplasmic astrocytes; (2) GFAP-positive cells that do not form gap junctions; (3) “GluR” cells that express neurotransmitter receptors but not neurotransmitter transporters [16,17]; and (4) cells that may or may not extend processes to contact distinct boundaries/surfaces (blood vessels, pia, ependyma). Astrocyte heterogeneity is being increasingly recognized [2,3,18].
Phylogeny of Astrocytes
How has the morphology and function of astrocytes evolved in phylogenetic terms? While the evolutionary origins of glia are obscure, in general terms glia have evolved to subserve by-product removal, structural support, phagocytic needs, developmental programming, and circuit modulation [19]. Studies of simpler nervous systems and comparison among mammalian astrocytes (eg, rodent vs human) have led to important clues to the diversity of astrocyte specializations. As we will see, the roles of mammalian glia have become even more complex and integral to nervous system function.
Caenorhabditis elegans
The nervous system of the nematode Caenorhabditis elegans contains exactly 302 neurons and 56 supportive/glial cells, 50 of which are ectodermal and 6 mesodermal in origin. Most of the epithelial-derived glial cells (46/50) are associated with sensory organs called sensilla. The six mesodermal glia are located around a central “nerve ring” and make gap junctions with neurons and muscle cells [1]. Functional roles of C. elegans glia are thought to include: sensilla function, ion homeostasis, development and morphogenesis, phagocytosis, and modulation of neuronal activity and behavior [20–23].
Drosophila
Insects developed diversification of glia [24]. In Drosophila, neuroglia comprises approximately 10% of the cells in the CNS (which has in total about 90,000 cells) but are divided into many types, including surface glia (make the hemolymph–brain barrier), cortex glia (located in the neuropil around axons and synapses), and tract glial cells (covering axonal tracts). Distinct areas of the Drosophila CNS, such as the optic lobe and optic lamina, have further specialization of glia. In insects, the hemolymph–brain barrier is formed by septate junctions between glia (rather than by tight junctions between endothelial cells which comprise the blood–brain barrier of mammals) [1]. Functions of glial cells in insects include ion (especially K+) homeostasis, redistribution of ions via gap junction-connected networks, neurotransmitter homeostasis (histamine in the retina, and Drosophila CNS glia express glutamate transporters dEAAT1 and dEAAT2 and glutamine synthetase), control of circadian rhythms, and trophic support for neurons [1,25].
Mammals
The remarkable increase in complexity and size of the mammalian brain is paralleled by similarly remarkable increases in glial number and complexity [26,27]. In addition, comparison among mammalian species has led to interesting insights. Glia:neuron ratio has generally increased in phylogeny as brain size has increased [27,28], although this is controversial [29]. Much more impressive than glia:neuron ratio is the marked difference in morphology of individual astrocytes by species. Notably, human astrocytes are much larger and more complex than rodent astrocytes [26,30] (Fig. 2.6). The mean diameter of a human protoplasmic astrocyte (142 µm) is about 2.5 times larger than rat (56 µm) and the astrocyte domain volume is about 16.5 times larger. Human fibrous astrocytes also are about 2.2 times larger in humans than rodents [30]. Perhaps most remarkably, a single human protoplasmic astrocyte has about 10 times more primary processes and manifold more fine processes than a rodent astrocyte [30]. Therefore a single human astrocyte may contact about 2 million synapses within its domain volume compared to about 100,000 in the rodent [5,26,30]. By contrast, volumetric neuronal synaptic density and the number of synapses per neuron is quite similar between rodents and primates. In addition, primates appear to have distinct populations of specialized astrocytes not found in other mammals: “interlaminar astrocytes” whose processes penetrate through the cortex and end in layers III and IV [31–34]; and “polarized astrocytes” dwelling in layers V and VI. Terminal processes of interlaminar astrocytes were reported to be particularly large in Albert Einstein’s brain [35] but the significance is unclear. To assess the cell-autonomous properties of human astrocytes, Han and colleagues engrafted human glial progenitor cells into neonatal immunodeficient mice. Engrafted human glia integrated with host astrocytes, propagated Ca2+ signals threefold faster than native mouse astrocytes, and most strikingly supported enhancement of long-term potentiation of synaptic transmission (LTP) and improved learning in multiple paradigms [36]. This remarkable result suggests a relationship between cognitive performance and astrocyte complexity for the first time.

(A) Typical mouse protoplasmic astrocyte. GFAP, White. Scale bar, 20 µm. (B) Typical human protoplasmic astrocyte in the same scale. Scale bar, 20 µm. (C) and (D) Human protoplasmic astrocytes are 2.55-fold larger and have 10-fold more main GFAP processes than mouse astrocytes (human, n=50 cells from 7 patients; mouse, n=65 cells from 6 mice; mean±SEM; *P=0.005, t test). (E) Mouse protoplasmic astrocyte diolistically labeled with DiI (white) and sytox (blue) revealing the full structure of the astrocyte including its numerous fine processes. Scale bar, 20 µm. (F) Human astrocyte diolistically labeled demonstrates the highly complicated network of fine process that defines the human protoplasmic astrocyte. Scale bar, 20 µm. Inset, Human protoplasmic astrocyte diolistically labeled as well as immunolabeled for GFAP (green) demonstrating colocalization. Scale bar, 20 µm. Source: Reproduced with permission from Oberheim et al. Uniquely hominid features of adult human astrocytes. J Neurosci 2009;29:3276–87.
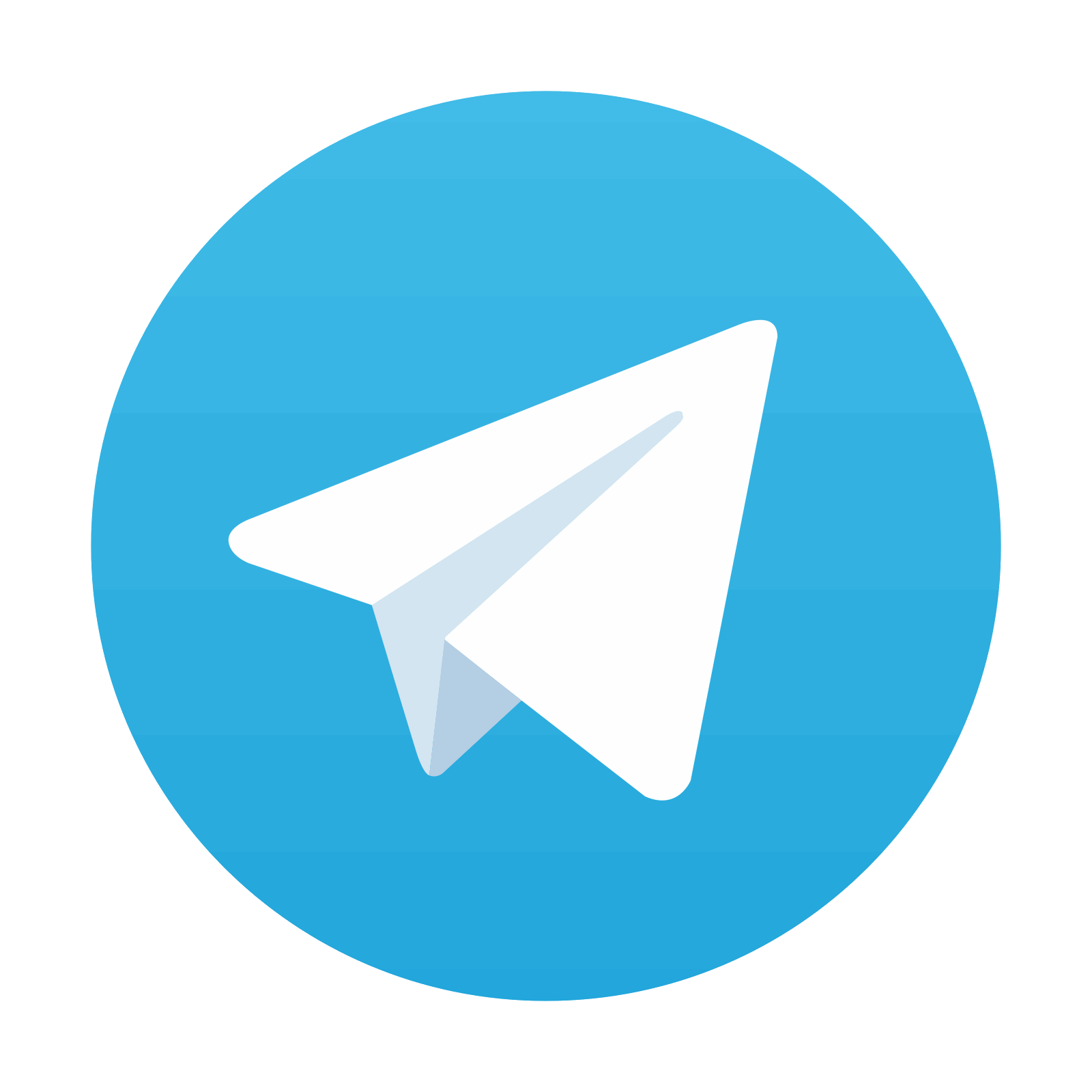
Stay updated, free articles. Join our Telegram channel

Full access? Get Clinical Tree
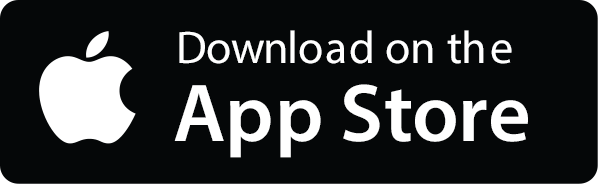
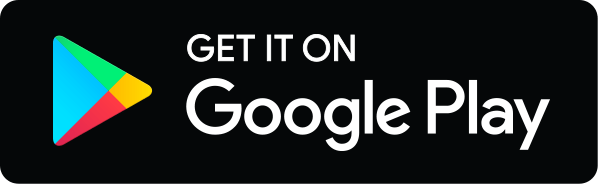
