Abstract
The cardiac autonomic nervous system (ANS) is a multilevel distributed neural network that extends from intrathoracic ganglia to the spinal cord, brainstem, and above. This interdependent network of central and peripheral reflexes control chronotropy, lusitropy, dromotropy, and inotropy on a beat-to-beat basis. Cardiac afferent input is projected to each of these processing centers with the ultimate outcome of modulating sympathetic and parasympathetic efferent inputs to all regions of the heart. Within peripheral autonomic ganglia, reflex processing of afferent input with descending projections from higher centers is dependent upon local circuit neurons. Herein, we outline the structural and functional organization of the hierarchy for cardiac control and how this neural network remodels in response to cardiac pathology. The fundamental premise is that the evolution of cardiac disease is dependent on dynamic interactions between the cardiac substrate and the neural networks that control it.
Keywords
Arrhythmia, Cardiac autonomic innervation, Myocardial infarction, Parasympathetic, Sympathetic
Outline
Cardiac Afferent Neurons 1265
Cardiac Efferent Neurons 1267
Local Circuit Neurons 1267
Interactions Among Autonomic Neurons in the Coordination of Regional Cardiac Indices 1267
Overview 1267
Intrinsic Cardiac Neuronal Interactions 1268
Intrathoracic Extracardiac Reflexes 1268
Central-Peripheral Reflex Interactions in the Coordination of Cardiac Indices 1268
Cardiac Autonomic Dysfunction Secondary to Cardiac Pathology 1268
Conclusion 1269
References 1270
Autonomic control of the heart is achieved by a complex, multilevel, interconnecting network involving central and peripheral reflexes. This network is made up of aggregates of neurons in intrathoracic (intracardiac and extracardiac) ganglia, extrathoracic sensory ganglia (nodose, dorsal root ganglia, and petrosal ganglia), and the central nervous system (CNS) ( ). The cardiac ANS is responsible for the reflex control of chronotropy, lusitropy, dromotropy, and inotropy, doing so via modulation of sympathetic and parasympathetic efferent inputs to the heart ( ). Fig. 104.1 summarizes the structural/functional organization for cardiac control. Rational neuromodulation for cardiac disease is predicted on understanding these nerve networks and the impact of therapy on integrated neural regulation. To this end, we first review the critical structural neural elements for cardiac control and then how these elements are interlaced within the hierarchy for cardiac control.

Cardiac Afferent Neurons
Cardiac control, first and foremost, depends on cardiovascular sensory transduction by populations of afferent neurons in nodose, dorsal root, intrinsic cardiac, stellate, and middle cervical ganglia. Normally, these afferent inputs evoke a balanced and integrated control of regional cardiac function that involves central and peripheral aspects of the ANS ( ). With respect to evolving cardiac pathology, however, nociceptive sensory inputs arising from the ischemic heart represent a stimulus that destabilizes neural network interactions throughout the hierarchy ( ), contributing to an increased potential for sudden cardiac death ( ). As a corollary, targeting these multilevel reflex pathways represents an emerging neuromodulation-based, therapeutic option for managing cardiac arrhythmia, angina, and/or pump failure.
The afferent sensory neurites can be mechanosensory, chemosensory or multimodal in nature ( ). The chemicals that are transduced include adenosine, adenosine triphosphate (ATP), bradykinin, substance P, and other peptides ( ). It is also known that the transduction properties of cardiac sensory neurites involve a number of ion species in situ ( ). There are also mechanosensory afferent neurites located in the fibrous coating of the major vessels adjacent to the heart (the aortic arch) and in the carotid sinus. These neurites respond to change in length and stretch of the vessel walls and transduce information to the nucleus tractus solitarius (NTS).
The characteristics of afferent activity depend on their spheres of influence as defined by their soma and sensory neurite location, the strength of the applied stimulus, the context/history of the applied stimulus, and the potential for information processing ( ). Furthermore, the time course of sensory transduction can outlive the primary stimulus; that is to say, sensory inputs can exhibit memory functions ( ). The net result of this organization is that limited numbers of afferent inputs are capable of responding to and transducing complex sensory environments in short-, mid-, and long-time frames.
While bipolar, cardiac afferent neurons in nodose ganglia project to neurons in the NTS of the medulla oblongata ( ), those in the dorsal root ganglia project first to spinal cord neurons ( ). Petrosal ganglia project to the NTS ( ). Autonomic sensory inputs, acting via central interneurons, reflexly modulate cardiac parasympathetic efferent preganglionic neurons in the medulla and spinal cord sympathetic efferent preganglionic neurons, respectively, for the control of cardiac adrenergic and cholinergic efferent outflow ( ).
Cardiac Efferent Neurons
Cardiac tissues are modulated by sympathetic and parasympathetic motor neurons as well as circulating hormones. Activation of the sympathetic nervous system (SNS) increases heart rate, blood pressure, and cardiac contractility ( ). In relation to electrophysiological properties, cardiac efferent sympathetic activation enhances cardiac electrical conduction by shortening the action potential (AP) duration, enhancing atrioventricular nodal conduction, and increasing sinoatrial firing rate ( ). Parasympathetic activity exerts directionally opposite effects, exerting negative chronotropic, inotropic, and dromotropic effects ( ). Sympathetic-parasympathetic interactions for control of regional cardiac function may be manifest at the end-effectors ( ), within peripheral autonomic ganglia ( ), and within the CNS ( ).
Cardiac, sympathetic efferent preganglionic neurons located in the caudal cervical and cranial thoracic spinal cord project axons via the right- and left-sided spinal nerves to sympathetic efferent postganglionic neurons in ganglia located in the neck and thorax ( ). The latter neurons are located in the superior and middle cervical ganglia, stellate ganglia, and mediastinal ganglia ( ) with lesser numbers synapsing with sympathetic efferent postganglionic neurons located throughout the major atrial and ventricular ganglionated plexuses ( ). The right postganglionic sympathetic nerve fibers predominantly cover the ventral aspect, whereas the left predominantly cover the dorsal aspect of the heart ( ). Neural network interconnections between the CNS and extracardiac ganglia, including those intrinsic to the heart, facilitate coordination of regional cardiac electrical and mechanical function to ensure adequate cardiac output to meet whole body flow requirements ( ).
The parasympathetic efferent projections to the heart reduce heart rate and cardiac contractility, delay atrioventricular electrical conduction, and prolong ventricular AP duration ( ). The preganglionic soma are located within the nucleus ambiguous and the dorsal motor nucleus of the medulla ( ) with peripheral projections coursing the 10th cranial nerve (vagus). The preganglionic fibers project to divergent aggregate of intrinsic cardiac ganglia, located in atrial and ventricular tissues ( ). Specific ganglia preferentially control different aspects of cardiac function such as sinoatrial node activity, but there is sufficient redundancy within the system that even when one ganglia is removed, other ganglia within the intrinsic cardiac nervous system (ICNS) take over the control of that region ( ). As the result of convergence of parasympathetic, preganglionic projection neurons to the ICNS and interconnections manifest between aggregates of intrinsic cardiac ganglia, there is balance in control of all regions of the heart, as exerted from right and left vagus ( ).
Local Circuit Neurons
Local circuit neurons exist throughout all of the intrathoracic ganglia, including the ICNS ( ). By local circuit neurons we mean neurons that are not directly transducing cardiac indices (cardiac afferent neurons) or having direct motor function. These neurons play a principal role in integrating sensory inputs from the heart and major intrathoracic vessels with descending inputs from central autonomic motor neurons ( ). The local circuit neurons can be responsible for processing only afferent or efferent information or they can be convergent; in other words, they receive both afferent and efferent inputs ( ). There is another population of local circuit neurons that don’t receive either afferent or efferent inputs and their function remains elusive and yet to be determined.
Interactions Among Autonomic Neurons in the Coordination of Regional Cardiac Indices
Overview
Intrathoracic ganglia function as centrifugal relays, distributing efferent sympathetic (intrathoracic extracardiac ganglia) or parasympathetic (intrinsic cardiac ganglia) information to the heart ( ). They also process centripetal information ( ), specifically ascending afferent projections that reflexly modify efferent projections to the heart at multiple levels of the hierarchy for cardiac control. The complex functional interactions that occur among neurons within these various intrathoracic ganglia utilize excitatory and inhibitory synapses ( ). As schematically represented in Fig. 104.1 , afferent inputs are fundamental to the multilevel coordination of reflex action, from the intrinsic nervous system, extracardiac intrathoracic ganglia, spinal cord, and brainstem, with higher centers modulating lower level, cardiocentric processing ( ). These reflexes subserve short-term coordination of electrical and mechanical function of the heart while ensuring long-term control of cardiac output ( ).
Intrinsic Cardiac Neuronal Interactions
The ICNS functions as the final common pathway for cardiac control ( ). The intrinsic cardiac neural network contains afferent, efferent, and local circuit neurons that are capable of controlling regional cardiac function even when chronically disconnected from all higher centers ( ). There is a growing body of evidence, which indicates that intrinsic cardiac neurons utilize a variety of neurochemicals. Intrinsic cardiac neurons can also be modified by locally applied nicotinic, muscarinic, β-adrenergic, amino acidergic, peptidergic and purinergic agonists ( ). The clinical efficacy of angiotensin-converting enzyme (ACE) inhibitor therapy or β-adrenergic blockade may be due in part to altering activity within and between aggregates of neurons contained within the ICNS ( ).
Intrathoracic Extracardiac Reflexes
Reflex control of the heart depends in part upon neuronal circuits located in the intrathoracic (middle cervical, stellate and mediastinal) sympathetic ganglia ( ). These intrathoracic, extracardiac reflexes control, in part, adrenergic efferent neurons innervating the heart ( ). Cardiac pathology leads to remodeling of the peripheral circuits ( ). The clinical relevance of these peripheral circuits are evidenced by the effective treatment of refractory ventricular tachycardia by bilateral stellate decentralization, a surgical procedure that leaves intrathoracic autonomic reflexes mostly intact ( ). These peripheral reflexes may also be modified by spinal cord stimulation (SCS) with a consequent stabilization of cardiac arrhythmia potential ( ) and preservation of myocyte viability ( ). A primary theme that is emerging from these studies is that neuromodulation therapy targeting primary nexus points for cardiac control has the greatest potential to impact disease progression while minimizing the potential to induce autonomic imbalances that, by their very nature, induce adverse cardiac remodeling ( ).
Central-Peripheral Reflex Interactions in the Coordination of Cardiac Indices
Intrathoracic reflex circuits involve peripheral sensory, local circuit, and efferent motor neurons ( ). These circuits impart a dynamic, short-loop coordination of regional cardiac electrical and mechanical function ( ). Superimposed upon these peripheral reflexes are those mediated by the CNS that involve spinal cord and medullary neurons, ones that are essential to coordinating cardiac function with whole-body blood-flow demands ( ). This concept is predicated upon the fact that neuronal somata in the various intrathoracic extracardiac and intrinsic cardiac ganglia are in constant communication, not only with each other, but also with neurons in the spinal cord, medulla oblongata, and higher centers in the initiation of interdependent feedback control of regional cardiac indices. Normally functioning in an interdependent fashion, imbalances in network function within the hierarchy for cardiac control can manifest in deleterious fashion, sometimes with lethal consequences ( ). Mechanistically understanding these structural/functional changes is fundamental to evolving new therapeutic targets ( ).
Cardiac Autonomic Dysfunction Secondary to Cardiac Pathology
During a cardiac insult, such as in the case of myocardial ischemia, the cardiac SNS augments its activity and results in an increase in heart rate, cardiac contractility, and left ventricular systolic pressure. This is vital to maintain life, but problems arise when persistent neural activation of the SNS occurs coupled with a suppressive effect on parasympathetic activity ( ). This maladaptive state can precipitate atrial and ventricular arrhythmias, contribute to worsening heart failure (HF), and result in sudden death.
Persistent maladaptations of neuronal activity results in functional reorganization within the cardiac ANS ( ). This plasticity occurs at every level within the system from the CNS to the intrathoracic cardiac ganglia ( ). The changes that occur can be brief or long term, resulting in exaggerated reflex responses within the system. Persistent afferent signaling that arises from a site of injury, as in the case of myocardial infarction (MI), elicits a process that changes the excitability of cell membranes, results in central sensitization of neurons and reduces the natural inhibitory mechanisms that help balance the system. The cardiac afferents appear to be involved in initiating local inflammatory and vascular cascade reactions and as such may be responsible for adverse cardiac remodeling ( ).
Morphological changes that occur in the system that are secondary to MI include neuronal enlargement, altered neurochemical expression patterns, and enhancement of neural signals in the stellate ganglion. Nerve sprouting around the ischemic territory has also been described with nerve growth factor upregulation ( ). There is transformation of cholinergic neurons to adrenergic expression which partly contributes to the adrenergic overexpression ( ). Reduced nitric oxide, increased oxidative stress, and activation of angiotensin II–type receptors all contribute to the centrally driven increase in sympathetic activity. This is likely as a result of abnormal afferent feedback. Despite the sympathetic hyperactivity, there exists a paradoxical reduction in norepinephrine synthesis with a downregulation of tyrosine hydroxylase, and decreased norepinephrine levels in the myocardium ( ).
Therapies aimed at reducing sympathetic input to the myocyte have prognostic benefit for the treatment of ventricular arrhythmias ( ). These therapies involve surgical resection of the lower third of the stellate ganglion to the fourth thoracic, vertebral ganglion or injection of local anesthetic to the stellate ganglion under fluoroscopic guidance ( ). It may be possible to resect less of the sympathetic paravertebral ganglia, resulting in less off-target effects and still maintain efficacy ( ). Renal sympathetic denervation is also being studied as a potential treatment, not only for hypertension. but for HF and ventricular arrhythmias, as well ( ).
While atrial arrhythmias can be precipitated by both adrenergic and cholinergic triggers, modulation of the ANS, by altering sympathetic or parasympathetic inputs to the myocyte, is of considerable interest for the treatment of atrial fibrillation ( ). This can be achieved with ablation of the ICNS or vagal nerve stimulation (VNS), but the mechanism behind atrial fibrillation remains poorly understood. As such, therapies targeting the ANS for atrial fibrillation remain questionable. Further mechanistic work needs to be completed before the ideal nexus point can be identified and targeted.
Heart rate variability, a marker of sinus node automaticity under the control of the ANS, is reduced in the setting of cardiac dysfunction. This is particularly the case in the presence of an MI or HF and is a poor prognostic indicator ( ). Alteration in baroreflex control is also disrupted in HF as a result of excessive sympathetic activation and progressive inability of the end-terminus nerve terminals to release or reuptake catecholamines ( ). Activation of the carotid baroreceptor is thought to confer benefits in prevention of sudden cardiac death in patients with HF and may prove to be a safe and effective therapy for HF one day.
Finally, inherited arrhythmias such as long QT, Brugada syndrome, idiopathic ventricular fibrillation, and catecholaminergic polymorphic ventricular tachycardia are affected by the ANS. These inherited disorders are predominantly affected by sympathetic activation, except in the case of Brugada syndrome or some forms of long QT syndrome, where increased parasympathetic activation precipitates arrhythmias. Modulation of the ANS with medications such as beta-blockers or surgical resection of the sympathetic paravertebral ganglia have shown some benefits for the treatment of sympathetic driven channelopathies ( ).
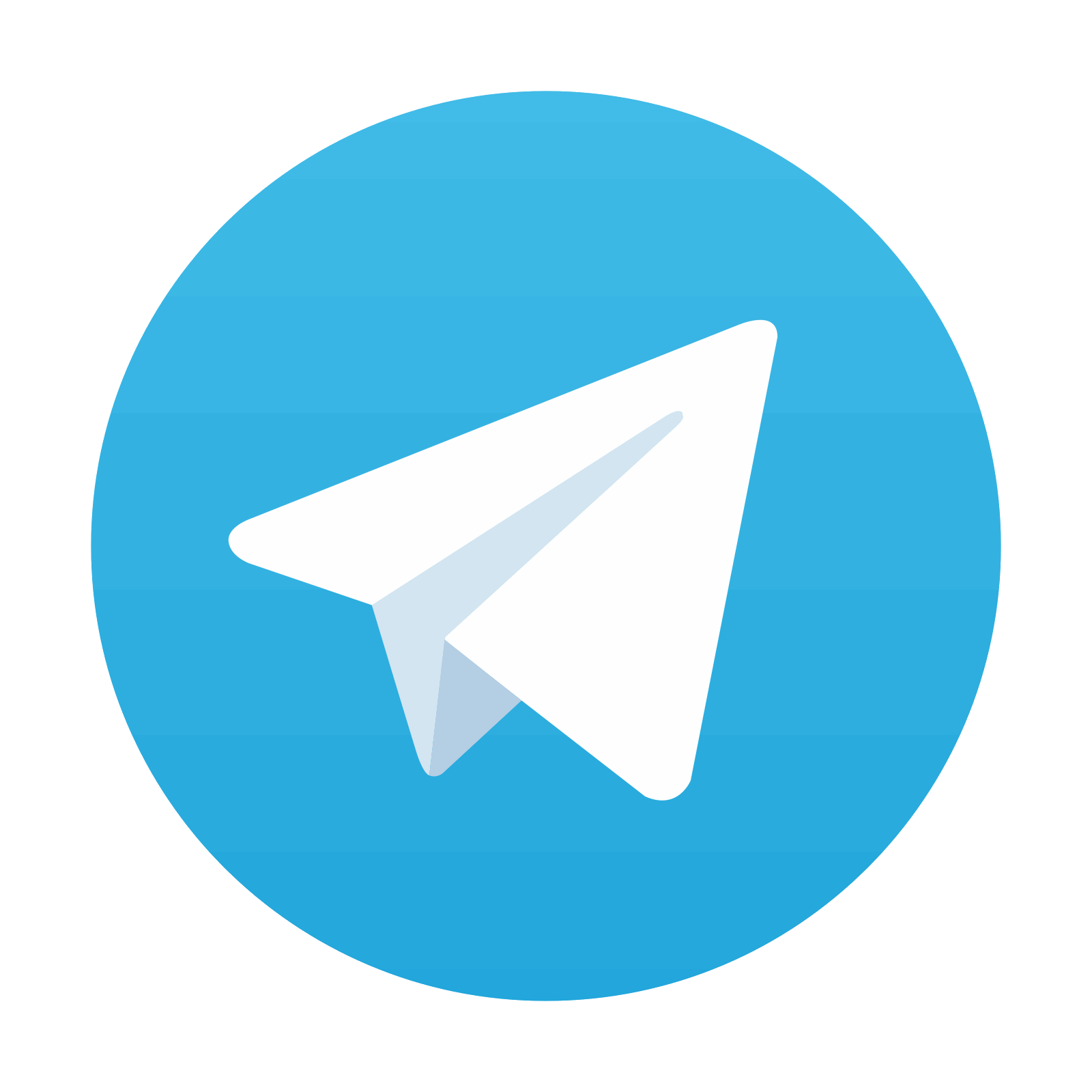
Stay updated, free articles. Join our Telegram channel

Full access? Get Clinical Tree
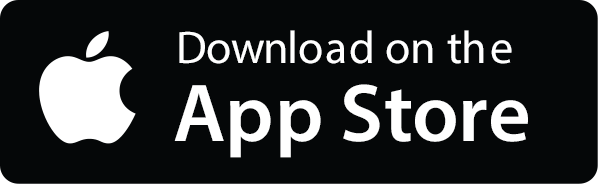
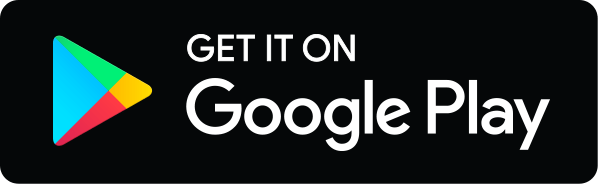