Abstract
Postural instability is a major problem in Parkinson’s disease (PD), being poorly responsive to l -dopa treatment. There is now hope that deep brain stimulation (DBS) of the pedunculopontine tegmental nucleus (PPTg) may ameliorate posture problems, likely acting on nondopaminergic pathways. To explore this possibility we investigated PPTg DBS on fluctuations in the center of pressure (CoP) during upright stance. Total length of oscillations (TL) increased in patients under different drug/DBS and eye-state combinations when compared with controls. CoP displacement velocity was higher in off-drug/off-DBS when compared with controls, but was lower when comparing off-drug/on-DBS versus off-drug/off-DBS. The Romberg’s index (RI) referring to sway ellipse (SE) did not change when comparing controls versus the four studied treatments. In contrast, significant increases occurred in the RI for TL when comparing controls versus each treatment. TL and SE were higher when l -dopa-treated patients kept their eyes closed, but decreased irrespective of eye state when DBS was applied. We conclude that PPTg DBS may improve l -dopa-resistant axial and postural abnormalities in PD.
Keywords
Deep brain stimulation, l -Dopa, Parkinson’s disease, Pedunculopontine tegmental nucleus, Static stabilometry
Acknowledgments
This work was partly supported by small RIA grants (2014–2015) from University of L’Aquila.
Introduction
Postural instability is a severe symptom in Parkinson’s disease (PD) and atypical Parkinsonisms that causes falls and postural deformities ( ). The treatment of postural instability in PD with antiParkinsonian drugs is unsatisfactory, and frequent falls occur in patients who experience good pharmacological control of other motor disabilities ( ).
The origin of this postural instability that leads to falling is unclear. It has been hypothesized that postural instability in PD patients may result from dysfunctions of processing and integration of sensory signals and deficits in motor adjustment processes and muscle tone regulation. Correct sensory processing requires the integration of visual, vestibular, and somatosensory signals, so that motor adjustments can be executed in the presence of proper basic muscle tone. In normal subjects control mechanisms occur in a normal fashion, and visual cues play an essential role in overall balance control. In contrast, in PD patients one or all of these control systems is affected ( ), and balance becomes highly dependent on visual cues.
Deep brain stimulation (DBS) of basal ganglia nuclei has been applied in an attempt to improve postural instability in l -dopa-resistant PD and other Parkinsonian syndromes. The subthalamic nucleus (STN) and globus pallidus internus (GPi) have been the most common targets for DBS ( ) in an attempt to improve postural control in PD. The results of these and other related studies were the object of a recent comprehensive review ( ). In brief, unsatisfactory results for STN DBS and GPi DBS on balance disorders have been reported in PD patients, and even aggravation of imbalance has been observed in some patients. Also, a combination of l -dopa with DBS has yielded unsatisfactory results, in particular when medication has been used with STN DBS.
In the last decade, in patients with PD who were poorly responsive to pharmacological and/or surgical therapies, the pedunculopontine tegmental nucleus (PPTg) has emerged as a promising DBS target ( ). Besides the fact that stimulation of this structure has been found to elicit locomotion in animals, interest in the PPTg has been raised by the possibility that it may serve as a basal ganglia output to motor spinal cord mechanisms, bypassing both the thalamocortical route and dopaminergic nigrostriatal mechanisms ( ), and facilitating arousal and reward mechanisms that might increase patient attention in the production of voluntary movements ( ). In addition, it has been shown that PPTg DBS may modulate somatosensory evoked potentials, suggesting that it could also affect integration and processing of sensory signals ( ).
The relatively low number of PPTg DBS patients does not allow one to draw definitive conclusions concerning the role of PPTg in motor control, but improvement of freezing of gait and a reduction of falls have been consistently reported, despite some inconsistencies in gait parameters that are likely due to patient selection, stage of disease, and precise site of stimulation. In a previous study ( ) we reported that PPTg DBS improved gait initiation and specific spatio–temporal and kinematic parameters during unconstrained walking, suggesting that the improved gait initiation may help overcome the block of preparation for movement that is present in gait freezing.
Given these premises, the aim of our study was to assess the effects of unilateral PPTg stimulation on postural balance in patients who were previously subjected to gait analysis ( ). Postural balance was assessed by evaluating fluctuations in the center of pressure (CoP) during upright stance. We used the CoP to assess postural balance because it represents a reliable index of the point location of the ground reaction force vector, reflecting the sway of the body and the forces used to maintain the center of gravity within the support base.
Methods and Materials
Patients
Ten male PD patients who completed 1 year of follow-up were studied. All patients gave informed written consent, and the protocol was approved by the local ethics committee. Surgical procedures and details for targeting, implanting, and stimulating the PPTg have been previously described ( ). Briefly, all patients were implanted under general anesthesia with the 3389 DBS lead (Medtronic Neurological Division, Minneapolis, MI, USA). The PPTg targeting was planned on the basis of stereotactic angio computed tomography (CT) scans, using the pontomesencephalic junction line and the obex as reference points. In the midsagittal slice we identified the axial level for the determination of the z plane, corresponding in the patient’s anatomy to the PPTg. The x and y coordinates were calculated by overlapping the axial CT scans with slides +31 to +36 mm from the obex of , in which the PPTg is reported. A three-dimensional virtual surgery system allowed us to reproduce the surgical procedure exactly and follow an angled trajectory, which proved to be avascular, intraparenchymal, and extraventricular. In this way surgery was tailored according to the patient’s anatomy, thus overcoming the issue of large differences that occur in the brainstem from patient to patient. Intraoperative monitoring of somatosensorial-evoked potentials, recorded through the electrode contacts, allowed us to evaluate the correct position of the stimulating electrode ( ).
The demographic characteristics of patients, stimulation parameters, and drug treatment are given in Table 79.1 . An overview of electrode position in the studied patients is reported in Fig. 79.1 . The electrode implantation was contralateral to the most disabled hemisoma, thus nine patients were implanted on the right side and one on the left side.
Total Studied Patients | 10 |
---|---|
Age | 60.2 ± 7.4 years |
Sex | Male |
Disease duration | 10.8 ± 9.5 years |
Preoperative UPDRS part III items 27–30 | 5.3 ± 3.0 |
Postoperative UPDRS part III items 27–30 | 2.8 ± 0.9 |
Preoperative Hoehn and Yahr scale | 3.5 ± 0.6 |
Postoperative Hoehn and Yahr scale | 2.6 ± 0.8 |
Preoperative levodopa equivalent daily dose | 1050.1 ± 397.9 mg |
Postoperative levodopa equivalent daily dose | 350.0 ± 275.8 mg |
Stimulus amplitude | 2.5 − 3.0 V |
Stimulus pulse width | 60 μs |
Stimulus rate | 40 Hz |

Stabilometry and Postural Instability
A stabilometric platform (Global Postural System, GPS 400, Chinesport SpA, Udine, Italy) was used to evaluate the displacement of the CoP and variation of the confidence ellipse area (i.e., the area in which consecutive positions of the CoP were included), with eyes either open or closed. To measure postural sway, the barefoot patients were instructed to maintain an upright standing position on the platform with arms unfolded at their sides. According to international standard procedures, patients were first asked to remain with head straight and eyes open, and instructed to maintain their gaze on a fixed point 90 cm in front of them that was placed at eye level for each patient ( ). Feet were kept at an angle of 30 degrees and the distance between the heels was 2 cm. Before starting the platform recording, the tendency of patients to fall was evaluated using the pull test, according to .
During the stabilometric evaluation, four treatments were tested: drugs-off/DBS-off (off/off), drugs-on/DBS-off (on/off), drugs-off/DBS-on (off/on), and drugs-on/DBS-on (on/on). Ten age-matched healthy subjects were used as controls. Treatments were tested in the following order.
- 1.
Off/off (medication and DBS were suspended 12 h and 20 min before stabilometry, respectively).
- 2.
On/off (stimulation was switched off 20 h before stabilometry). This time interval was chosen on the basis of the observation that the effects of PPTg DBS decline within 20 h of ceasing stimulation ( ).
- 3.
Off/on (medication was suspended 12 h before stabilometry).
- 4.
On/on (stabilometry was performed 2–3 h after the first daily medication dose).
The evaluation of CoP variations was performed during a standard task using three parameters.
- 1.
The sway ellipse (SE, mm 2 ), which corresponds to the area that contains 90% of the positions of the sampled CoP values.
- 2.
The total length (TL, mm) of oscillations, which corresponds to the sum of the distances between each position of the CoP.
- 3.
The mean velocity (MV, mm/ms) of CoP displacement, which corresponds to the average velocity of the CoP calculated by dividing the TL of the CoP trajectory by the recording time.
Ten minutes after the first task, a second session was performed to evaluate the Romberg’s index (RI). For this purpose patients were requested to repeat the stabilometric test, but this time with their eyes closed. Thus the RI was calculated as the ratio [(SE) or (TL) (eyes closed)/(SE) or (TL) (eyes open)] × 100.
Statistics
One-way or two-way ANOVA for repeated measures was used to compare the postural parameters across the four treatments and the two eye conditions (open vs. closed). Post hoc comparison with a Newman–Keuls test was used to compare means across treatments. The statistical package software STATISTICA 8.0 (Statsoft Inc., Tulsa, OK, USA) was used. Values are given as mean ± standard deviation (SD)
Results
Fig. 79.1 illustrates the position of the stimulating electrode in each operated patient as inferred from magnetic resonance imaging (MRI). Note that the negative contact of the active pair was always located in the pontine extension of the PPTg, clearly below the pontomesencephalic junction.
Fig. 79.2 shows that a significant increase in the number of falls in the pull test occurred in the off/off state when compared to controls. Falls were significantly reduced in patients under on/off, off/on, and on/on treatments when compared to patients in the off/off state. On/on was the most effective combination of all those tested. This means that a synergistic effect of drug and DBS had occurred, improving the patient’s ability to respond with an appropriate postural adjustment to the sudden retropulsive movement induced by the examiner (pull).

Fig. 79.3 shows that, as far as the SE was concerned, there was a significant increase in on/off when comparing controls in the two eye states. However, there was a trend of DBS to reduce SE, irrespective of the eye state, which became statistically significant when comparing on/off versus off/on when patients kept their eyes closed. There was also a synergistic effect to combining drug and DBS which resulted in a significant increase of SE when comparing controls versus on/on, regardless of eye state.

Fig. 79.4(A) shows that a significant increase occurred in TL in each of the experimental conditions when compared with controls independent of eye state, with the effect being more evident when the eyes were closed. In regard to MV ( Fig. 79.4B ), there was a significant increase in off/off when comparing this treatment with controls, while a significant decrease occurred by comparing off/on versus off/off in both eyes open and eyes closed conditions. Thus this data supports the idea that in the absence of visual input, the ability of patients to maintain their balance was worsened to the point that neither medication, nor stimulation, nor their combination was effective in restoring normal postural control.

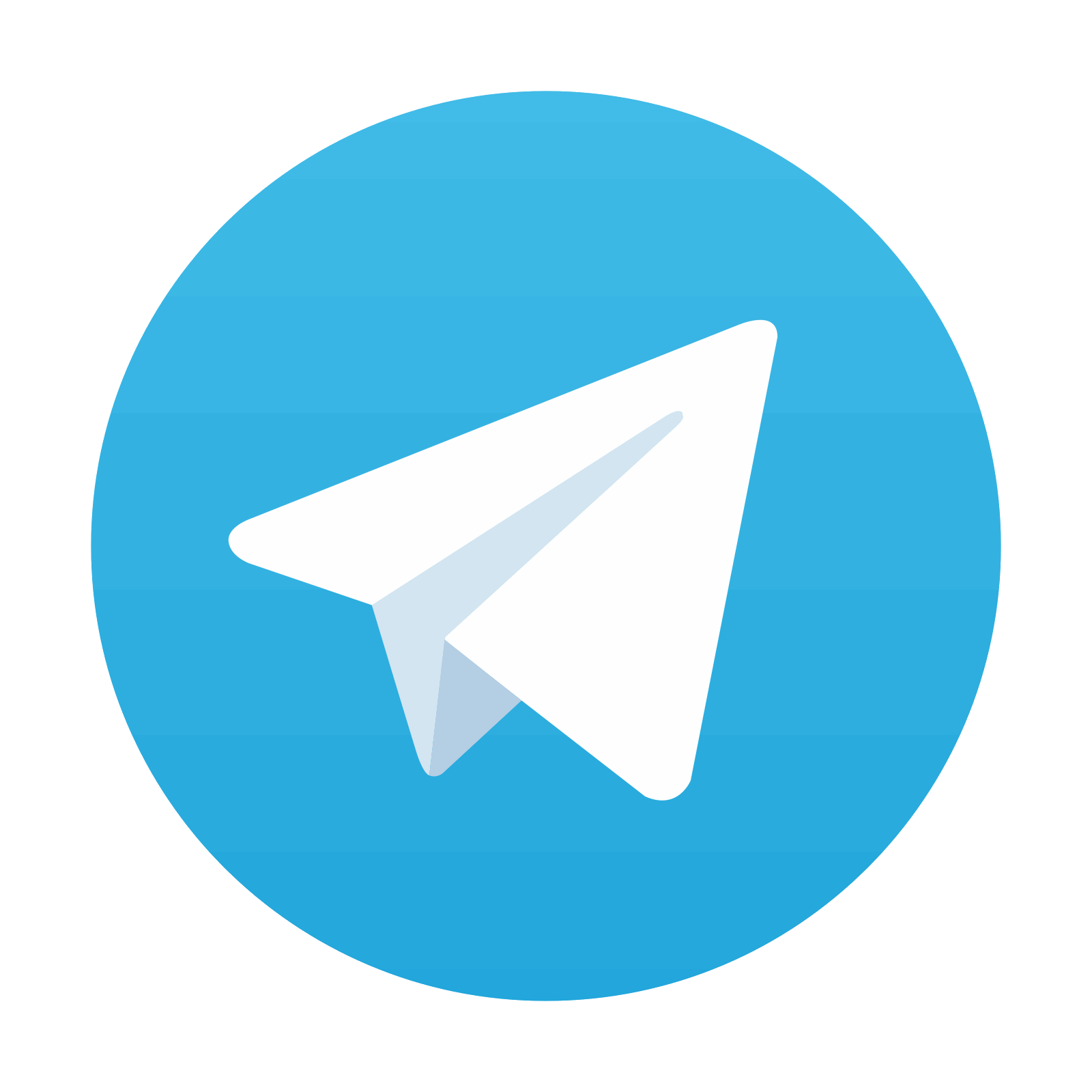
Stay updated, free articles. Join our Telegram channel

Full access? Get Clinical Tree
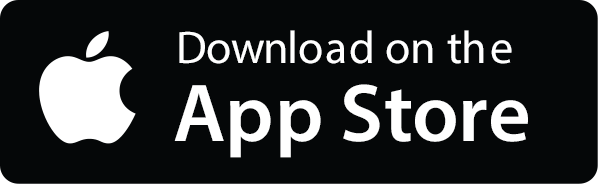
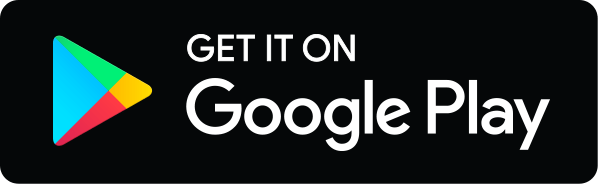
