CHAPTER 292 Bone Graft Options, Bone Graft Substitutes, and Bone Harvest Techniques
In 1998, individuals spent $91 billion (1% of the U.S. gross domestic product) to treat back pain directly and $26 billion (2.5% of national health care expenditures) on other expenditures attributable to back pain.1 Compression of neural elements, malalignment, and abnormal motion across intervertebral segments are three surgically correctable causes of back pain. Unlike limited neural decompression, such as hemilaminotomy for removal of a herniated disk fragment, more extensive decompression can compromise the structural integrity of a spinal segment, thereby necessitating fusion, or arthrodesis, across that motion segment. Spinal arthrodesis is also required in situations of gross spinal instability as occurs in traumatic fractures, pathologic fractures, and rheumatologic disorders. Despite significant advances in spinal instrumentation technology and surgical techniques, without solid bony fusion, all spinal instrumentation will eventually fail, highlighting the essential role of bone grafting for successful arthrodesis.
History of Spinal Arthrodesis
Autogenous bone graft was first used to induce spinal fusion in 1911 by Albee2 and Hibbs.3 Albee used tibial grafts placed between spinous processes for patients with Pott’s disease, and Hibbs used laminar and spinous process bone fragments for dorsal fusion along decorticated facet joints. Their idea of creating a rigid union between vertebral segments to correct segmental instability was the basis of further advancements in spinal fusion over the next several decades.
Beginning in 1933, Burns4 used an anterior approach for lumbar interbody graft placement for spondylolisthesis. Cloward,5 among others, used a posterior approach to lumbar interbody graft placement. From the 1930s through the 1960s metallic internal fixation devices were developed by pioneering spine surgeons such as Harrington, Luque, Judet, Roy-Camille, Magerl, and Wiltse, revolutionizing spinal stabilization. More recently, advances in biomechanics, metallurgic sciences (metal alloys), bone allograft material, and instrumentation design have continued to improve fusion rates.
A growing understanding of bone physiology also played a role in the advancement of spinal fusion techniques and the achievement of higher fusion rates, particularly in patients at higher risk of nonunion. In the 1960s a new set of cell-signaling proteins, bone morphogenetic proteins (BMPs), was discovered and characterized by Urist.6,7 These proteins have since been shown to enhance spinal arthrodesis, thus lessening the need for autograft harvesting and reducing the morbidity associated with it.8 As science continues to elucidate the biology of bone formation, the techniques of bone grafting and spinal fusion will continue to evolve.
Principles of Bone Physiology
Bone is a dynamic tissue in a continuous state of metabolic activity (deposition, resorption, and remodeling). In fact, the human body replaces its skeletal mass once every 10 years.9 There are several cell types important in bone growth and formation: osteogenic precursor cells, osteoblasts, osteoclasts, osteocytes, and hematopoietic elements of bone. Osteogenic precursor cells are located in the deep layer of the periosteum and are present on all nonresorptive bone surfaces, serving as a major component of the covering of bone.10 Osteoblasts are metabolically active, mature bone-forming cells. They primarily secrete an unmineralized organic matrix (osteoid), which mineralizes to give the bone strength and rigidity. As osteoblasts secrete osteoid and become trapped within bone matrix, they mature and become osteocytes. Osteocytes are key players in the control of extracellular concentrations of calcium and phosphorus, as well as in adaptive remodeling of bone.11 Osteoclasts are bone-resorbing cells affected by hormonal and cellular mechanisms.
These cellular components are the building blocks for the three types of bone: woven bone, cortical bone, and cancellous bone. Woven bone is present during embryonic development and fracture healing (callus formation).12 Comprised of randomly arranged collagen bundles and vascular spaces lined with osteoblasts, woven bone is remodeled and eventually replaced with cortical (lamellar) or cancellous (trabecular) bone. Cortical bone’s primary structural unit is an osteon, or haversian system. The packing density of osteons determines the mechanical strength of cortical bone. Cancellous bone fills the spaces between cortical bone surfaces with a network of honeycombed spaces, known as bony trabeculae, and contains hematopoietic elements.
In addition to the cellular components of bone, the extracellular matrix provides an important part of its structural integrity. Twenty percent of bone is water, with the dry weight consisting of 35% organic and 65% inorganic substances. The organic components, secreted by the osteoblasts, consist of collagen (principally type I collagen) and glycosaminoglycans (chondroitin sulfate, hyaluronic acid, and keratan sulfate). The inorganic matrix consists of 85% calcium phosphate, 10% calcium carbonate, 5% fluoride derivatives, and trace elements. These compounds mineralize the organic osteoid and enhance the rigidity and hardness of bone.13
Bone metabolism is regulated heavily by hormones, including parathyroid hormone (PTH), calcitonin, and vitamin D. PTH acts on osteoblasts, which leads to the release of osteoclast-stimulating factor and thereby activates the resorptive process. PTH analogues that influence this interaction have been used to treat osteoporosis.14 Calcitonin, released by parafollicular cells in response to an increased plasma calcium concentration, inhibits calcium-dependent cellular processes. Osteopenia and osteoporosis are also treated with calcitonin supplementation, with evidence of a decreased number of fractures in patients so treated.15 Vitamin D stimulates increased renal and intestinal absorption of calcium. In cases of vitamin D deficiency, children develop rickets and adults develop osteomalacia.
In addition to hormones, growth factors have a profound effect on the maturation and formation of bone. Growth factors such as BMPs, tumor growth factor (TGF), and platelet-derived growth factor (PDGF) all have the ability to induce stem cell differentiation and chemotaxis. BMPs are one of the key components of inducing bone formation by activating the transcription of genes that mediate cell migration, proliferation, and differentiation.16 TGF and PDGF are other potential osteoinductive agents, and both peptides are released in high concentrations in inflammation and after tissue injury to stimulate osteogenesis, angiogenesis, fibroblast migration, and deposition of matrix. Osteoblast proliferation and collagen synthesis are also stimulated by PDGF.17
Osteogenesis, osteoconduction, and osteoinduction are the three main properties that are desirable in bone grafts. Osteogenesis refers to the cellular component of fusion, which gives the graft the ability to form new bone. Bone marrow aspirates and fresh autografts contain these cells. Osteoconduction refers to the presence of a solid matrix for new bone formation. Autograft, allograft, tricalcium phosphate, and collagen matrix may serve as good osteoconductors. Osteoinduction refers to the process by which precursors of osteoblasts are actively induced to differentiate into mature bone-forming cells. Growth factors (BMPs and PDGF) are the largest components of osteoinduction.18
Bone Healing
There are three phases of bone healing: early inflammation, repair, and remodeling. Early inflammation occurs during the first 2 weeks and consists of hematoma formation with subsequent inflammatory cell and fibroblast infiltration. Inflammatory cytokines and growth factors then lead to vascularization and the formation of granulation tissue (procallus). Between the fourth and sixth weeks, the repair phase is characterized by continued vascular ingrowth and the secretion of osteoid and fibrocollagenous fibers. This cartilaginous callus has limited mechanical strength. Over the next several months, the organic osteoid matrix is mineralized, converting the cancellous bone to compact bone. The remodeling phase continues over months to years as the fractured bone is restored to its normal size, shape, and strength. In 6 months, adequate bone strength develops.10
Proper bone healing may be limited by a number of clinical factors. Because the healing process is dependent on the early boost in inflammatory cytokines and growth factors, anti-inflammatory agents (including steroids and nonsteroidal anti-inflammatory drugs) inhibit overall fusion rates.19,20 The presence of nicotine, not just smoking, also results in decreased healing rates owing to impaired vascularization of tissues.21 In addition, systemic factors such as radiation, diabetes, rheumatoid arthritis, and osteoporosis are all known to increase pseudarthrosis rates.10 Advanced age is also a predictor of pseudarthrosis. The ratio of stem cells present in bone marrow decreases as one ages,22 and the postmenopausal state itself is associated with increased bone resorption in women.13
Biomechanical Considerations
It is important to understand several basic biomechanical principles when planning spinal arthrodesis. Wolff’s law states that bone will form in places where stress requires its presence.23 Improper placement of rigid instrumentation, brace therapy, and bed rest may shield a bone graft from mechanical stress (stress shielding) and ultimately weaken bony fusion.24 Load sharing refers to the instrumentation or graft sharing the load with the patient’s spinal elements; load bearing refers to the instrumentation or graft bearing the entire load, removing all stress from the spinal elements. To maintain the appropriate load-bearing capacity, the 80-20 rule of Harms should be considered, which states that the anterior spinal column supports 80% of the axial load, and the remaining 20% is transmitted through the posterior column.
Assessment of Fusion
Fusion success does not always correlate with patient outcome; patients with pseudarthrosis may still have a good outcome. However, it is important to evaluate for pseudarthrosis in a patient who does not have a good outcome following a spinal arthrodesis procedure. The “gold standard” for fusion assessment is direct surgical assessment.25,26 Plain radiographs, the most common noninvasive method of evaluation, are accurate in only about two thirds of patients when compared with surgical exploration and palpation.27 Static radiographs have a positive predictive value of 76% and a negative predictive value of 54%.28 Dynamic (flexion-extension) radiographs have greater accuracy, with a positive predictive value of 70% and a negative predictive value of 86%.29
Computed tomography (CT) improves the detail with which observers can assess spinal fusion, and it is more sensitive to the detection of pseudarthrosis than static films and flexion-extension radiographs.27 Many investigators have determined that thin-cut CT scans are even better at detecting pseudarthrosis.30 In posterior lumbar interbody fusion procedures, CT has been demonstrated to be more sensitive for detecting abnormalities than plain radiographs.31,32
Technetium 99m bone scanning has been used for the evaluation of pseudarthrosis; however, one study showed that its positive predictive value was only 40% and it negative predictive value was 95%,33 suggesting that bone scanning does not reliably diagnose pseudarthrosis following lumbar fusion surgery.
Roentgen stereophotogrammetric analysis evaluates bony fusion by using radiopaque markers implanted into each vertebral level incorporated into the fusion at the time of surgery. Postoperatively, the patient undergoes computerized radiographic assessment with biplanar roentgen tubes in different positions to detect movement.34 Small trials have demonstrated the ability of this imaging modality to detect motion between adjacent vertebral bodies. In one such study, 10 patients underwent roentgen stereophotogrammetric analysis following lumbar fusion, and the findings were confirmed in all 10 patients via open exploration.35 Major drawbacks of this method of analysis are that it is invasive and not widely available.
Magnetic resonance imaging (MRI) has been of limited use in the evaluation of pseudarthrosis because of poor visualization of bone; however, MRI may add unique information to detect pseudarthrosis in specific cases that CT scanning does not.36 Other minor techniques, such as ultrasonography and polytomography, have also been used to assess bony fusion.29,37
Autograft
Autogenous bone graft is the gold standard against which other bone graft materials are compared (Table 292-1). It contains the natural combination of osteogenic, osteoconductive, and osteoinductive properties that allow optimal bone integration and fusion. Given the autogenous source, there is no additional risk of disease transmission or a deleterious host-graft reaction. Disadvantages of autograft include its limited availability, harvest site morbidity, possible additional incision, and increased operative time. Harvested autograft can consist of cancellous bone, cortical bone, or a combination of both.
Cancellous autograft contains a trabecular scaffold with matrix proteins, collagen, mineral, and bone marrow cells, creating an almost ideal environment for new bone growth. Cancellous autograft is incorporated into new bone relatively rapidly compared with larger segments of cortical autograft (e.g., fibular strut graft), which may take years to incorporate.38 As with autograft in general, there is a limited amount of cancellous autograft available to harvest, and it has a low weight-bearing capacity. Indications for cancellous autograft include the need for a nonstructural graft, such as an onlay graft or facet joint packing. It can also be used to fill a structural graft, such as an interbody cage or cortical strut graft.
Autograft can be harvested in a variety of forms, such as strut graft, tricortical graft, bicortical graft, unicortical graft, and cancellous pieces. Depending on the form of graft required, the anatomy of the patient, and the location of the primary operation, potential donor sites include the iliac crest, rib, fibula, and locally collected pieces of bone from the bony decompression. Iliac crest is the most common source for both cortical and cancellous autograft for spine surgery.39
Harvest Sites
Anterior Iliac Crest
The anterior iliac crest is well suited for procedures in which the patient is in the supine position. The anterior iliac crest, specifically the anterior superior iliac spine (ASIS), acts as an attachment site for various muscles as well as the inguinal ligament. A skin incision should be made parallel to the iliac crest and started approximately 4 cm lateral to the ASIS to avoid the lateral femoral cutaneous nerve (Fig. 292-1). Variations in the course of the lateral femoral nerve have been studied extensively in human cadavers.40,41 The nerve’s normal course is inferomedial to the ASIS; however, but it may be superolateral in up to 13% of patients, where it is within 2 cm of the ASIS 98% of the time.40–44
Posterior Iliac Crest
The posterior iliac crest is another common autograft harvest site for spine surgery, especially for posterior fusion procedures. The posterior iliac crest can be accessed through a separate incision, parallel and overlying the lateral portion of the crest, made at least 8 cm lateral to midline to avoid injury to the cluneal nerves (Fig. 292-2). In the case of posterior lumbar fusion, the dissection in the fascial plane can be performed from the midline lumbar incision laterally to access the posterior iliac crest. The attachment of the deep posterior fascia is identified and then dissected off the iliac crest. The gluteal muscle can also be dissected off the lateral aspect of the crease to expose the required amount of crest. The autograft can then be harvested with a combination of drills, osteotomes, and curets. Once inside the cancellous marrow space of the ileum, a significant amount of cancellous autograft can be obtained by harvesting in all four directions. One needs to keep in mind the medial location of the sacroiliac joint to avoid injury to it. Once the harvest is complete, the donor cavity and wound are closed as previously described.
Fibula
A fibular graft’s shape, strength, and relative ease of harvest make it well suited for use in anterior cervical arthrodesis as a structural graft. It can be harvested in both a vascularized and nonvascularized form. The former is much more technically demanding to harvest and implant and is therefore recommended in high-demand situations such as failed prior fusions, cervical arthrodesis of three or more levels, osteomyelitis, and tumor cases in which radiation has been or will be used.45
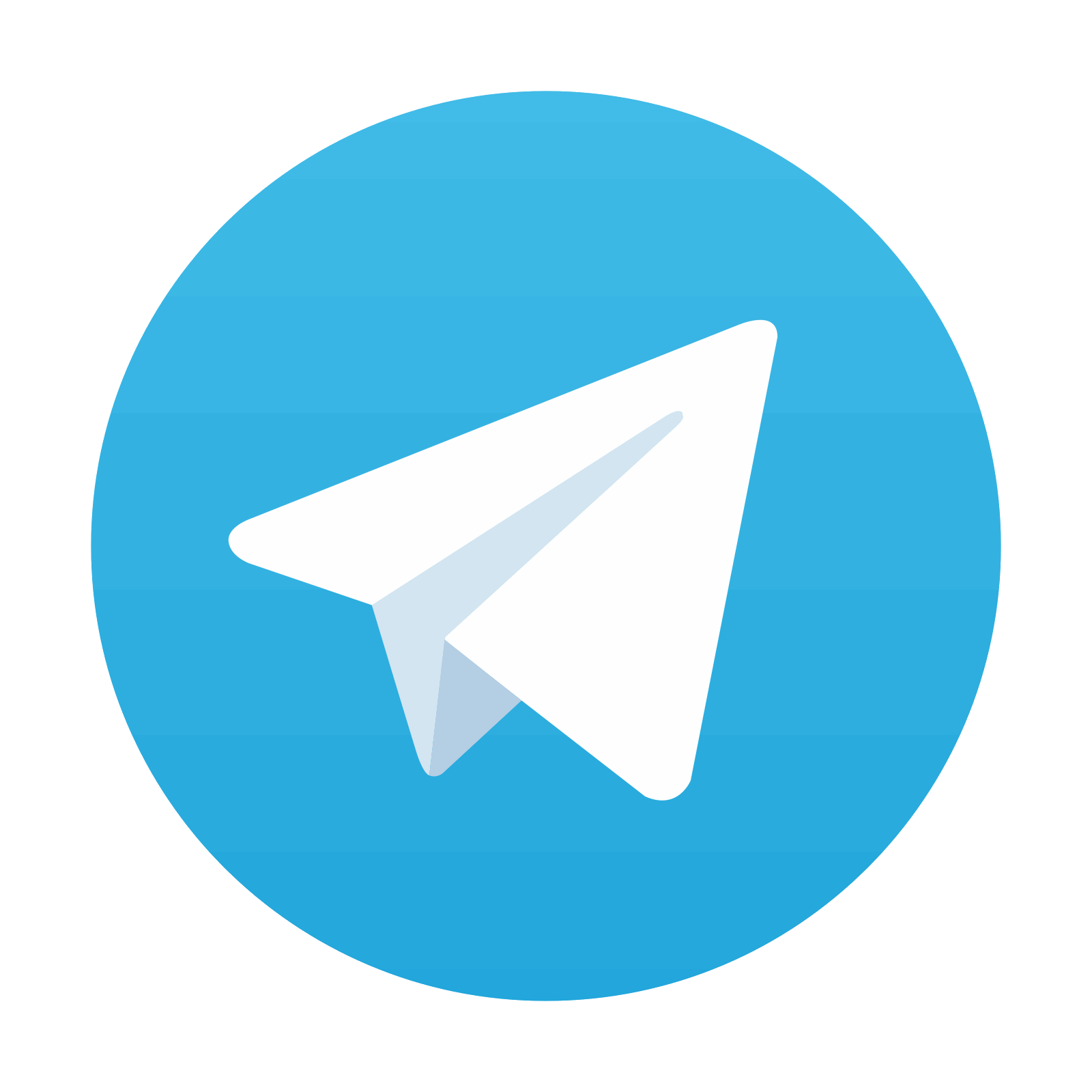
Stay updated, free articles. Join our Telegram channel

Full access? Get Clinical Tree
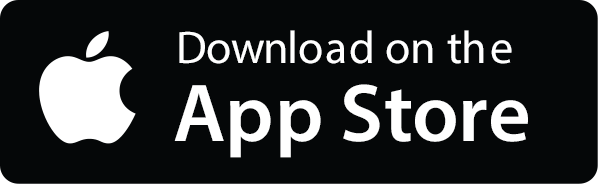
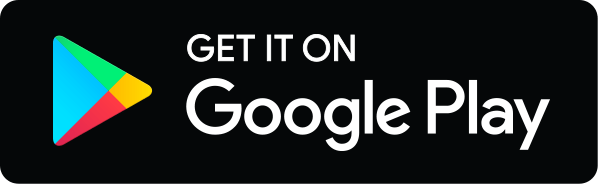