Chapter 18 [Note to readers: Botulinum toxin is available in three commercial preparations: two of botulinum toxin type A (Botox®; Allergan, Irvine, CA; and Dysport®; Ipsen, Slough, UK) and one of type B (Myobloc®; Solstice Neurosciences, Inc., Malvern, PA). Dosages discussed in this chapter refer specifically Botox, and the reader should note that these are not equivalent to those of either of the two other preparations.] Botulinum toxin is a naturally occurring neurotoxin that causes systemic muscle weakening by reversible blockade of acetylcholine release at the synaptic junction. In therapeutic applications, it is administered by intramuscular injection, and its effects are limited by dose and toxin distribution. The superficial location of the larynx makes its muscles accessible, and the clear anatomic division between adductors and abductors of the vocal fold, separated by the lamina of the cricoid cartilage, allows selective treatment of each functional group. As a result, botulinum toxin has proved useful in a variety of laryngeal disorders of inappropriate or hyper-functional muscle activity, spasmodic dysphonia most prominent among them. Botulinum neurotoxins are produced by Clostridium botulinum bacteria as protein complexes consisting of a 150-kd neurotoxin molecule and several associated nontoxin hemagglutinating and nonhemagglutinating proteins.1 The protein complex sizes formed are approximately 300 kd, 600 kd, and 900 kd (previously called M, L, and LL) depending on the strain of the organism.1 The nontoxin complex proteins appear to protect the neurotoxin from degradation and may influence the migration properties of therapeutic preparations.2–5 Seven different botulinum neurotoxin serotypes have been identified (A, B, C1, D, E, F, G),6 although only types A and B are routinely used clinically. For all serotypes, the 150 kd neurotoxin molecule is produced as a single-stranded molecule that must be cleaved or nicked by proteases into a di-chain molecule consisting of a heavy chain and light chain.7 When injected into muscles, botulinum neurotoxins inhibit the release of acetylcholine from motor neurons, resulting in a reduction in muscle contractions.6 This inhibition occurs via a multistep process that begins with the binding of the heavy chain of botulinum neurotoxin to nerve terminal receptors.8 These receptors have recently been identified as synaptic vesicle (SV) proteins—SV2 for botulinum toxin type A9,10 and synaptotagmins I and II in association with gangliosides for botulinum toxin type B.11,12 Once bound, the neurotoxin protein is internalized into nerve cells along with the synaptic vesicles.9,10 After internalization, the neurotoxin light chain is translocated across the vesicular membrane into the cytosol via a process involving the heavy chain.13 The light chain functions as a zinc-dependent endopeptidase that cleaves one or more proteins necessary for vesicular neurotransmitter release.14 Each botulinum toxin serotype cleaves at least one peptide bond on a SNARE protein (soluble N-ethyl-maleimide-sensitive factor attachment protein receptor),15 which makes up the vesicle docking and fusion apparatus required for neurotransmitter exocytosis. Botulinum toxin types A, C1, and E cleave SNAP-25 (synaptosomal membrane-associated protein, 25 kd), whereas types B, D, F, and G cleave one or more of the vesicle associated membrane proteins (VAMPs; synaptobrevins).16 Serotype C1 also cleaves syntaxin.17 The clinical effects of botulinum neurotoxins appear approximately 24 to 72 hours following injection. As acetylcholine release is decreased, neuronal sprouts develop at the neuromuscular junction and begin to release neurotransmitter.18,19 Eventually, these sprouts retract, and exocytosis is reestablished in the original nerve terminal.19 The clinical effects of botulinum neurotoxins last on the order of months, and reinjection is typically required to maintain clinical benefits. Botulinum neurotoxins not only inhibit acetylcholine release from α-motor neuron terminals, but also from γ-motor neurons.20,21 This action has been found to reduce the muscle spindle inflow to α-motoneurons, which may alter reflex muscular tone.20 Similarly, botulinum neurotoxins inhibit acetylcholine release from cholinergic autonomic fibers,22 which makes them useful for the treatment of certain conditions characterized by localized autonomic overactivity (e.g., hyperhidrosis). Botulinum neurotoxin has been found to inhibit the release of some noncholinergic transmitters in the central nervous system under experimental conditions.23 However, unlike tetanus toxin, botulinum neurotoxins are not retro-gradely transported to the central nervous system following peripheral administration and therefore do not directly influence central neurotransmitter release.24 In contrast, botulinum toxin type A appears to inhibit the evoked release of several peripheral neuropeptides that are involved in the transmission of pain. Specifically, botulinum toxin type A has been found to inhibit the depolarization-induced release of substance P from primary culture of embryonic rat dorsal root ganglia neurons,25 as well as the stimulated release of calcitonin gene-related peptide (CGRP) from cultured trigeminal ganglia neurons.26 A preclinical study of bladder pain found that botulinum toxin type A inhibited CGRP release from afferent nerve terminals and reduced pain responses.27 Botulinum toxin type A has also been found to inhibit the formalin-evoked release of glutamate from primary afferent terminals.28 It is possible that some of these nonclassical mechanisms of action contribute to the clinical effects of botulinum toxin type A in a variety of indications. The small size and proximity of the laryngeal muscles to one another place a premium on accuracy in botulinum toxin injection. Electromyographic (EMG) guidance enables the clinician to locate deep, small muscles that are impossible to palpate, and enables localization of the most electrically active areas of target muscles. EMG can help to minimize unwanted effects on neighboring muscles, as well as to maximize the benefit of each treatment by placing toxin close to its site of action at the motor end plates, allowing a smaller dose and volume to be used. We inject botulinum toxin though a 27-gauge insulated needle attached to an EMG, functioning like a monopolar electrode, in virtually all laryngeal applications. To treat the thyroarytenoid muscle, the patient is placed in a reclining position with the neck extended. A shoulder roll may be used. We find that local or intratracheal anesthesia is unnecessary in most cases, and may interfere with the EMG signal.29 It is helpful to bend the needle upward some 30 to 45 degrees, especially when injecting women, as the shorter anterior-posterior distance of the female larynx requires a more acute angle of entry under the inferior border of the thyroid cartilage (Fig. 18.1). The needle is inserted through the skin at or just off of the midline at the level of the cricothyroid membrane and advanced superiorly and laterally toward the side of the target thyroarytenoid muscle. Often, the needle enters the air column in the laryngeal lumen after traversing the cricothyroid membrane, producing a characteristic “buzz” on EMG. This tells the injector that the needle lies medial to the vocal fold, and must be directed more laterally. Crossing the endolaryngeal mucosa is irritating to the patient, however, and may provoke a cough or a swallow. By piercing the cricothyroid membrane a few millimeters to the side of the midline, the experienced injector may enter the thyroarytenoid muscle directly without first entering the airway, significantly decreasing patient discomfort. Once the needle is in an area that demonstrates crisp motor unit potentials, the patient is asked to phonate. Brisk recruitment and a full interference pattern in EMG confirms placement, and the botulinum toxin is injected. With experience, the clinician becomes able to identify the characteristic acoustic signature of the motor unit end plates, making it unnecessary to refer to the visual signal. Fig. 18.1 Thyroarytenoid muscle injection for adductor spasmodic dysphonia. (From Sulica L, Blitzer A. Botulinum toxin treatment of spasmodic dysphonia. Op Tech Otolaryngol Head Neck Surg 2004;15:76–80. Reprinted with permission.) The posterior cricoarytenoid muscle may be reached in two ways. Most commonly, the injector places his or her thumb at the posterior edge of the thyroid cartilage on the side to be injected and, using counterpressure from the other four fingers on the opposite thyroid lamina, rotates the entire larynx to expose its posterior aspect (Fig. 18.2). The needle is inserted along the lower half of the posterior edge of the thyroid cartilage, traversing the inferior constrictor, and it is advanced until it stops against the cricoid. The needle is then pulled back slightly and the patient is asked to sniff to activate the posterior cricoarytenoid to check placement. Alternately, the needle may be inserted through the cricothyroid membrane in the midline, guided across the lumen of the subglottic space (again identified by the characteristic airway “buzz”) and through the posterior lamina of the cricoid cartilage to one side or the other of midline (Fig. 18.3).30–32 An intratracheal injection of plain lidocaine helps to prevent coughing and does not affect the EMG signal, as the target muscle lies on the opposite side of the cricoid lamina. Once through the cricoid cartilage, the first electrical signal encountered on the far side represents the posterior cricoarytenoid muscle. Placement is confirmed by muscle activation during sniffing, and the botulinum toxin is injected. In our experience, this approach is most useful in younger patients in whom the cartilage has not undergone extensive calcification. Even so, fragments of cartilage often plug the needle as it crosses the cricoid, and expelling them to begin injection may require considerable force on the plunger of the syringe. Fig. 18.2 Posterior cricoarytenoid muscle injection for abductor spasmodic dysphonia via the retrocricoid approach. (From Sulica L, Blitzer A. Botulinum toxin treatment of spasmodic dysphonia, Op Tech Otolaryngol Head Neck Surg 2004;15:76–80. Reprinted with permission.) Fig. 18.3 Transcricoid injection of the posterior cricoarytenoid muscle for abductor spasmodic dysphonia. (From Sulica L, Blitzer A. Botulinum toxin treatment of spasmodic dysphonia, Op Tech Otolaryngol Head Neck Surg 2004;15:76–80. Reprinted with permission.) Alternatives to EMG-guided injection include a variety of visually guided techniques. Botulinum toxin may be administered transcutaneously, as described above, under flexible fiberoptic laryngoscopic observation.33 It may be injected transorally via a curved needle under endoscopic or mirror control,34 or via the instrument channel of a flexible fiberoptic endoscope so equipped.35 Probably only the last of these is suitable for reaching the posterior cricoarytenoid muscle. In any case, any method that enables the physician to achieve reliable and repeatable chemodenervation can be used to deliver the toxin. Subjecting the patient to a general anesthetic, as in the early days of the procedure, is no longer warranted. Spasmodic dysphonia is a type of dystonia, a chronic disorder of central motor processing characterized by task-specific, action-induced muscle spasms. Initial use of botulinum toxin for this disorder was intended to relieve this involuntary muscle activity, based on encouraging treatment results in blepharospasm and torticollis, dystonias affecting the periocular and cervical muscles, respectively. In his original description of recurrent nerve section, Dedo36 proposed that the abnormality in spasmodic dysphonia was not solely a matter of abnormal neural signal to laryngeal muscles, but also involved abnormal feedback from the larynx to the central nervous system. The well-known action-induced, task-specific nature of dystonia suggests that afferent feedback may indeed play a role in the pathophysiology of spasmodic dysphonia. Further, the phenomenon of the sensory trick—a tactile or proprioceptive maneuver that can be used to improve symptoms—suggests that the alteration of afferent signals may be therapeutically useful. Over time, the sensory trick generally loses its effectiveness. The reason for this is not known, but it appears that the central nervous system tends to eventually overcome this change in input and return to sending inappropriate signals to involved muscles. This may explain why permanent interventions have been largely unable to control symptoms permanently in various dystonias. In the case of spasmodic dysphonia, although recurrent nerve section, anterior commissure release, and other surgical measures have all produced encouraging short-term results, long-term benefit has proved difficult to achieve. The broad success of botulinum toxin as a treatment for focal dystonias, spasmodic dysphonia among them, may be due to the specificity, repeatability, and reversibility of the chemodenervation. Nerve terminal recovery from poisoning is a continuous, multiphase process, beginning practically as soon as acetylcholine release is blocked.19
Botulinum Toxin Treatment of Spasmodic Dysphonia and Other Laryngeal Disorders
Pharmacology of Botulinum Toxin
Techniques for Laryngeal Injection
Clinical Applications
Spasmodic Dysphonia
Rationale for Use
Stay updated, free articles. Join our Telegram channel

Full access? Get Clinical Tree
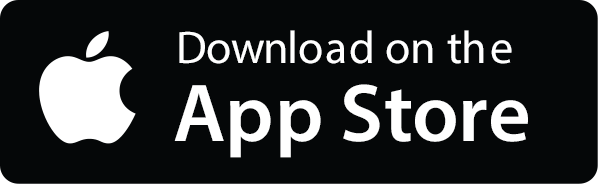
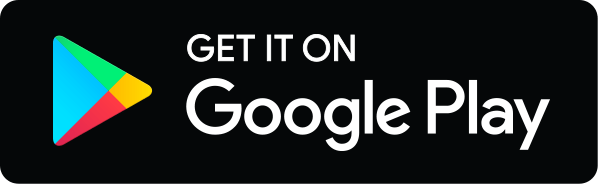