Fig. 9.1
Individual cortical potentials evoked by transcranial magnetic stimulation (TEPs) of the primary motor cortex and recorded from both hemispheres for the two groups of patients: VS patients (8) are shown in the left column, and MCS patients (5) are shown in the right column. TEPs recorded over the stimulated hemisphere are displayed in the upper row, and TEPs recorded over the contralateral hemisphere are in the lower row (Reproduced under the terms of a Creative Commons Attribution License from Ragazzoni et al. 2013 [11])
The primary benefit of assessing EEG reactivity is that it is easy to perform and can be interpreted by visual inspection from a trained EEG reviewer. However, the subjective and time-consuming nature of the analysis and inconsistency among reviewers (depending on experience and expertise) has prompted some early attempts at quantitative analysis [12–15]. Although quantitative measures provide more objectivity to the assessment of reactivity, they are vulnerable to artifactual EEG changes that might be mistaken as EEG reactivity and should be corroborated by experienced reviewers.
9.3 Short-Latency Evoked Potentials
Short-latency evoked potentials (EPs) are stimulus-locked EEG responses that occur within a brief interval from a stimulus (~10–150 ms) and are shaped by the physical characteristics of that stimulus. Together they measure the integrity of the central sensory pathways from the brainstem to the primary sensory cortices and thereby provide a crucial measure of basic cortical integrity. Somatosensory EPs (SEPs), for example, are unanimously acknowledged as reliable predictors of positive/negative outcome in acute DOC [16–18] and have been included in major practice guidelines [4, 19]. However, short-latency EPs have been the object of a limited number of studies in pDOC.
Brainstem auditory EPs are usually normal or delayed, with no differences between UWS and MCS [9, 20–22] and no prognostic implications [23, 24]. Bilateral abolition of cortical SEP components (N20 and the following) is a frequent finding in UWS following anoxia [25], at variance with posttraumatic patients [23]. Ragazzoni et al. [11] found no correlation between SEP abnormalities and clinical diagnosis. However, the presence of median nerve cortical SEPs reliably predicts long-term recovery of responsiveness in anoxic patients [25]. Visual EP latencies can also predict long-term outcome in the post-acute UWS [26, 27].
Short-latency EPs therefore provide crucial information on the structural integrity of ascending pathways and are easily employed as an extension of the neurological examination. However, their power in making the differential diagnosis between UWS and MCS is weak.
9.4 Event-Related Potentials
Event-related potentials (ERPs) represent a class of EEG responses with latencies longer than 100 ms that reflect the mass activity of neuron assemblies underpinning a series of cognitive processes. The sequence and latencies of ERP components are related to successive stages of the information-processing stream, spanning from perception to higher-order cerebral processes, such as attention, memory updating, and semantic comprehension. Importantly, the earlier components elicited in the 100–250 ms time interval (such as P1, N1, P2, N2, mismatch negativity—MMN) have been associated with the automatic sensory and perceptual processing of stimuli, operating independently from attention on an unconscious level.
Access to conscious awareness is signaled by the appearance over the central-parietal scalp of a later positive component, named P3b (or P300 for its modal latency) peaking between 300 and 500 ms, depending on the sensory modality of the eliciting stimulus [28–30]. Although not all experimental evidences support this interpretation (for a detailed discussion [31]), P3b appears at present as one of the most reliable electrophysiological marker of conscious access.
An abundance of ERP studies in pDOC have been produced in the last 20 years. Some reports concerned single or few patients or were cohort studies and are therefore of limited clinical relevance and will not be considered here. Table 9.1 summarizes the results of thirteen studies with relatively large samples of patients analyzed at the individual level and aiming at differentiating patients with UWS from those with MCS. We categorize the studies into two levels, reflecting the different complexities of ERP components analyzed. Level 1 indicates analysis of earlier components (i.e., MMN, P3a, N400) associated with preconscious cognitive functions, such as sensory memory, orienting response, or semantic processing. Level 2 refers to analysis of later components (P3b, LPC, P600) that in healthy controls reflect conscious processing.
Table 9.1
ERP diagnostic studies in UWS and MCS
Authors | ERP examined | UWS N° | Response level 1a | Response level 2b | MCS N° | Response level 1a | Response level 2b | Task |
---|---|---|---|---|---|---|---|---|
Kotchoubey et al. (2005) [68] | MMN, P3b, N400, P600 | 50 | 26 (52 %) | 12 (24 %) | 38 | 13 (34 %) | 14 (36 %) | Auditory and semantic oddball |
Perrin et al. (2006) [69] | N1, P3b | 5 | 3 (60 %) | 6 | 6 (100 %) | SON oddball | ||
Schnakers et al. (2008) [70] | N1, P3b | 8 | 0 (0 %) | 14 | 9 (64 %) | SON oddball | ||
Bekinschtein et al. (2009) [71] | MMN, P3b | 4 | 3 (75 %) | 0 (0 %) | 4 | 4 (100 %) | 3 (75 %) | Auditory local-global paradigm |
Fischer et al. (2010) [22] | MMN, P3a, P3b | 16 | 3 (19 %) | 1 (6 %) | 11 | 4 (36 %) | 1 (9 %) | Auditory and SON oddball |
Cavinato et al. (2011) [72] | N1, P3b | 11 | 5 (45 %) | 6 | 6 (100 %) | Auditory and SON oddball | ||
Boly et al. (2011) [73] | MMN | 8 | 8 (100 %) | 13 | 13 (100 %) | Auditory oddball | ||
Holler et al. (2011) [74] | MMN | 16 | 2 (12 %) | 6 | 0 (0 %) | Auditory oddball | ||
Faugeras et al. (2012) [75] | MMN, P3b | 24 | 6 (25 %) | 2 (8 %) | 28 | 9 (32 %) | 4 (14 %) | Auditory local-global paradigm |
Chennu et al. (2013) [76] | P3a, P3b | 9 | 1 (11 %) | 1 (11 %) | 12 | 3 (25 %) | 0 (0 %) | Auditory verbal oddball |
Ragazzoni et al. (2013) [11] | N1, P3b | 8 | 0 (0 %) | 5 | 0 (0 %) | Auditory oddball | ||
Steppacher et al. (2013) [77] | N400, P3b | 50 | 19 (38 %) | 32 (64 %) | 39 | 18 (46 %) | 29 (74 %) | Auditory oddball, sentences |
Rohaut et al. (2015) [78] | N400, LPC | 15 | 1 (7 %) | 1 (7 %) | 14 | 5 (36 %) | 5 (36 %) | Auditory semantic priming paradigm |
Total number | 224 | 69 (34 %) | 57 (28 %) | 196 | 69 (42 %) | 77 (43 %) |
Inspection of Table 9.1 indicates that, remarkably, an average of 1 in 3 UWS patients presented ERP responses at level 1 and/or 2, indicating that a substantial proportion of the patients could harbor some level of cognitive processing and even of conscious awareness. Unfortunately, the clinical evidence of a recovery of consciousness was available only for a few studies. Another notable fact is that no more than 43 % of MCS patients presented with ERP signs of consciousness, despite behavioral evidence of conscious awareness. Possible explanations for these false-negative results are fluctuations in arousal, lack of motivation, fatigue, difficulty in understanding the task, sensory defects, technical factors such as artifacts, and latency variability of responses. These arguments a fortiori are valid for UWS patients and suggest that ERPs underestimate the cognitive capacities of DOC patients and have poor power for differential diagnosis.
The prognostic value of ERPs has been tested mostly in terms of recovery of consciousness and neurological functions. Table 9.2 presents results from the main seven studies addressing prognosis in DOC, with follow-ups extending to 14 years. The strongest ERP predictor for recovery of consciousness is the presence of MMN, which also provided prognostic information on the functional outcome. P3b emerged as a less reliable predictor of outcome, whereas the presence of N400 was associated with a favorable neurological recovery over a long-term follow-up period. There is therefore growing evidence for the prognostic power of ERPs in pDOC.
Table 9.2
ERP prognostic studies in UWS and MCS
Authors | ERP examined | UWS N° | MCS N° | Follow-up | |
---|---|---|---|---|---|
Kotchoubey et al. (2005) [68] | MMN | 23 | 20 | 6 mos | MMN absent: 22 % of patients improved MMN present: 59 % of patients improved |
Wijnen et al. (2007) [79] | MMN | 10 | 1.5–5.2 mos | MMN amplitude and latency predict recovery from VS | |
Qin et al. (2008) [80] | MMN | 6 | 2 | 3 mos | SON MMN presence predicts recovery of consciousness |
Cavinato et al. (2009) [23] | P3b | 34 | 1 yr | P300 presence predicts recovery of consciousness | |
Luauté et al. (2010) [24] | MMN | 12 | 39 | 5 yrs | MMN not associated with favorable outcome |
Steppacher et al. (2013) [77] | N400, P3b | 50 | 39 | 2–14 yrs | N400 presence predicts favorable outcome P3b presence does not predict outcome |
Wijnen et al. (2014) [81] | N200, P3b, N350 | 10 | 1.5–5.2 mos | Presence and amplitude of N350 predict outcome | |
Total number | 145 | 100 |
9.5 Cognitive EEG Oscillations
Following the success of fMRI mental imagery paradigms to identify patients misdiagnosed as in the UWS, some success has been achieved through similar approaches in EEG. Using a variant of the tennis imagery task from fMRI [32], Goldfine et al. [33] observed significant increases in posterior alpha rhythms when one MCS patient was instructed to imagine swimming, relative to when they were instructed to rest. Furthermore, at 4-month follow-up, this patient had emerged from the MCS.
However, as noted by Goldfine et al. [33] and others [34, 35], even within healthy individuals, there is a high level of variability in the type of EEG modulation associated with mental imagery. Methods from “machine learning” have therefore been employed in order to identify the subject-specific EEG response that is consistent during periods of mental imagery, which do not rely on strict a priori predictions.
In the first example of this approach, Cruse et al. [36] asked a group of 16 UWS patients to alternately imagine moving their right hands and toes. By analyzing the spatiotemporal power values within EEG frequency bands known to be associated with motor imagery, support vector machines were able to significantly differentiate between the two types of motor imagery in 75 % of healthy individuals. Crucially, significantly greater than chance differentiation was also observed in 3 out of the 16 VS patients, indicating that these patients were following the commands and had therefore been misdiagnosed. Using the same approach, 3 out of 8 MCS patients who were incapable of following commands with their external behavior showed signs of covert command following in their EEG [37].
Despite the strengths of machine-learning methods for identifying subject-specific responses, they are also susceptible to EEG changes that are not task-relevant. For example, in a documented discussion of statistical methods [38–40], Goldfine et al. [39] highlighted the potential problems of blocked mental imagery tasks, such as those employed by Cruse et al. [36]. A blocked design overcomes criticisms of the potential automatic activation caused by task instructions [41] but also introduces potential violations of the assumptions of certain classes of statistical methods that may lead to unacceptable rates of false positives. While increasing the amount of EEG data features that are available for machine learning can in fact decrease the rate of false positives [42], the accuracy of a diagnosis made on the basis of neuroimaging-detected command following is of great importance. As has been discussed extensively elsewhere [43, 44], in all statistical analyses, a case-by-case trade-off must be drawn between an acceptable rate of false positives (i.e., evidence of command following when the patient is in fact unaware) and an acceptable rate of misses (i.e., no evidence of command following when the patient is in fact aware).
Nevertheless, in subsequent years, alternative methods that were demonstrably less susceptible to false positives have found EEG command-following abilities in a number of UWS patients (see Fig. 9.2; [34, 45]). Furthermore, Horki et al. [46] found that imagery of a user-selected sport was sufficient to produce appropriate modulations of EEG rhythms in a group of MCS patients. Oscillatory measures of command following therefore have shown promise for diagnosing awareness in pDOC. However, their prognostic value is currently unknown.
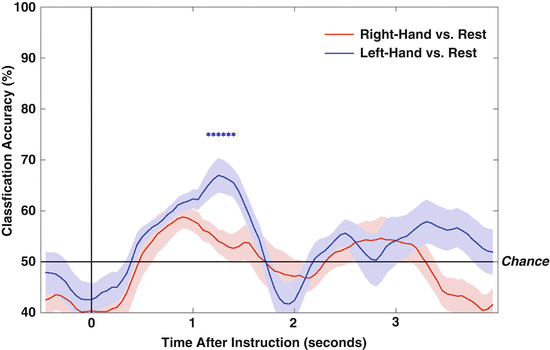
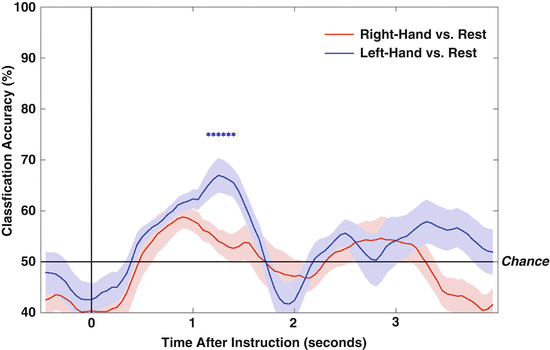
Fig. 9.2
Evidence for covert command following in a patient diagnosed as VS/UWS for more than 12-years. Data show time courses of machine-learning discrimination (classification accuracy) between EEG activity at rest and EEG activity following instructions to move the right and left hands. Stars denote time points with significantly above-chance discrimination for left hand vs. rest (p < 025) (Reproduced under the terms of a Creative Commons Attribution License from Cruse et al. 2012 [34])
9.6 Sleep EEG
The return of “wakefulness” is a diagnostic characteristic of entry into a pDOC. However, the results of the limited EEG investigations of sleep-wake cycling in pDOC patients are inconsistent with the assumption of preserved wakefulness in UWS patients [47]. This fact, however, can be employed as a diagnostic marker. Isono et al. [48] reported no quantifiable EEG changes between overnight and daytime periods in four out of twelve UWS patients. Landsness et al. [49] observed sleep-wake-like changes in the EEG of six MCS patients across 1 day, while the EEG of five UWS patients remained unchanged between periods of eye opening and eye closure. Furthermore, sleep spindles and/or REM sleep was observed in all MCS patients but was not observed in any of the VS patients.
Further emphasizing the diagnostic value of assessments of sleep architecture, De Biase et al. [50] found that patients showing evidence of both REM sleep and sleep spindles scored significantly higher on behavioral scales of awareness than those patients for whom both of these sleep markers were absent. Sleep spindles are a characteristic of non-REM sleep and reflect interactions between the thalamus and cortex. The absence of sleep spindles in behaviorally less-responsive patients is consistent with structural and functional data that find specifically impaired thalamocortical connectivity in these patients [51, 52].
It is evident that sleep EEG provides both diagnostic information and an indirect measure of neural connectivity in pDOC. Furthermore, sleep-wake assessments can identify times of day in which the patient may be more alert and therefore more likely to respond to behavioral prompts or therapies.
9.7 Putting Consciousness Theory into Practice
While many EEG approaches to identifying residual consciousness employ methods akin to conventional behavioral assessments – i.e., evidence for command following is considered evidence for consciousness – in recent years there has been a growth in theoretically based markers of awareness. With the increased availability of neuroimaging, a number of theories of consciousness have gained support and made specific predictions regarding pDOC. One set of theories considers the ability of the brain to both integrate and differentiate information to be a key mechanism for supporting human consciousness [29, 53].
One experimental approach has been to assess EEG responses to transcranial magnetic stimulation (TMS). In awake UWS patients, TMS triggers a simple, local slow response that indicates a breakdown in effective connectivity, similar to that observed in unconscious sleeping or anesthetized patients [54–56]. In contrast, in MCS patients, TMS triggers complex activations that sequentially involve distant cortical areas ipsilateral and contralateral to the site of stimulation. Casali et al. [57] quantified the complexity of EEG responses evoked by TMS with the perturbational complexity index (PCI), a measure of the amount of information integration and differentiation within the brain’s response to perturbation. Consistent with the theory linking integration and differentiation to consciousness, PCI values were consistently lower during sleep and sedation than during wakeful periods. Interestingly, PCI values also tracked the behavioral signs of awareness shown by DOC patients, with values for UWS within the bounds of sleep and anesthesia, and those for MCS between healthy wakefulness and sleep/sedation, indicating the potential diagnostic value of this index.
Repetitive TMS can also induce significant perturbations on subcortical regions, including increased dopamine release and long-lasting oscillatory effects, indicating a potential therapeutic effect [58, 59]. rTMS modulates the excitability of targeted cerebral areas alongside changes in remote cortical and subcortical regions, including the thalamus and striatum, and has been shown to modify motor, sensory, and high-level cognitive functions [60, 61]. Indeed, a 10 Hz rTMS protocol applied to a MCS patient promoted clinically significant neurobehavioral recovery [62].
Noninvasive rTMS may therefore provide a new tool for brain investigation and could identify those patients most likely to benefit from therapy. Indeed, Strafella et al. [63] found that rTMS of the motor cortex induced the release of striatal dopamine. There is mounting evidence that consciousness depends on the capacity of remote brain regions to interact through cortico-cortical and cortico-thalamo-cortical connections [51]. The central thalamus and cortex are closely linked through both direct connections and indirect connections through the frontal cortical striato-pallidal-thalamocortical loops. Modulations of striatal activity by cortical rTMS may therefore help strengthen these pathways and restore awareness.
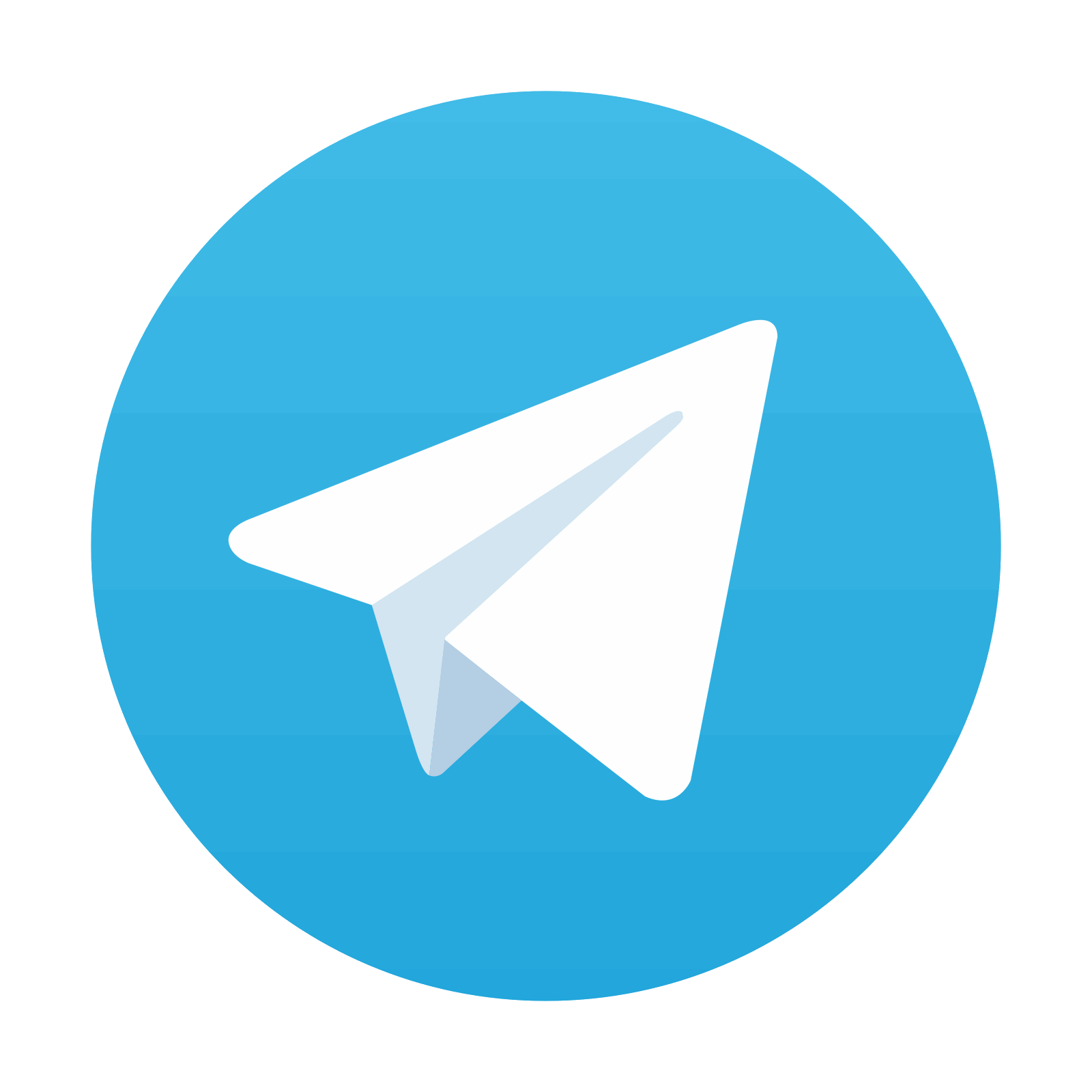
Stay updated, free articles. Join our Telegram channel

Full access? Get Clinical Tree
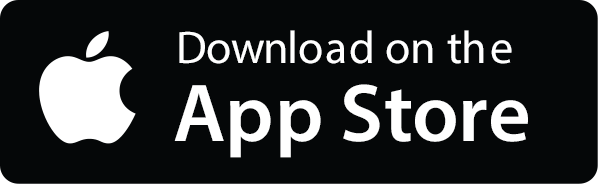
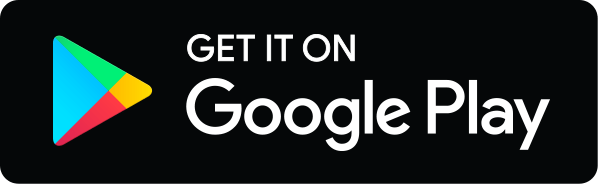