(1)
The Jerold B. Katz Professor of Neurology and Neuroscience, Feil Family Brain and Mind Research Institute, Weill Cornell Medical College, New York, NY 10065, USA
Abstract
This chapter reviews the mesocircuit hypothesis for the recovery of consciousness after severe brain injuries. Building up from a single premise that multifocal brain injuries typically produce widespread neuronal death and dysfunction, the mesocircuit model proposes a lawful circuit-level mechanism on the basis of known anatomical and physiological specializations of the corticothalamic system. The hypothesis is deeply generative of testable predictions at the mesocircuit and cellular level across multiple cerebral structures. Recent tests of some of these predictions are discussed as well as next steps in evaluating theoretical implications of the mesocircuit model.
Keywords
MesocircuitCentral thalamus Arousal systemsEEGDeafferentationBrain injury15.1 The Mesocircuit Hypothesis for Recovery of Consciousness After Severe Brain Injuries
Severe brain injuries impair consciousness in many different contexts in which bi-hemispheric cerebral function may be altered by enduring loss or dysfunction of many neuronal populations (see Posner et al. [1] for a comprehensive review). Recovery of consciousness following such brain injuries has been proposed to result from restoration of activity across a defined mesocircuit within the anterior forebrain that is particularly vulnerable to the effects of multifocal structural or functional deafferentation [2–4]. Unstable levels of behavioral responsiveness associated with initial recovery of consciousness after coma in the context of behavioral signs of consciousness emerging in the minimally conscious state (MCS) and in patients recovering just past the border of MCS in confusional state can be understood in the context of dynamical shifts in patterns of activity across this anterior forebrain mesocircuit [5].
In brief, the mesocircuit hypothesis (Fig. 15.1) starts with the basic observation that all severe brain injuries share common pathological substrates of widespread functional or structural deafferentation of neocortical, striatal, and thalamic neurons that maximally impacts survival or function of central thalamic neurons [6–8]. The primary consequence of broad deafferentation following diffuse multifocal patterns of corticothalamic disconnection is disfacilitation, a passive hyperpolarization of neurons that reduces their firing rates; experimental studies demonstrate that powerful consequences on background firing rates occur even with disfacilitation producing only modest reductions measured in cerebral blood flow [9]. A marked reduction of global brain synaptic background activity results from the loss of the primarily long-range connections across the forebrain which are dominantly excitatory projections to neocortical, striatal, and thalamic neurons (Fig. 15.1A). The overall impact of widespread disfacilitation is a sharp reduction in cerebral metabolic rate which is considered the common denominator in all disturbances of consciousness following severe brain injuries. The broad reduction of neuronal activity across the mesocircuit is predicted to be specifically graded by a result of two core interacting effects. Most important and general is the role of deafferentation of the neurons of the central thalamus. These central thalamic neurons are unique in providing, in the aggregate, the shortest point-to-point connections across the entire corticothalamic system and as consequence suffer the greatest impact of multifocal deafferentation [8, 10]. Moreover, their functional role in the wakeful state is in adjusting overall levels of activity in the frontostriatal systems to respond to challenges of increasing cognitive load, stress, or fatigue (see Schiff [10] for comprehensive review). Thus, reduction of the output of these neurons is anticipated to have a negative synergistic effect across the corticothalamic system, as powerful modulatory excitatory effects across the cortex and striatum are withdrawn. A second interacting mechanism is the anticipated complete shutdown of firing of the medium spiny neurons (MSNs) of the striatum as a result of their high-threshold UP state and dependence on high-frequency inputs from corticostriatal and thalamostriatal afferents to maintain their firing rates [11]. Shutdown of the striatal MSNs produces disinhibition of the globus pallidus interna and would add subsequent active inhibition of the already disfacilitated central thalamus and possibly a synergistic effect of inhibition of outflow of pedunculopontine nucleus input to the central thalamus further hyperpolarizing these thalamic neurons [3, 12].
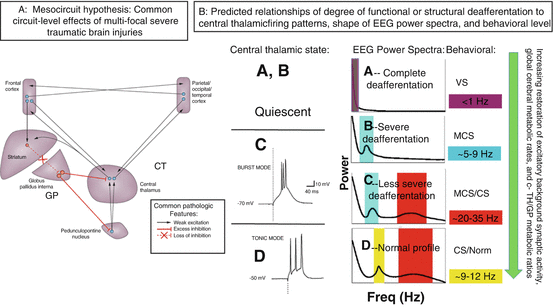
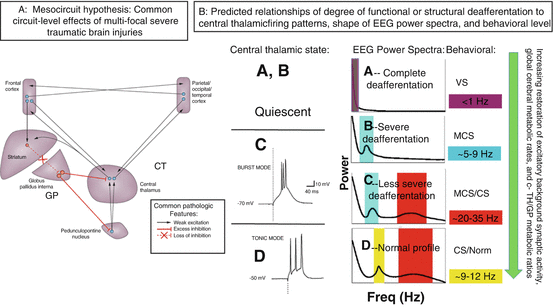
Fig. 15.1
(Panel a) The mesocircuit hypothesis [2–5, 15, 16] proposes that, based on known pathologic changes after trauma, hypoxia, or multifocal ischemia, reduction of thalamocortical and thalamostriatal outflow following deafferentation and loss of neurons in central thalamus withdraws important afferent drive to frontal cortex and the medium spiny neurons (MSNs) of the striatum (Adapted from Giacino et al. [12]). Impact across the frontal cortical systems is maximal because of the density of central thalamic projections and a contribution from a failure of MSNs to reach firing threshold because of their requirement for high levels of synaptic background activity [11]. (Panel b) Relationship of degree of deafferentation in disorders of consciousness after severe brain injuries, central thalamic state, and electroencephalogram patterns [17]. At varying levels of functional or structural deafferentation, changes in central thalamic firing patterns are expected to lawfully appear and associate with neocortical dynamics measured in the EEG: quiescence in the setting of very severe structural or functional deafferentation (A, B); bursting (C) in setting of significant deafferentation [18], and tonic firing mode with recovery (D). Expected changes in EEG spectra are linked to increases in overall level of excitatory drive to neocortical neurons with increasing central thalamic activity and overall shifts of cerebral metabolism that are predicted to arise in a sequence in recovery and to grade with levels of structural or functional deafferentation and behavioral outcome (green arrow running vertically down). At the extreme of complete functional deafferentation of neocortical neurons, very low average membrane potentials (AMPs) of neocortical neurons of ~−70 mV are measured and a concentration of spectral power <1 Hz is expected [19] as shown in the “A” spectrum. Patients with very severe cerebral deafferentation who remain in vegetative state may show this pattern (see, e.g., Fig. 15.2a). With sufficient afferent input to place the AMP in the range ~−60–65 mV, spontaneous oscillations of Layer V pyramidal cells [20] produce ~5–9 Hz oscillations. This mechanism is proposed to account for a ~7 Hz oscillation that dominates the resting activity of the EEG in the “B” spectrum as observed in some MCS patients [5]. In both the A and B setting, the central thalamus is predicted to be quiescent (direct measurements of central regions of 6 thalami in 3 MCS patients from our database support this expectation, Giacino et al. [21, 22]). In the setting of more normal levels of global background synaptic activity and cerebral metabolism, more deafferented regions of the thalamus are expected to burst and produce co-existing ~5–9 and ~15–40 Hz EEG oscillations local to cortical projection regions [18, 23], seen in the “C”-type spectrum. Normal EEG background activity (“D”) is by contrast, globally dominated by 8–12 Hz and features within the ~15–40 Hz range [24]. The mesocircuit hypothesis predicts that more functionally deafferented neurons will be concentrated in frontocentral regions; this specific spatial localization prediction has been verified for a set of zolpidem responsive subjects with “B”-type spectra [5] and traumatic brain injury patients with spontaneous recovery of communication and higher cognition [25]
There is further a direct relationship between graded reduction of activity across the anterior forebrain mesocircuit and the existing and compelling evidence for a graded relationship of frontoparietal activity in outcomes of severe brain injuries, particularly resting activity within the posterior medial complex of the parietal cortex [13]. Fernandez-Espejo et al. [14] reported that diagnostic categories of disorders of consciousness can be stratified by diffusion tensor imaging (DTI) of connections between the thalamus and the posterior medial complex (PMC). Anatomical and physiological studies show that the central thalamus and the posterior medial cortical regions have strong functional connections. Track-tracing studies in nonhuman primates demonstrate that the anterior intralaminar nuclei provide a substrate through their broad innervations of all components of the posterior medial complex (PMC) of the parietal lobe [26]. The human central lateral nucleus and surrounding paralaminar regions receive the heaviest innervations of both brainstem and basal forebrain cholinergic systems (Heckers et al. [27]), and studies of pharmacological stimulation during human anesthesia demonstrate that brief recovery of consciousness is associated with selective significant increases regional blood flow to thalamus and precuneus compared with wakeful baseline when administering a systemic cholinergic agonist [28].
15.2 Bulk Activation of Anterior Forebrain Mesocircuit by Pharmacological and Other Interventions
The impact of several pharmacologic interventions and central thalamic deep brain stimulation on some patients with severe brain injuries can be explained as a result of bulk activation of the anterior forebrain mesocircuit [29]. A variety of specific pharmacological interventions are known to be effective in improving behavioral responsiveness in some severely brain-injured patients, including the dopaminergic agonists such as bromocriptine, apomorphine, L-Dopa, and amantadine [29, 30]. Dopaminergic tone is the other dominant modulator of the output of the medium spiny neurons, and the mesocircuit model would predict activation of the anterior forebrain in cases where sufficient afferent input remained capable of turning on the MSNs once dopamine levels were restored within a deafferented striatum. Direct modulation of mesial frontal cortical neurons by dopaminergic agonists alone would likely be insufficient as an explanation as neuromodulators in general do not drive firing rates. Release of the glutamatergic afferents of the central thalamus, however, by release of the active pallidal inhibition is predicted to restore anterior forebrain activity across the loop connections of the frontal cortex, striatum, pallidum, and central thalamus.
Amantadine has been shown to be a consistently effective medication in improving behavioral function in disorders of consciousness [22], and its strong and broad effects likely reflect a variety of actions [29]. Perhaps most important is its role as an NMDA antagonist, like ketamine which produces broad cortical excitation [2]. Amantadine may produce bulk activation of the mesocircuit via a combination of NMDA antagonism and dopaminergic as well as noradrenergic agonism [29]. Schnakers et al. [30] demonstrated that effective amantadine response in a minimally conscious state patient reliably associated with broad medial and lateral frontal cortical increase in cerebral metabolism. The paradoxical phenomenon of behavioral facilitation observed in some severely brain patients with administration of the sedative agent zolpidem (a GABA-A alpha 1 positive allosteric modulator) may reflect a similar multiplicity of sites of action across the mesocircuit including a critical effect of suppressing active pallidal inhibition [4], direct modulation of cortical and striatal populations, and even a possible role for ephaptic excitation of axons (see Williams et al. [5] for detailed discussion of these mechanisms and their relationship mesocircuit components).
Bulk activation of the anterior forebrain mesocircuit can also be achieved via direct activation of the glutamatergic afferents of the central thalamus with consequent behavioral improvements in severe brain injury [31]. In a single-subject study activation of midline central regions measured by evoked responses obtained from DBS electrodes showed that effective contacts generated bilateral patterns of activation of the electroencephalogram over frontocentral and frontotemporal electrodes, EEG [31]. Of note, evoked cerebral activity from the human central thalamus also showed localized activation of electrodes over the medial parietal cortical regions in this subject supporting the strong functional connectivity between the anterior forebrain mesocircuit and the medial parietal cortex through the central thalamus discussed above (see Laureys and Schiff [13] for further discussion).
15.3 Testing Specific Model Predictions
Recent studies have tested and confirmed several predictions of the mesocircuit hypothesis related to structural injury patterns, cerebral metabolic profiles, and patterns of resting electrophysiological activity in the severely injured brain. In a series of studies, Lutkenhoff et al. [32, 33] elegantly demonstrated that tissue atrophy within the basal ganglia and thalamus systematically associated with clinical measures of awareness and wakefulness providing evidence that the anatomical integrity of these key subcortical components of the anterior forebrain mesocircuit alone is a sensitive marker for behavioral outcome. Physiological studies have also addressed more specific predictions of the mesocircuit hypothesis. Drover et al. proposed an “ABCD” classification scheme that organizes the relationship of the degree of deafferentation in disorders of consciousness after severe brain injuries, central thalamic state, and expected electroencephalogram patterns as shown in Fig. 15.1B [17]. The mesocircuit hypothesis makes several predictions that can be precisely framed using this ABCD model.
The ABCD model is a coarse graded linear organization of degree of cerebral deafferentation and expected effect on neocortical neurons measured in the EEG. One key prediction is that graded functional deafferentation across the neocortex should consistently associate with a anterior-posterior gradient of greater to lesser functional deafferentation producing a lawful progression of patterns of neuronal firing rates reflected in the shape of the EEG power spectrum that strictly covary with regional and global patterns of cortical metabolic activity from most severely downregulated to fully recovered (Fig. 15.1). Beginning with the extreme case of nearly complete cortical deafferentation, the conditions of the experimental slab preparation [19] are approximated where neocortical neurons remain with an average membrane potential of ~−70 mV and population activity reflected in the EEG power spectrum is restricted to frequencies of ~1 Hz. Patients with very severe anoxic brain injuries in vegetative state may demonstrate these “A-type” features of EEG power spectra (See Fig. 15.2A and Schiff et al. [16]). In the healthy intact cerebral cortex without deafferentation, the resting average membrane potential is ~−55 mV [34], and population activity reflected in EEG power spectra show a peak in the alpha (8–12 Hz) along with variable number of peaks in higher frequency ranges (“D-type” spectra, see Fig. 15.2B).
