, Jitka Annen1, Georgios Antonopoulos1, Enrico Amico1, Carlo Cavaliere1 and Steven Laureys1
(1)
Coma Science Group, Neurology Department, Cyclotron Research Center, University of Liège, Liège, Belgium
Abstract
At present, the global hallmark of impaired consciousness appears to be a reduced metabolism in a widespread frontoparietal network and a multifaceted dysfunctional connectivity architecture characterized by intra-/internetwork altered connectivity, both in the sense of decrease and increase.
However, despite ongoing efforts, the mechanisms underlying the emergence of consciousness are not thoroughly understood. Moreover, recent studies highlighted difficulty in forming a diagnosis in patients with disorders of consciousness (DOC) based only on behavioral assessments.
We here aim at providing an overview of the insights that functional and structural neuroimaging techniques can provide in comprehending the mechanism underlying consciousness and assessing patients with DOC.
Keywords
DOCFunctional neuroimagingPETResting-state fMRIDTI5.1 Introduction
Back in the 1950s, improved health care in the intensive care unit allowed patients to sustain heart function and systemic circulation after traumatic and nontraumatic brain injuries. Ever since, some surviving patients were found to suffer from altered states of consciousness that were never encountered before [1]. These were mainly characterized by prolonged states of dissociation of the two components of consciousness: wakefulness and awareness [2]. Wakefulness, or arousal, refers to the level of alertness which is necessary but not sufficient to sustain consciousness [2].
Awareness refers to the ability to live experiences of any kind, comprising subjective experiences such as sensations, thoughts, emotions, memory, imagination, and other major psychological processes [3]. Awareness has been further subdivided into internal awareness (i.e., awareness of self) and external awareness (i.e., awareness of the environment) [4]. Wakefulness can be clinically detected by opened eyes. On the other hand, there is no singular marker of awareness, yet its presence can be clinically deduced from a range of behaviors (e.g., responses to command, visual pursuit) which indicate that an individual can perceive self and surroundings [5].
Coma is a state characterized by the inability to support wakefulness and awareness of the surroundings and of the self, lasting no longer than a few weeks. Patients who awaken (i.e., open their eyes) but remain unresponsive to any external stimulation are considered to be in a vegetative state (VS) [6], a condition now also coined as unresponsive wakefulness syndrome (UWS) [7]. It was only in 2002 that the medical community recognized another entity which characterized patients showing fluctuating yet reproducible behavioral signs of consciousness; these patients were said to be in a minimally conscious state (MCS) [8]. The heterogeneity of MCS has been recently recognized, and it has been proposed to subcategorize this group based on the level of complexity of the observed behavioral responses into MCS minus and MCS plus, of which the latter shows the ability to understand language and therefore to follow simple commands [9, 10]. Patients are considered to emerge from an MCS (EMCS) once reliable communication and object use in a functional manner are restored [8]. Patients in a locked-in syndrome (LIS) are conscious but have lost voluntary motor control, except for small eye movements, making it possible for them to answer yes-no questions. More rarely, LIS patients can lose the control of all their voluntary muscles, including extrinsic eye muscles (complete LIS) [11]. In these patients, the only evidence for preserved consciousness may be their ability to communicate via assisting technologies (i.e., fMRI, EEG, or evoked potentials). As such, the term “functional LIS” has been recently proposed to describe those patients with a dissociation between extreme behavioral motor impairment and the identified preserved higher cognitive abilities only detectable by functional imaging techniques [9].
Despite considerable research, disorders of consciousness (DOC) remain poorly understood in regard to their neural basis, clinical recognition, and long-term outcome [12–14]. Indeed, even if the border zone between patients in a VS/UWS and MCS is at present well delimited, bedside assessment of consciousness is intrinsically gated by behavioral responsiveness. Numerous studies highlight the difficulty in forming a diagnosis for DOC patients when this is established merely on behavioral assessments [15–17]. It is now increasingly recognized that the absence of purposeful behaviors cannot be taken as a definitive proof of the absence of consciousness, that is, the lack of responsiveness does not necessarily imply lack of awareness. Indeed, neurophysiological and functional neuroimaging data indicate that a subset of behaviorally unresponsive patients retain patterns of cerebral processing similar to those observed in conscious patients (functional LIS) [15]. This knowledge has led to the search for nonclinical assessment techniques in order to better understand brain function in these patients and to overcome the limits of behavioral assessment in the detection of possible retained consciousness in unresponsive patients.
We here aim at providing an updated overview of the application and contribution of structural and functional neuroimaging techniques for the understanding of brain functions underlying consciousness and the assessment of patients with DOC. We will first discuss the information we obtain about the brain’s metabolic activity (with positron emission tomography-PET) and its hemodinamic functions (with functional magnetic resonance imaging – fMRI). We will focus on resting-state fMRI studies, i.e. in the absence of external or internal stimuli. We will then discuss the information we obtain about the brain’s structural properties (with conventional MRI and diffusion-weighted MRI). To our mind, an integration of functional and structural neuroimaging techniques, and more generally of the different technical modalities, is important to gain a holistic vision of the underlying pathophysiology of disorders of consciousness in general and to promote single-patient management in particular.
5.2 Functional Neuroimaging in DOC
Studies using conventional brain structural imaging have shown highly variable and heterogeneous findings in patients with DOC, suggesting that awareness cannot be strictly related to an anatomic region. This induced researchers to adopt alternative neuroimaging techniques in order to investigate the role of brain function in the alterations of consciousness [18].
5.2.1 Positron Emission Tomography
Positron emission tomography (PET) is one of the first functional neuroimaging techniques used to investigate brain function in patients with DOC. It assesses brain activity and function by recording the emission of positrons from radioactively labeled molecules. When the chosen molecule is 18fluorodeoxyglucose (FDG), the concentration of tracer indicates glucose uptake, indirectly representing cerebral functioning, whereas the H2 15O-radiolabeled PET, reflecting blood flow, is usually used to detect activation during active and passive paradigms [19].
In comatose patients, PET studies show a reduced brain metabolism up to 50–70 % of normal range [1]. After recovery from postanoxic coma, cerebral metabolic rates for glucose showed no drastic recovery, exhibiting glucose rates of only up to 75 % of the normal values [20]. Furthermore, no established relation between cerebral metabolic rates of glucose or oxygen and patient outcome has been found [20]. In addition, a global decrease in cerebral metabolism is not unique for coma, as it can be associated with several different situations with temporary loss of consciousness such as deep sleep [21], general anesthesia [22], and Cotard’s syndrome [23].
VS/UWS patients are characterized by a reduced global metabolism as compared with healthy subjects. However, recovery of consciousness did not necessarily coincide with resumption of global metabolic activity [17]. This observation suggested that awareness is not related to global brain metabolism [24], or at least the association between global brain metabolism and awareness is not absolute. Resting-state voxel-based PET studies have indeed demonstrated that impairment of awareness was related to decreased glucose uptake in specific brain areas, consisting of a large-scale frontoparietal network encompassing the polymodal associative cortices [25] (Fig. 5.1). In addition, the connections of this network with thalamic nuclei seem also important as suggested by the recovery of thalamocortical activity in a VS/UWS patient who had subsequently recovered consciousness [26]. Moreover, PET studies using passive auditory and noxious stimulation showed a particular disconnection between the primary sensory areas and these higher-order associative cortices [27], which are thought to be required for conscious perception [2]. In simple words, in VS/UWS, any stimulus (auditory, tactile, sensory, etc.) can reach the primary sensory cortex; however, it cannot be processed by the associative cortices which have been proposed to be necessary for conscious perception. As expected regarding the clinical condition of MCS patients, they show a partial metabolic preservation of this large-scale associative frontoparietal network [28]. For example, passive noxious stimuli have elicited the activation of associative areas related to pain processing in MCS patients similarly as in normal controls, suggesting a potential pain perception capacity in this patient category [19].
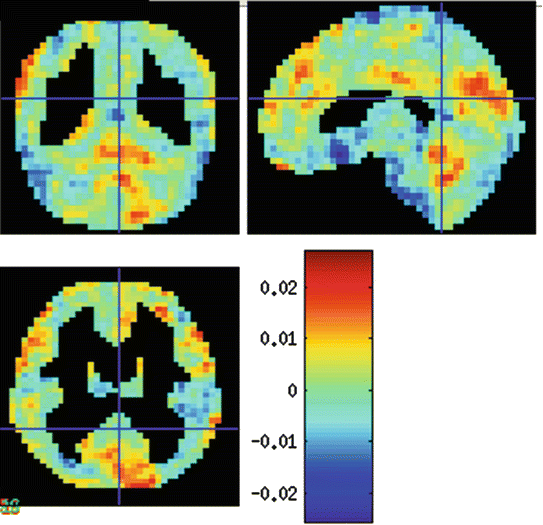
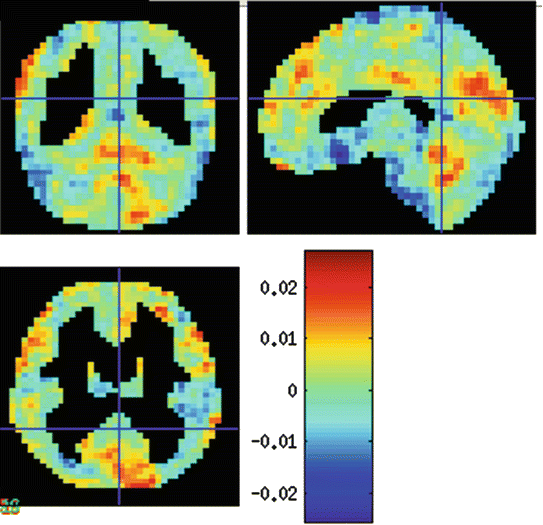
Fig. 5.1
A PET probability map of the hypometabolic areas (in red) found in VS/UWS compared to healthy controls
The abovementioned studies have suggested the importance of the associative cortices, rather than the primary sensory ones, in the emergence of consciousness. The widespread frontoparietal network encompassing associative cortices, commonly impaired during altered states of consciousness, has been recently functionally subdivided into two different networks: the extrinsic awareness network and intrinsic awareness network [29]. The extrinsic network, or executive control network (ECN), encompasses the lateral frontoparietal regions and is active during attention-demanding task. The intrinsic network, also called default mode network (DMN), encompasses the midline frontoparietal regions (anterior cingulate/mesial prefrontal cortex and posterior cingulate/precuneus) and is active during rest [30]. More specifically, several event-related fMRI studies have demonstrated the ECN and the DMN are linked to external awareness and internal awareness, respectively. For example, by giving somatosensory stimuli to subjects, it has been shown that at a certain intensity, one could perceive the stimulus only when the external awareness network (ECN) was activated. Conversely, a stimulus at the same intensity could not be consciously perceived when the internal awareness network (DMN) was activated [31]. Furthermore, a number of autoreferential stimuli such as the own name and own face pointed to the critical role of the midline structures (DMN) for self or internal awareness [32–35].
The key role of these two networks in the emergence of awareness has been further supported by a recent study on DOC patients in comparison with healthy controls showing metabolic impairment of the DMN and ECN in VS/UWS patients, preserved metabolism of the ECN in MCS patients, and a partial metabolic recovery of both the DMN and ECN only in EMCS [36]. These latter findings suggested that the (partial) metabolic recovery of both networks might be a feature of the emergence from MCS.
In terms of diagnostic accuracy, cerebral metabolism has shown to be able to specifically and reliable differentiate VS/UWS from LIS patients and healthy controls [37]. PET investigation of brain function in MCS has been in accordance with the clinical subcategorization into MCS plus and MCS minus. As expected, patients in MCS plus exhibited preserved metabolism in language comprehension-related areas compared with patients in MCS minus [10]. Furthermore, a recent clinical investigation of the diagnostic accuracy of multimodal neuroimaging methods in disorders of consciousness suggested that cerebral PET could be used to complement bedside examinations and predict long-term recovery of patients in VS/UWS. Indeed, PET was employed to correctly predict outcome in 75 of 102 patients, while active-task fMRI predicted the outcome in 36 of 65 patients [38].
5.2.2 Functional Magnetic Resonance Imaging (fMRI)
fMRI relies on the natural paramagnetic properties of deoxygenated hemoglobin to infer changes of blood oxygenation in the brain associated with neural activity (blood oxygenated-level dependent (BOLD) signal). As fMRI does not require the use of X-rays or radiotracer injection, and for its superior spatial and temporal resolution in comparison with PET, it is being increasingly used for detecting brain activity. In the last decades, active-task fMRI has been largely used in patients with DOC to detect brain activity related to residual cognition and awareness. In some cases, it was even employed to establish a two-way communication without requiring any external output, a so-called brain–computer interface [17]. However, active-task fMRI paradigms can suffer from false-negative results because absent command-related brain activation does not allow to infer that awareness is not present [39]. To overcome these limits, resting-state fMRI has been used increasingly.
Resting-state fMRI is particularly suitable for patients with DOC or generally in noncompliant patients as it surpasses the need for patients’ participation (the subject is only asked to be still in the scanner without thinking of anything), and it does not require sophisticated experimental setup to administer external stimuli. Resting-state fMRI is used to investigate the spontaneous temporal coherence in BOLD fluctuations related to the amount of synchronized neural activity (i.e., functional connectivity) existing between different brain locations [40]. Brain regions showing the same time course are believed to be functionally connected and form the so-called resting-state functional networks.
Several spatially consistent networks have been identified, such as the auditory network, the visual network, the salience network, and the cerebellum network [41]. However, the DMN has been investigated the most intensively by the scientific community. Given that the DMN seems to be linked to internally oriented cognitive content, such as mind wandering and autobiographical memory recall [4, 42, 43], it is not surprising that it has raised interest in the field of consciousness.
Recent resting-state fMRI studies have shown a breakdown of functional connectivity within the DMN decreasing with the degree of severity of DOC [44]. The reduced connectivity affected mostly the precuneus, a main node of the DMN considered to be a critical hub for consciousness [1]. Similarly, decreases of DMN connectivity were observed in healthy controls during different states of altered consciousness, like sedation, general anesthesia [45], and deep sleep [46].
Recently, more resting-state networks have been investigated in DOC, such as the bilateral executive control network (ECN), the salience, the sensorimotor, the auditory, the visual, and the cerebellar network. It was found that besides the DMN, also the bilateral external awareness network (ECN) and the auditory system were significantly less identifiable (in terms of spatial and neural properties) in DOC patients compared to healthy controls showing consciousness-level-dependent decreases in functional connectivity across the spectrum of DOC (Fig. 5.2). Furthermore, machine learning classification was used to identify if these networks showed neuronal activity or not, employed to separate healthy controls from patients in DOC with 85 % accuracy [47].
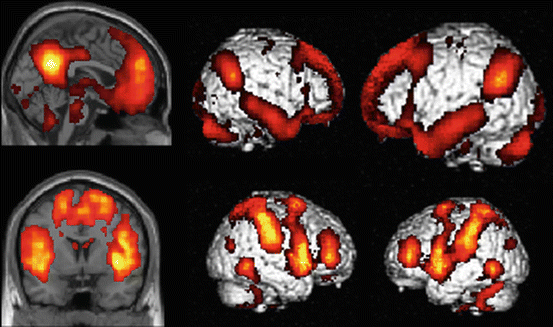
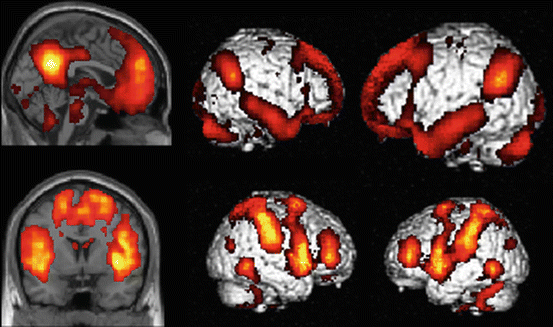
Fig. 5.2
Two anticorrelated resting-state functional networks: default mode network (top line) and executive control network (bottom line). Statistical maps (in red) are superimposed on biplanar and triplanar anatomical slices
Along with the reduced intranetwork DMN connectivity reported in patients with DOC, an increased connectivity in structures of the limbic system was recently reported that was stronger in VS/UWS than in MCS patients [48]. This hyperconnectivity has been thought to reflect the persistent engagement of residual neural activity in self-reinforcing loops which may impair the normal connectivity pattern [48]. Taking this into account, we have reasons to believe that hypoconnectivity might be only one aspect of a possibly multifaceted and more complex dysfunctional architecture of brain connectivity in altered states of consciousness, not being necessarily the global hallmark of these conditions.
Although these findings show that part of the resting-state brain activity may be related to mental activity and cognition, they appear in contrast with other studies showing the persistence of DMN functional connectivity even during loss of consciousness due to general anesthesia and in VS/UWS patients [49–51]. Although it is known that a small percentage of patients behaviorally in VS/UWS show a residual cognitive activity, this is very unlikely to happen during anesthesia-induced unconsciousness if DMN functional connectivity was solely related to consciousness. These findings suggested that resting-state brain activity might actually have a twofold meaning. An aspect of this activity reflects a fundamental intrinsic property of the brainʼs anatomical organization that seems to transcend the levels of consciousness, i.e., it is generated by the circulation of spontaneous neural activity along fixed anatomical architecture. The other one seems to be related to cognitive processes that actually characterize the conscious state [52].
A recent study on three monkeys during different levels of consciousness attempted to disentangle these two components and identify the resting-state brain pattern strictly related to consciousness. During the unconscious state, they found resting-state brain activity characterized by few functional connectivity patterns similar to the brain structure and the lack of anticorrelation patterns. Conversely, wakefulness was characterized by the sequential exploration of a richer repertoire of functional connectivity patterns, often dissimilar to anatomical structure, and the presence of anticorrelation between DMN and ECN, suggesting the importance of anticorrelation for the emergence of consciousness. Indeed, since the early studies of resting state, the DMN and the ECN have been shown to be functionally anticorrelated, meaning that when regions belonging to one network show above baseline activity, the regions belonging to the other network are active below their baseline and vice versa [30, 53]. In other words, there is a dynamic interaction between the two functional networks (Fig. 5.2). Such anticorrelation patterns have been observed as well in active-task fMRI studies on motor performance and perceptual discrimination [31], as mentioned above.
More recently, the characteristics of this resting-state activity have been investigated more thoroughly, and it was found that DMN and ECN naturally switch with a mean frequency of 0.05 Hz (range 0.01–0.1 Hz) [4]. Interestingly, this frequency is similar to that of behavioral correlates of external and internal awareness [4]. Activation of DMN was linearly linked to the intensity of internal awareness, whereas activation of ECN was linearly associated with intensity of external awareness [4], suggesting that anticorrelated patterns are functionally significant for mentation and cognition.
However, it should be mentioned the fMRI anticorrelation has been a subject of important debate in literature. It has been argued, for example, that the anticorrelated patterns could arise from the preprocessing procedure when the brain’s global activity [54] is regressed out. Nevertheless, as mentioned above, evidence for a physiological origin of the observed anticorrelation is increasing [30, 52, 55], as reduced anticorrelation of these two networks is observed in unconscious states such as anesthesia [45], deep sleep [56], and VS/UWS [50].
Taken together, all the above findings suggest a complex and multifaceted alteration of functional connectivity impaired consciousness, characterized by adapted intra-/internetwork connectivity (both in the sense of decrease and increase).
Unfortunately, fMRI is not suitable to every patient. Its use is virtually impossible for those patients carrying noncompatible MRI devices (pacemakers, metallic implants, etc.), often present in an intensive care setting. Apart from the known contraindication for MRI, the clinical applicability of resting-state fMRI is further limited by several practical issues. For example, patients that show prescan motion activity might need to be anesthetized during the scan. In this case, anesthesia will affect the acquired data which needs to be accounted for during data processing. Another concern is related to the severe brain deformation often observed in these statistical injured patients, which can influence spatial normalization, an essential step for group comparisons. The latter has been partially tackled in previous studies, where a midtemplate [48] or study template [37] was generated by taking into account the mean size of the group, in order to use the same level of deformation in both patient and control groups. Further issues are represented by metal and/or blood artifacts, which are commonly found in severely brain-injured patients and can cause a dropout of signal in the MRI images, and motion artifacts. Moreover, the heterogeneity of the methodological approaches and the lack of a gold standard for resting-state fMRI analyses further hamper the interpretation of these data.
Future work should focus on unraveling the relationship (correlation and anticorrelation) between the resting-state networks in different levels of consciousness, going beyond static functional connectivity measures to the assessment of temporal dynamics of connectivity, namely, looking at the changes of correlation and anticorrelation among networks across time. Finally, the biggest challenge will be the applicability of resting-state fMRI on the single-subject level, as required by clinical practice would require.
5.3 Structural Neuroimaging
5.3.1 Conventional Structural MRI
MRI with conventional sequences (T1-TSE, T2-TSE, FLAIR) is the method of choice to detect brain edema, contusion, hematomas, herniation, hemorrhage, hydrocephalus, or hemorrhagic shearing lesion due to diffuse axonal injuries that are commonly observed in posttraumatic patients (T2* sequences) [18].
Some studies have shown the predictive value of the conventional sequences. For example, the number of lesion detected by FLAIR and T2* sequences has been shown to be inversely correlated with the Glasgow Coma Scale of traumatic patients in a coma. The presence of lesions in the corpus callosum and dorsal midbrain has shown to correlate with reduced chance of recovery in coma patients [57, 58]. However, despite encouraging results, these methods have failed to explain the discrepancy between the structural lesions and the clinical condition observed in some patients, highlighting the lack of specificity and sensitivity of conventional MRI in DOC, which so far has not proven to be a reliable diagnostic tool for this patient category [18].
5.4 Diffusion-Weighted Imaging
Diffusion-weighted imaging (DWI) is an MRI technique used to make inferences about white matter structural integrity, otherwise not detectable by conventional structural imaging. The principle behind DWI is molecular diffusion. Molecules in a fluid diffuse randomly, or isotropic, by means of thermal energy (Brownian motion). However, in the brain, there are physical boundaries, especially along white matter tracts, causing higher diffusion along the axons than perpendicular to them. This is called anisotropic diffusion and allows for measurement of the direction of diffusion and, hence, can be used to make inferences about structural integrity. DWI are often employed to compute fractional anisotropy (FA) and mean diffusivity (MD) and to model fibers/axons (DTI) [59, 60] (Fig. 5.3).
