INTRODUCTION
Despite its complex phenotypic heterogeneity, all cases of Parkinson’s disease (PD) have in common two pathologic hallmarks: degeneration of the nigrostriatal dopaminergic system and accumulation of the protein α-synuclein in Lewy bodies. The first of these has provided the focus for two of the most successful therapeutic strategies to date in PD: pharmacotherapy and deep brain stimulation (DBS) (see Chapter 47). Pharmacotherapy (targeting dopamine receptors and replacing or enhancing endogenous dopamine) has revolutionized the treatment of PD and proved that restoring the presence of dopamine could improve many of the motor features (see Chapter 10). However, many patients develop debilitating side effects from this therapy, mainly in the form of levodopa (L-dopa)-induced dyskinesias and on–off phenomena, and the search for a way to deliver dopamine in a more biologic way to the striatum led to the investigation of dopamine cell-based therapies starting in the 1980s.
Cell-based therapy is a logical approach to PD; put simply, the idea is to replace or reduce the number of nigral dopaminergic cells lost to the disease process. Much time and effort has been expended upon attempts to do this with varying success in providing therapeutic benefit for patients. The approaches can be broken down into restorative versus supportive therapies:
• Restorative therapies aim to replace the cells lost as a consequence of neurodegeneration, for example, by transplantation.
• Supportive therapies attempt to maintain the existing or implanted cells, for example, by introduction of trophic factors such as glial-derived neurotrophic factor (GDNF) or related factors (e.g., neurturin).
Supportive strategies are discussed in Chapter 50, so we will concentrate on restorative strategies, and thus, the main focus will be on the field of neural transplantation which has a rich history within PD.
REASONS TO PIONEER NEURAL TRANSPLANTATION IN PD
PD affects a large number of patients and will become more common as the population ages; thus, there is a growing need for better therapies both at the symptomatic and disease-modifying level. The disease itself has a fairly well-described progression allowing for intervention with measurable results and has many intrinsic features making it a model disease for working on with cell transplantation. First, the focal degeneration of neurons in the substantia nigra provides a specific target for replacement. Second, the predominant cell loss in the substantia nigra is dopaminergic neurons, and then only involving a few 100,000 cells, which makes replacing them with grafts a feasible therapeutic approach. Third, the projections of the dopaminergic neurons are quite diffuse and as such provide an easier scope for incorporation of grafted cells than attempting to repair point-to-point rewiring that might be necessary in spinal cord injury, for example. Finally, it is known that giving patients dopamine has a dramatic impact on their clinical features which means that dopaminergic cells should, in theory, give the same benefits.
Cell-based therapy has great potential to provide therapeutic benefit to many, but it remains a field fraught with controversy. Some see the availability of DBS as an alternative effective therapy for PD as a reason to abandon transplant research, whilst others argue that cell-based therapies still have the potential to improve upon the results of DBS with long-term stability, lower maintenance, less complications, and improved health economics (1). Further to this, the proposed cell therapies target only dopaminergic deficits. Whilst motor symptoms can be disabling for patients with the disease, the nonmotor symptoms of PD often have a more debilitating impact on the lives of patients and carers (2). Initially, it was felt that many of these are unlikely to be affected by transplantation of dopaminergic neurons, but there is some evidence that dopaminergic therapy has a role to play in ameliorating symptoms such as depression and sleep dysfunction (3). It does not seem immediately obvious that it might influence other key aspects, for example, dementia. At present, the cognitive deficits leading to dementia appear to be dopamine-independent with loss of posterior cortically based tasks in an age and tau haplotype-dependent process distinct from the frontal based deficits of executive function related to dopamine (4). Nevertheless, the transplants may obviate the need to treat patients with drugs that are known to bring out the early features of PD dementia (e.g., dopamine agonists and hallucinations (5)) and by so doing delay the clinical manifestations of this aspect of the disease. Finally, the field is also burdened by numerous ethical debates, which are summarized in Table 49.1.
SELECTION OF CELLS AND TRIAL DESIGN
Having established that there might be a potential benefit to PD patients in the use of cell-based therapies, the next question that arose was what type of cells should be used and in what environment. Although every cell type has its own unique properties, there are several key factors which they must display if they are to be of use in transplantation for PD:
| Summary of Ethical Dilemmas in Cell-Based Therapy for PD |
The cells | Embryonic cells are controversial because the creation of a cell line entails destruction of the blastocyst from which they are generated. Thus, the ethical controversy revolves around the question of when an embryo becomes a human and consequently whether a life is being taken in creation of the cell line (6). The therapeutic use of fetal material is inherently controversial, though in hfVM transplants it is collected from planned medical and surgical terminations of pregnancy where the tissue would otherwise be discarded. Thus, its use is considered to be acceptable by many as long as a clear separation is maintained between those involved in the termination procedure and those using the tissue for research. The controversial aspect of iPSCs cells is less intuitive, as the use of adult tissue to generate stem cells could be seen to avoid the ethical issues around the start of life. However, some argue that they have the potential to generate a human life and thus should be treated in the same way as human ESCs (7). |
The patients | In agreeing to participate in neural transplantation research, patients make a decision to enroll in a trial with potential for significant harm and no guarantee of personal benefit. It is known that many patients with PD develop a dementia during their disease and even those who do not suffer from this may show early executive dysfunction or impulse control disorders as a result of both the disease process and dopaminergic medication (4,8). Even patients who are not cognitively impaired may show increased impulsivity, and the possibility of therapeutic benefit may distort their view of the risks involved (9). These issues should not prevent research taking place but must be taken into account in the design of trials. |
The clinical trial | The question of double-blind versus open-label studies is a contentious area with strong opinions on both sides. In 2006, Kim et al. (10) canvassed the opinion of American PD Clinical Researchers and concluded that a surgical trial would not be perceived as truly efficacious unless it involved a sham control. Indeed half of respondents believed it unethical not to use sham controls because of the perceived potential for falsely positive outcomes and subsequent patient harm. The counter arguments for sham surgery being ethically unacceptable have been expounded by others (7,11) and include the level of risk to those involved in the sham arm, the enforced deception undertaken by the doctors involved, and the exposure to unnecessary adjuvant therapy such as immunosuppression. Indeed the previous sham-controlled trials (12,13) have been criticized for not using a comparable intervention, their small cohort sizes, and the short length of follow-up made necessary by the need to unblind the trial (7). Alternative strategies can be used to control for investigator bias such as using blinded third-party raters, and the placebo effect can also be minimized by using long-term follow-up of all patients. |
• Survive long term in the host, ideally recreating lost circuitry to some extent, and having functional benefits to the host;
• Remain in an appropriate differentiated state and not form mass lesions or tumors;
• Remain at the site of implantation without migrating to locations where they can interfere with the function of normal neural circuitry.
Whilst these are the basic requirements of the cells, many other factors are also important in any cell-based trial in patients, including
• patient selection (age, stage, and subtype of disease),
• tissue preparation (solid versus suspension grafts; hibernation periods for the harvested tissue),
• tissue placement (putamen +/− caudate),
• graft support (use of immunosuppression or not and for how long; trophic factor support and cografts, etc.), and
• trial design (open label versus double blind, primary end point, trial durations, etc.) (see Table 49.1).
With these properties in mind, there have been many cells investigated as potential therapies for PD, but only one has emerged as being of great value and that relates to the developing human dopaminergic neuroblasts found in the fetal ventral mesencephalon (fVM).
EARLY TRANSPLANT STUDIES IN PD
The early history of cell-based therapy in PD concentrated on autografts, which have obvious practical and ethical advantages over allografts of human fetal ventral mesencephalon (VM) or xenografts of porcine fetal dopamine cells and also circumvented the need for immunosuppression. The cells trialed were all known to produce dopamine or proteins relating to its production and transport. Many reached trials in man, but ultimately none have demonstrated long-term clinical success.
ADRENAL MEDULLA
The first cell-based therapy in PD used the adrenal medulla. The capacity for adrenal medullary tissue to secrete catecholamines including dopamine made it a natural choice, even though the amount of dopamine produced by the chromaffin cells is very low. Nevertheless, studies in animal models of PD showed that such cells could survive and release dopamine to a level to effect modest behavioral recovery in the grafted animals (reviewed by Barker and Dunnett (14)). Despite incomplete effects in animal models (15,16), the technique was taken forward into open-label trials in patients with PD. Initial results were modest at best, with the first patients transplanted in Lund showing transient improvement (17,18). However, subsequently a trial in Mexico showed significant improvements in motor features in two patients and the publication of their results (19) ignited widespread interest in the field. The use of adrenal medullary transplant in its various forms then blossomed, and hundreds of patients were transplanted across the world. Unfortunately, results were not reported, or published only in brief, resulting in a paucity of information available on outcomes to corroborate the initial findings. Thus, attempts were made to collate and comprehensively appraise the data by Freed et al. (20) and Goetz et al. (21) who concluded that there was inadequate available evidence on safety and efficacy for continued use of adrenal medullary transplants outside carefully defined experimental trial settings. This rightly halted the field of adrenal medullary autotransplantation, and the focus shifted toward allogeneic transplants, although some continued to pursue it using peripheral nerve cografts to support the adrenal medullary tissue (22).
SYMPATHETIC GANGLIA
Sympathetic ganglion neurons (SGN) do not secrete dopamine but instead express the proteins aromatic-l-amino acid decarboxylase and vesicular monoamine transporter-2, which are important in the synthesis and storage of dopamine, respectively, in vitro and in vivo (23). SGN grafts were trialed in humans and shown to reduce the time spent in the “off” phase by potentiating the action of L-dopa (reviewed by Nakao et al. (24)). However, relatively few transplants were undertaken with marginal benefits, and as such, this approach never really developed and was soon abandoned.
CAROTID BODY
Carotid body glomus cells are dopaminergic cells derived from the neural crest. These cells secrete not only dopamine but also GDNF, and their main benefits are thought to be mediated through trophic effects on the host dopaminergic network (25,26). In addition, these cells proliferate in response to hypoxic conditions and as such have obvious advantages in transplants, given the milieu the grafted cells find themselves in after implantation into the brain. Having successfully shown benefits in a primate model of PD (27), a clinical trial was undertaken, which at the outset looked encouraging. However, these initially promising motor benefits waned after 6 to 12 months in keeping with poor survival of the grafted cells (28).
RETINAL PIGMENT EPITHELIUM
Retinal pigment epithelium (RPE) cells are accessible, ethically acceptable, and produce L-dopa as well as secrete trophic factors (29). After initial success following transplantation in animal models (30), an open-label clinical trial allografting six patients with advanced PD showed that the procedure appeared safe and improved motor symptoms (31), though postmortem analysis later showed poor survival of the grafted RPE cells at 6 months (32) and a subsequent open-label trial showed peak improvement at 12 months followed by slow decline (33). Nevertheless, a sham-surgery controlled trial in patients with advanced PD was undertaken and showed no clinical benefit of transplanting microcarrier-bound RPE cells at 1 year (34), leading to concerns about continuing this line of investigation (35).
FVM TRANSPLANTS
The ideal cells to graft would specifically replace the A9 subtype of dopaminergic neurons that give rise to the substantia nigra pars compacta (36) and which are lost as part of the core pathology in PD. The obvious candidate would therefore be the developing dopaminergic midbrain neurons derived from fetal material, and this approach was pioneered by the team of Anders Björklund in Sweden.
EARLY EXPERIMENTAL ANIMAL WORK
The first studies using fetal dopaminergic cells were performed in 6-hydroxydopamine (6-OHDA) lesioned rats, a commonly used animal model of PD. These showed that allografts could survive with outgrowth of dopaminergic fibers into the surrounding striatum, resulting in functional recovery on simple motor tasks (37,38). Subsequent experiments moved to the use of human fVM tissue in rat models and refined the techniques involved, establishing that the success of the graft depended on the site of grafting within the striatum and the age of the donor fetus (39). Following this, studies were extended to nonhuman primates (40), paving the way for the start of human trials.
OPEN-LABEL CLINICAL TRIALS
Initially, results from human trials were promising with patients in open-label trials from Lund showing sustained clinical improvement as well as objective evidence of survival and growth of dopaminergic neurons with enhanced striatal uptake of [18F]-dopa on positron emission tomography (PET) (41–43). Further trials followed around the world (summarized in Barker et al. (44)), and although the majority of patients in these open-label studies derived some benefit from the transplant, there was a great variability of effect even within the same center which was a barrier for translating fetal grafting to the clinic as a mainstream therapy, irrespective of the ethical issues inherent in the use of such tissue.
DOUBLE-BLIND CLINICAL TRIALS
Nevertheless, the success of the open-label trials, coupled to a change in US president, prompted the National Institute for Health to fund two randomized control trials in the United States of America involving sham surgery. These trials were designed to see whether the grafts had an effect beyond placebo. One group of patients received grafts, whilst others only had imitation surgery with partial burr holes to represent the surgical procedure of grafting. The first of these trials was undertaken by Freed et al. (12), and once again, they were able to show survival and outgrowth of the grafted cells by increased uptake of [18F]-dopa PET imaging and by examining postmortem tissue. In this trial, this was seen even in the absence of immunosuppression, as this was deemed not to be necessary by the transplant team based on work they had done in privately funded open-label studies. However, the trial failed to meet its primary endpoint at 1 year with grafted patients reporting no significant overall improvements in their PD when asked. Conversely, when patients were grouped according to age (≤60 and >60 years old), the younger transplanted group displayed a significant improvement compared to their nontransplanted counterparts. Indeed, a longer-term follow-up of some of these patients revealed that many had significant improvements both clinically and on PET imaging 4 years postgrafting (45). This study also for the first time reported significant side effects in the form of graft-induced dyskinesias (GIDs) in 15% of patients (12).
In the second NIH-funded study, undertaken by Olanow et al. (13), there was once again evidence of graft survival and reinnervation of the striatum but no significant difference in the primary clinical endpoint. In this trial, patients received either cells from four VMs, one VM or imitation surgery, and there was no significant difference across all groups at the 2-year end point. In this trial, 56% of patients developed GIDs, which were so severe in some cases (as had been the case in the Freed et al. study) that further neurosurgical interventions were needed in the form of DBS (46).
THE FUTURE OF FETAL VENTRAL MESENCEPHALIC TRANSPLANTS
As a result of the two NIH-funded trials, many came to the conclusion that cell-based therapies for PD using fVM tissue did not show clinical efficacy and indeed caused unacceptable levels of morbidity. As such, this therapy had no future, especially given that DBS had by now been shown to be so efficacious (47), and thus, all cell-based transplant trials in PD came to a halt.
However, whilst the lack of effect on the trial primary end points in the double-blind studies could not be denied, it was clear that in these studies (and also in the long-term follow-up of patients in the open-label studies) significant long-term benefits could be had by some patients (45,48). In an attempt to better understand this and a desire to explore the full potential of the emerging field of stem cell–based therapy in PD, a group in Europe (funded as TRANSEURO www.transeuro.org.uk) sought to rigorously interrogate the data from patients who had previously received fVM transplants (44,49,50). Their initial aim was to ascertain the best procedures for a new open-label fVM transplantation trial, on the assumption that fVM transplantation has the potential to work consistently under the right conditions. However, creating these conditions requires careful attention to tissue preparation and grafting as well as patient selection and trial design to maximize the potential for a robust, reproducible, positive outcome. This clinical trial is now about to start, though it must be realized that ultimately fVM transplantation will not be used as a mainline therapy given the logistical and ethical problems involved with its use. Instead, this trial will provide a “proof of principle” for the future development of neural transplant treatments using alternative cell sources, most notably dopamine cells derived from stem cells.
STEM CELL–BASED THERAPY
Transplants of fVM have shown much clinical promise but will ultimately always be limited by tissue availability as well as ethical concerns. Stem cells however are defined by their ability to continuously divide and either self-replicate or differentiate into multiple cell lineages. This has made them key targets for those interested in neural transplantation, although some of the ethical concerns remain with the use of certain types of these cells (see Table 49.1). Stem cells can be harvested from many different types of tissues as well as created anew from differentiated cells (51). These methods are summarized in Figure 49.1 and explained in more detail below. For a summary of the advantages and disadvantages of different stem cell therapies with a comparison to fVM transplants, see Table 49.2.
MESENCHYMAL STEM CELLS
Mesenchymal stem cells (MSCs) are multipotent stromal cells which are the natural precursors to osteocytes, chondrocytes, and adipocytes (52). However, they can also undergo variably successful transdifferentiation in vitro and in vivo to form other cell types, including neurons (53). The concept of using MSCs is attractive for several reasons, including their accessibility, as it is possible to harvest adult stem cells from many tissue types, including the bone marrow. In addition, if injected peripherally into the subject, MSCs can exhibit “homing” activity, targeting lesioned cells in the CNS, a property being explored in therapeutic trials in other neurologic disorders such as stroke and multiple sclerosis where the blood–brain barrier is not intact (54,55). The ability to provide autologous transplants helps avoid issues to do with the host immune response, and indeed the cells may themselves have some immunosuppressive properties, a feature being exploited for experimental treatment of multiple sclerosis (56,57).
< div class='tao-gold-member'>
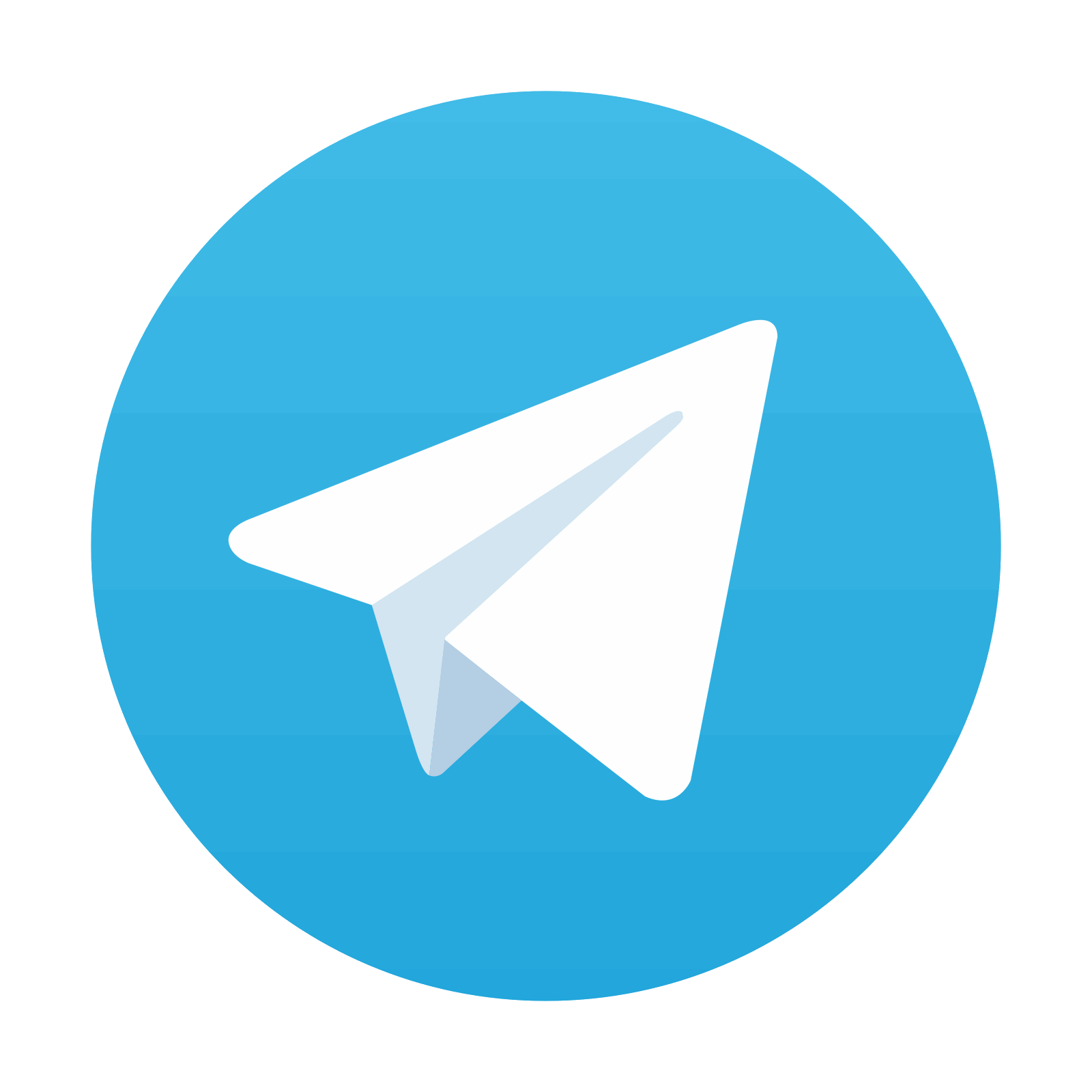