Cellular Effects of Antiepileptic Drugs
Robert L. MacDonald
Michael A. Rogawski
Introduction
Antiepileptic drugs (AEDs) protect against seizures through interactions with a variety of cellular targets, which include various ion channels, a neurotransmitter transporter, a neurotransmitter metabolic enzyme, and a synaptic vesicle protein. AED actions on these targets can be categorized into four broad groups:
Modulation of voltage-dependent ion channels (mainly sodium [Na] but also calcium [Ca] channels)
Effects on γ-aminobutyric acid (GABA) systems, including alterations in the cellular disposition of GABA and enhancement of synaptic inhibition mediated by GABAA receptors
Inhibition of synaptic excitation mediated by ionotropic glutamate receptors
Modulation of neurotransmitter release, particularly of glutamate, through presynaptic mechanisms.
A critical downstream effect of drugs that target voltage-dependent Na and Ca channels may be to selectively modulate glutamate release while only weakly affecting GABA release.132 Thus, group 1 and group 4 actions overlap in that they represent mechanisms for the presynaptic regulation of glutamate release. The ultimate effects of the interactions at these diverse targets are to modify the bursting properties of neurons and to reduce synchronization in localized neuronal ensembles. In addition, AEDs inhibit the spread of abnormal firing to distant sites, which is required for the expression of behavioral seizure activity in localization-related epilepsies. Generalized absence seizures, unlike other seizure types, are believed to result from thalamocortical synchronization. Interference with the intrinsic rhythm-generating mechanisms that underlie the synchronized activity in this circuit is necessary to abort these seizures. This chapter discusses the specific actions or combinations of actions that are believed to account for the anticonvulsant activity of marketed AEDs. Each of the AEDs has its own unique pharmacodynamic properties. In some cases, the uniqueness results because the biophysical parameters governing the drug’s interaction with its target are distinct. For example, phenytoin and carbamazepine both interact with voltage-gated Na channels, but the kinetic properties of the interaction are subtly different. In other cases, the AEDs act selectively on unique targets. For example, ethosuximide targets T-type Ca channels, vigabatrin targets GABA transaminase, tiagabine targets the GAT-1 GABA transporter, gabapentin and pregabalin target α2-δ proteins, and levetiracetam targets the SV2A synaptic vesicle protein. Finally, in other cases still, AEDs such as phenobarbital, felbamate, and topiramate have mixed actions on various targets; their unique properties as anticonvulsants result from the combination of effects on these targets. These various diverse actions confer on each AED a characteristic and unique clinical profile.147
Mechanisms of Action of Established AEDs
Phenytoin and Carbamazepine
Phenytoin and carbamazepine interact with voltage-dependent Na channels at concentrations found free in plasma in patients being treated for epilepsy.96 Both drugs are highly protective against tonic seizures in animal models (as in the maximal electroshock [MES] test), but do not protect against clonic seizures (as in the pentylenetetrazol [PTZ] test). This profile of activity is typical of AEDs that act as Na channel modulators. Na channels are composed of an α subunit (Nav1.1–1.9) associated with auxiliary β1-, β2-, or β3-subunits. An α-subunit is sufficient to form the channel and allow functional expression, but the kinetic properties and voltage-dependence of channel gating are modulated by the β-subunits.
Phenytoin and carbamazepine were demonstrated to reduce the frequency of sustained repetitive firing of action potentials.106,107 The characteristic property of these drugs is that they do not reduce the amplitude or duration of single action potentials but reduce the ability of neurons to fire trains of action potentials at high frequency. The limitation of high-frequency repetitive firing is voltage-dependent, with limitation of firing increasing after depolarization and reducing after hyperpolarization. Once developed, the limitation of firing is prolonged, lasting several hundred milliseconds. The action of the AEDs appear to be due to a shift of Na channels to an inactive state from which recovery is delayed.
On mammalian myelinated nerve fibers, both phenytoin and carbamazepine produced a voltage-dependent block of sodium channels that could be removed by hyperpolarization, a shift of the steady-state sodium channel inactivation curve to more negative voltages, and a reduction in the rate of recovery of sodium channels from inactivation.155 Sodium channels recovered from complete inactivation in a few milliseconds after a 500-ms depolarization to 25 mV. In the presence of 100 μmol/L phenytoin or carbamazepine, however, recovery was prolonged to 90 or 40 ms, respectively. At 50 μmol/L, phe- nytoin and carbamazepine each produced a frequency-depen- dent block. However, the frequency-dependent block produced by carbamazepine was somewhat less pronounced than that produced by phenytoin. Of interest was the finding that phenytoin had a longer time-dependence for frequency-dependent block and for recovery from block than did carbamazepine, which would result in a more pronounced frequency-dependent block for phenytoin than for carbamazepine. Thus, although phenytoin and carbamazepine have qualitatively similar actions on sodium channels, their actions are quantitatively somewhat different. This may explain, at least in part, differences in efficacy for these two drugs in different patients.
In rat hippocampal neurons, phenytoin (200 μmol/L) produced a 20-mV negative shift in the steady-state inactivation
curve for Na channels and produced frequency-dependent block of Na channels.191 Frequency-dependent block was shown at frequencies as low as 1 Hz, and the block increased to 50% at 10 Hz.191 It has been suggested that unbinding of phenytoin from the Na channel is driven by channel deactivation, and that phenytoin may not stabilize the “normal” inactivation state.78 The delay in recovery from apparent inactivation may be due to phenytoin blocking the Na channel by binding to a blocking site on the Na channel that is formed during activation and removed during deactivation and only slowly dissociating from the blocking site after deactivation. The phenytoin block is thus due to the voltage-dependency of deactivation. The slow dissociation, in part, accounts for phenyt- oin’s selective ability to block high-frequency firing: When recovery is slow, the block can accumulate during repetitive activation of the channel. Another important feature of the block of Na channels by phenytoin is its slow onset. Slow binding has two important implications. First, the time course of fast Na currents is not altered in the presence of the drug, and therefore, the kinetic properties of normal action potentials are not perturbed. Second, slow binding means that action potentials evoked by synaptic depolarizations of ordinary duration are not blocked. Even interictal discharges, characterized by depolarizations lasting 50 to 200 ms, are not sufficiently long for drug binding and block of Na channels. Consequently, the frequency of interictal discharges in unaffected by phenytoin. In focal epilepsies, seizure discharges are associated with more sustained depolarizations than those occurring during interictal discharges. Only depolarizations that are as long as those occurring during ictal discharges provide the conditions required for drug binding and block.
curve for Na channels and produced frequency-dependent block of Na channels.191 Frequency-dependent block was shown at frequencies as low as 1 Hz, and the block increased to 50% at 10 Hz.191 It has been suggested that unbinding of phenytoin from the Na channel is driven by channel deactivation, and that phenytoin may not stabilize the “normal” inactivation state.78 The delay in recovery from apparent inactivation may be due to phenytoin blocking the Na channel by binding to a blocking site on the Na channel that is formed during activation and removed during deactivation and only slowly dissociating from the blocking site after deactivation. The phenytoin block is thus due to the voltage-dependency of deactivation. The slow dissociation, in part, accounts for phenyt- oin’s selective ability to block high-frequency firing: When recovery is slow, the block can accumulate during repetitive activation of the channel. Another important feature of the block of Na channels by phenytoin is its slow onset. Slow binding has two important implications. First, the time course of fast Na currents is not altered in the presence of the drug, and therefore, the kinetic properties of normal action potentials are not perturbed. Second, slow binding means that action potentials evoked by synaptic depolarizations of ordinary duration are not blocked. Even interictal discharges, characterized by depolarizations lasting 50 to 200 ms, are not sufficiently long for drug binding and block of Na channels. Consequently, the frequency of interictal discharges in unaffected by phenytoin. In focal epilepsies, seizure discharges are associated with more sustained depolarizations than those occurring during interictal discharges. Only depolarizations that are as long as those occurring during ictal discharges provide the conditions required for drug binding and block.
Benzodiazepines and Barbiturates
Benzodiazepines and barbiturates enhance GABAergic inhibition at the free serum concentrations found in ambulatory patients96 by interacting directly with GABAA receptors. GABAA receptors are formed by the assembly of multiple subunit subtypes (α1–α6, β1–β3, γ1–γ3, δ, ε, π, θ, and ρ1–ρ3) into a pentamer, although the most common and likely subunit composition has been determined to contain two α-subunits, two β-subunits, and a γ-subunit.10 The five subunits are arranged in a counterclockwise sequence (as seen from the synaptic cleft) γβαβα.10 Once assembled, GABAA receptors form chloride (Cl) ion channels, and the current carried by these channels can be modulated by a number of AEDs, including barbiturates and benzodiazepines. GABAA receptors have been shown to be involved in both phasic, inhibitory synaptic transmission and tonic, perisynaptic inhibition36,98 (see Chapter 23). GABA regulates gating (opening and closing) of the ion channel.96,186,197 Binding of GABA increases the probability of channel opening, and the open channel can close and rapidly reopen to create bursts of openings.
Barbiturates enhance GABAA receptor current by binding to an allosteric regulatory site on the receptor, but the specific residues that constitute the allosteric binding site are unknown.126 Mutagenesis studies have demonstrated that a glycine residue in the first transmembrane domain21 and a tryptophan residue in the third transmembrane domain2 of the β-subunit may be involved in barbiturate actions. All GABAA receptor isoforms containing at least an α- and β-subunit have been shown to be sensitive to barbiturates; in general, only minor differences in sensitivity are noted for different isoforms. However, GABAA receptors that contain a δ-subunit instead of the more common γ2-subunit and that are believed to be localized perisynaptically, where they mediate tonic inhibition, are more sensitive to barbiturates than are those containing γ2-subunits that are synaptically localized.1 Single-channel recordings of barbiturate-enhanced single GABAA receptor currents have directly demonstrated that barbiturates increase mean channel open duration but do not alter receptor conductance or opening frequency.96,185
The sensitivity of GABAA receptors to benzodiazepines requires the presence of a γ-subunit coexpressed with an α1-, α2-, α3-, or α5-GABAA receptor subtype.134 Expression of the α4- or α6-subtypes with β1- and γ2-subtypes results in receptors that are insensitive to benzodiazepines.203 Thus, benzodiazepine sensitivity of GABAA receptors depends on both γ-subunit and α-subtypes. The single high-affinity binding site for benzodiazepines is located at the α/γ-subunit interface, whereas the two binding sites for GABA are at the α/β-interfaces. These two binding sites are allosterically coupled.126 Benzodiazepines increase GABAA receptor current, and single-channel recordings have demonstrated that they increase GABAA receptor opening frequency without altering mean open time or conductance.148,189
Ethosuximide and Trimethadione
Ethosuximide and trimethadione, which are effective in the treatment of generalized absence seizures, have been shown to interact with voltage-dependent T-type Ca channels (Table 1). The multiple Ca channel types22,122 are designated as L (Cav1.1–1.4), P/Q (Cav2.1), N (Cav2.2), R (Cav2.3), and T (Cav3.1–3.3), each with different voltage ranges and rates for activation and inactivation. Each channel type is composed of an ion conducting α-subunit (Cav1.1–1.4, Cav 2.1–2.3) and may include smaller accessory subunits, β- (Cav1–4) and α2δ1–4, which are not required for function but do modify gating.81,22
Table 1 Molecular targets of antiepileptic drugs | |||||||||||||||||||||||||||||||||||||||||||||||||||||||||||||||||||||||||||||||||||||||||||||||||||||||||||||||||||||||
---|---|---|---|---|---|---|---|---|---|---|---|---|---|---|---|---|---|---|---|---|---|---|---|---|---|---|---|---|---|---|---|---|---|---|---|---|---|---|---|---|---|---|---|---|---|---|---|---|---|---|---|---|---|---|---|---|---|---|---|---|---|---|---|---|---|---|---|---|---|---|---|---|---|---|---|---|---|---|---|---|---|---|---|---|---|---|---|---|---|---|---|---|---|---|---|---|---|---|---|---|---|---|---|---|---|---|---|---|---|---|---|---|---|---|---|---|---|---|---|
|
Generalized absence epilepsy is characterized clinically by brief periods of loss of consciousness and electrically by generalized 3-Hz spike-and-wave discharges recorded on the electroencephalogram. Thalamic relay neurons play a critical role in the generation of the abnormal thalamocortical rhythmicity that underlies the 3-Hz spike-and-wave discharge. Whole-cell voltage clamp recordings from acutely dissociated relay neurons from the rat thalamus have demonstrated the presence of low-threshold (T-type) and high-threshold Ca currents.174 The T-type currents had properties such that channel activation was necessary and sufficient to cause the generation of low-threshold Ca spikes in thalamic relay neurons. Ethosuximide and dimethadione, the active metabolite of trimethadione, both reduced T-type Ca currents of thalamic neurons isolated from guinea pigs and rats27,28 at clinically relevant concentrations. Phenytoin and carbamazepine, which are ineffective in the control of generalized absence seizures, had minimal effects on T-type current. Dimethadione reduced T-type Ca current by a mechanism similar to that of ethosuximide. Another anticonvulsant succinimide, α-methyl-α-phenylsuccinimide, also reduced T-type currents, whereas a convulsant succinimide, tetramethylsuccinimide, only reduced the T-type current at very high concentrations.29 These results suggest that anticonvulsant succinimides and dimethadione may have their primary action by reducing the T-type Ca current in thalamic relay neurons. More recent studies have largely confirmed these effects of ethosuximide in additional preparations, although variability in responsiveness occurs.55,184,183 It has been proposed that certain factors regulating pharmacologic sensitivity, such as the presence of accessory Ca channel subunits, may contribute to the variability. Recent studies with recombinant human T-type Ca channels indicated that the action of ethosuximide is more complex than previously appreciated.45 There may be two binding sites, one with high-affinity (∼0.1 mM) that accounts for ∼20% of total channel blockade and a low-affinity site (∼10 mM) that accounts for the remainder. Although
ethosuximide did not alter the voltage-dependency of steady-state inactivation or the time course of recovery from inactivation of the T-type Ca current, the drug did inhibit the current in a voltage-dependent manner, with current reduction most prominent at negative membrane potentials and less prominent at more positive membrane potentials.45 Thus, under conditions in which neurons are normally hyperpolarized (resting membrane potentials varying between –55 and –70 mV), the sensitivity of T-type Ca channels to succinimide block will be enhanced. In particular, these agents would be expected to block T-type Ca currents during slow, GABA-dependent network activity, as is believed to occur during absence seizures.55
ethosuximide did not alter the voltage-dependency of steady-state inactivation or the time course of recovery from inactivation of the T-type Ca current, the drug did inhibit the current in a voltage-dependent manner, with current reduction most prominent at negative membrane potentials and less prominent at more positive membrane potentials.45 Thus, under conditions in which neurons are normally hyperpolarized (resting membrane potentials varying between –55 and –70 mV), the sensitivity of T-type Ca channels to succinimide block will be enhanced. In particular, these agents would be expected to block T-type Ca currents during slow, GABA-dependent network activity, as is believed to occur during absence seizures.55
Valproate
Valproate has many pharmacologic actions, none of which by itself can completely account for its clinical activity in epilepsy and the other conditions for which it is used, including bipolar disorder and migraine headache. It has therefore been proposed that valproate acts through a combination of neurophysiological and neurochemical actions.88 Among these diverse actions, effects on GABA systems may play a leading role. Although the precise mechanism is obscure, in some specific brain regions thought to be involved in the control of seizure generation and propagation, valproate increases the turnover of GABA and presumably enhances GABAergic function. Indeed, valproate has actions in animal epilepsy models that overlap with drugs that are believed to act on GABA systems. Thus, valproate is especially active in genetic models of absence seizures, such as the genetic absence epilepsy rat from Strasbourg (GAERS).100 It is also protective against seizu- res induced by methyl 6,7-dimethoxy-4-ethyl-β-carboline-3-carboxylate (DMCM)129 and in the 6-Hz model, an alternative electroshock model that is sensitive to GABAergic agents.8,67 Valproate also exhibits protective activity in virtually all other animal AED screening models including the MES and PTZ models, but its potency in any specific model varies depending on the species and route of administration.88 In view of the activity of valproate in the MES model, which is shared with drugs like phenytoin, carbamazepine, and lamotrigine that are well recognized to modulate voltage-gated Na channels, it has been proposed that valproate might also have an action on Na channels. Indeed, valproate does block sustained high-frequency repetitive firing of neurons in culture.106 However, detailed voltage clamp experiments of valproate actions on Na currents have not been performed; therefore, it cannot be concluded that valproate has a mechanism of action similar to that of the classical Na-channel AEDs.
Although valproate is effective in the treatment of generalized absence seizures, studies in rat thalamic neurons did not demonstrate any effect on T-type Ca current, but subsequently, the drug was shown to reduce T-type currents in primary afferent neurons.70 This effect occurred over a concentration range of 100 to 1,000 μmol/L. However, the magnitude of block was modest, with a 16% reduction seen at 1 mmol/L. Whether this modest reduction in T-type Ca current is sufficient to
explain the effect of valproate on generalized absence seizures is unclear. Furthermore, the basis for the discrepancy between the results obtained in thalamic neurons and primary afferent neurons remains uncertain. Whether this is a relevant mechanism of action for valproate will have to be determined by future investigation.
explain the effect of valproate on generalized absence seizures is unclear. Furthermore, the basis for the discrepancy between the results obtained in thalamic neurons and primary afferent neurons remains uncertain. Whether this is a relevant mechanism of action for valproate will have to be determined by future investigation.
Mechanisms OF Action of Newer AEDs
Felbamate
Felbamate is a dicarbamate, which is an analog of the sedative-hypnotic drug meprobamate (2-methyl-2-propyl-1,3-propanediol dicarbamate). Despite its idiosyncratic toxicities, felbamate is a highly effective AED with a broad spectrum of activity, and is one of only three AEDs for which there is evidence, from a controlled clinical trial, of efficacy in the treatment of the Lennox-Gastaut syndrome.5 Felbamate has been reported to inhibit sustained repetitive action potential firing in cultured neurons, suggesting that it modulates voltage-gated Na channels.201 Although an effect on recombinant Na channels is observed only at relatively high (1 mM) concentrations,175 inhibition of Na-channel function could, at least in part, contribute to felbamate’s anticonvulsant activity. Felbamate has also been reported to inhibit high voltage–gated Ca channels,167,168 but the significance of this action for seizure protection is uncertain. Like meprobamate, felbamate potentiates GABAA receptor currents via an interaction with a site on the GABAA receptor that is distinct from the benzodiazepine recognition site.77,137 The threshold concentration for felbamate modulation of GABAA receptor currents is ∼100 μM, and the IC50 value for allosteric inhibition of t-[3H]butylbicycloorthobenzoate binding to GABAA receptors in rat brain slices is 250 μM.77 Because low therapeutic (anticonvulsant) serum concentrations of felbamate are in the range of 100 to 300 μM, the action of felbamate on GABAA receptors is probably relevant to its anticonvulsant activity. In addition, felbamate at a concentration of 100 μM has been found to block N-methyl-D-aspartate (NMDA) receptor-mediated synaptic responses135 and to inhibit NMDA-receptor currents in cultured neurons (Kd, ∼1 mM137,171). It has been proposed that felbamate may act as a competitive antagonist at the glycine recognition site of NMDA receptors,105,193 and studies in animal models seemed to support this concept.26,200 However, several studies directly examining the interaction between felbamate and the NMDA receptor have shown conclusively that felbamate does not act at the glycine site per se.73,135,137,171,181 On the basis of whole-cell and single-channel recordings, Subramaniam et al.171 concluded that felbamate acts both by a channel blocking mechanism and also by distinct effects on channel gating. Details of the effects on gating have been defined by Kuo et al.,79 who found that low, submillimolar concentrations of felbamate more readily blocked the late sustained phase of NMDA receptor responses but not the initial onset of the response. This phenomenon was ascribed to an acceleration of the decay of responses. It can be speculated that this property would confer selectivity on felbamate to block seizures, because the prolonged pathologic activation that occurs during seizures would be suppressed more strongly than normal (rapid) synaptically generated NMDA responses. In addition, NMDA receptor blockade responses was greater with high concentrations of NMDA, another factor that would allow the drug to selectively block seizure discharges associated with strong activation of NMDA receptors. Kuo et al. further found that felbamate selectively binds with higher affinity to the desensitized state of NMDA receptors (55 μM) than to the resting or activated states (200 and 110 μM, respectively), so that the allosteric blocking action relates to stabilization of the desensitized state.79
Felbamate also selectively blocked currents from recombinant NMDA receptors composed of NR2B subunits at lower concentrations (IC50, 0.5 mM) than it blocked currents from NMDA receptors formed from other subunit combinations.52,73 This selectivity could contribute to the relatively low neurobehavioral toxicity of felbamate in relation to other NMDA receptor antagonists. Unlike the NR2A subunit that is distributed ubiquitously in the central nervous system (CNS), expression of the NR2B subunit in the adult is largely restricted to the forebrain. Thus, felbamate may selectively target NMDA receptors in forebrain areas critical to seizure generation, with less effect on nonforebrain structures that could mediate side effects. The subunit selectivity could also account for the clinical utility of felbamate in seizure disorders affecting the immature brain, such as Lennox-Gastaut syndrome, because NR2B subunits are more abundant in the developing brain.
Gabapentin and Pregabalin
Gabapentin, the lipophilic 3-cycylohexyl analog of GABA, was originally synthesized in an attempt to develop a brain-penetrant GABA agonist. Pregabalin [S(+)-3-(aminomethyl)-5-methylhexanoic acid; S(+)-3-isobutyl GABA] is a congener of gabapentin with similar properties. Although both gabapentin and pregabalin are based on a GABA backbone, bulky aliphatic substituents in the molecules preclude binding to the GABA recognition site on GABAA receptors. The drugs also do not interact with other sites on GABAA receptors, including the benzodiazepine recognition site.172 Although there are reports of agonist activity at GABAB receptors, the preponderance of evidence indicates that GABAB receptors are not a pharmacologic target.178 Additional studies have shown that gabapentin does not inhibit GABA uptake or GABA catabolism, although it may enhance GABA turnover.91 Increases in GABA levels have been reported in humans using noninvasive spectroscopic techniques,80,131 but neither gabapentin nor pregabalin affect brain GABA levels in the rat,40 and thus the significance of these alterations in GABA metabolism are uncertain. Nevertheless, there is little doubt that the therapeutic activities of gabapentin and pregabalin do not reside in effects on GABA systems. Indeed, the molecular targets of gabapentin and pregabalin are almost certainly α2δ-proteins, which are believed to be auxiliary subunits of voltage-activated Ca channels. Strong evidence for this concept is the observation that the binding affinities of gabapentin, pregabalin, and related structures to α2δ-subunits correlate in a stereoselective fashion with their anticonvulsant potencies.12,19 In addition, knockin of a mutation (R217A) in the α2δ-1 subunit in mice, which results in markedly reduced binding of gabapentin and pregabalin, reduces the anticonvulsant activity of pregabalin and eliminates its analgesic activity.148 Because the anticonvulsant actions of phenytoin and the analgesic actions of morphine are not altered by the same mutation, it is likely that the α2δ-1 protein is a target for the pharmacologic effects of pregabalin. However, since the mutation does not completely eliminate the anticonvulsant activity of pregabalin, it is plausible that effects occur on targets other than α2δ-1. Because gabapentin and pregabalin also bind to α2δ-2, this is a logical second target; but until experiments with mice bearing mutations in both α2δ-1 and α2δ-2 are available, the possibility that the drugs confer anticonvulsant activity through interactions with non-α2δ targets cannot be eliminated.
The α2δ-subunits are highly glycosylated proteins having a molecular mass of ∼150 kD (997 to 1150 amino acid residues). Four homologous forms exist, but only subtypes 1 and 2 bind gabapentin and pregabalin with high affinity.75 Each of the α2δ-subunits are products of a single gene that is posttranslationally cleaved into α2- and δ-peptides, which are then covalently linked by a disulfide bridge. Within the multimeric complex that forms the functional Ca channel, the α2δ-subunit is adjacent to or embedded in the pore forming α1-subunit. The δ-subunit portion of α2δ is in close association with the channel and serves as an anchor for the α2-subunit, which is located largely extracellularly.204 Mutagenesis experiments have shown that the gabapentin binding site is probably confined to the α2-subunit protein and the external portion of the associated δ component.18,195 The α2δ-1 and α2δ-2 subunits are believed to form complexes with many voltage-dependent Ca channel types, but it is not yet clear which Ca channels are important for the therapeutic activity of gabapentin and pregabalin, nor is the functional role of the α2δ subunit complex fully understood. However, for some Ca channel types, the α2δ subunit complex has been shown to allosterically enhance current amplitude and also promote channel trafficking to the membrane.
Functional studies of the effects of gabapentin on Ca channel activity have yielded divergent results178; however, the general consensus is that gabapentin and pregabalin reduce the release of neurotransmitters from neural tissue, with effects on glutamate release being of particular relevance in epilepsy.11,38 Thus, in various preparations, gabapentin and pregabalin have been shown to reduce the amplitude of evoked and spontaneous excitatory postsynaptic currents. Surprisingly, however, a reduction also occurs in the frequency of miniature excitatory synaptic currents.3,128 Because these events do not depend on Ca entry through voltage-sensitive Ca channels, gabapentin and pregabalin do not seem to be acting simply by inhibiting Ca channels in presynaptic terminals. Rather, it has been speculated that binding of the drugs to α2δ-subunits directly influences the release machinery,147 possibly by affecting physical interactions between presynaptic Ca channels and proteins mediating exocytosis.165
Gabapentin and pregabalin are absorbed in the gut and pass across the blood–brain barrier via the system L transporter, which is specialized for the transport of large, neutral amino acids.170 The fact that these drugs are substrates for this transporter is essential to their anticonvulsant activity because it allows them to gain access to the CNS.12 However, an interaction with the transporter is not responsible for seizure protection or other pharmacologic activities of the drugs.
Both gabapentin and pregabalin are highly effective in the rat MES test and also are protective against tonic seizures induced by chemoconvulsants, with pregabalin being modestly more potent than gabapentin.20,178,202 Neither agent is as effective in the mouse, which is unusual, because most AEDs show the reverse species selectivity. Both drugs are effective in protecting against seizures in kindled rats, and are highly potent against audiogenic seizures in genetically susceptible mice. The drugs are only weakly active against chemoconvulsant-induced clonic seizures. They are not protective in models of absence epilepsy, and can even promote absence seizures at high doses.178 Overall, the preferential activity against tonic seizures, in the MES test and other models, and lack of protective activity against absence seizures, is consistent with the presumed mechanism of action, which is to inhibit synaptic release. This conclusion is based on a comparison with other AEDs with a similar profile in animal seizure models, most notably Na-channel blocking AEDs. Although Na-channel blocking AEDs do not directly alter synaptic responses, as noted earlier, their inhibitory action on Na channels translates into reduced transmitter output at synapses, with a preference for the release of glutamate.
Lamotrigine
Lamotrigine [3,5-diamino-6-(2,3-dichlorophenyl)-1,2,4-tria- zine] is a phenyltriazine with weak antifolate activity. Lamotrigine was developed after it was observed that the use of phenobarbital, primidone, and phenytoin resulted in reduced folate levels, and that folates could induce seizures in experimental animals.136 It was proposed that antifolate activity may be related to the anticonvulsant properties of these AEDs; however, this has not been confirmed in structure-activity studies.146 Lamotrigine has anticonvulsant activity against tonic seizures in the MES test and also against tonic seizures induced by PTZ, but it is not active in the conventional PTZ test in which clonic seizure activity is the end-point.110 Lamotrigine is also inactive in rat models of absence epilepsy.188 Therefore, its profile in animal models is similar to that of Na-channel modulators such as phenytoin and carbamazepine.
Early studies of the mechanism of action of lamotrigine examined its effects on the release of endogenous amino acids from rat cerebral cortex slices in vitro. As is the case for AEDs that act on voltage-dependent Na channels,87 lamotrigine inhibited the release of glutamate and aspartate evoked by the Na-channel activator veratrine and was less effective in the inhibition of acetylcholine or GABA release.85 At high concentrations, lamotrigine had no effect on spontaneous or potassium (K)-evoked amino acid release. These studies suggested that lamotrigine acts presynaptically on voltage-gated Na channels to decrease glutamate release. In radioligand studies, the binding of [3H]batrachotoxinin A 20-α-benzoate, a neurotoxin that binds to receptor site 2 on voltage-dependent Na channels, was inhibited by lamotrigine in rat brain synaptosomes.25 Several electrophysiological studies have investigated the effects of lamotrigine on voltage-dependent Na channels. Lamotrigine blocked sustained repetitive firing in cultured mouse spinal cord neurons in a concentration-dependent manner at concentrations therapeutic in the treatment of human seizures.25 In cultured rat cortical neurons, lamotrigine reduced burst firing induced by glutamate or K, but not unitary Na action potentials evoked at low frequencies.86 In cultured hippocampal neurons, lamotrigine reduced Na currents in a voltage-dependent manner, and at depolarized potentials showed a small frequency-dependent inhibition.115 Lamotrigine increased steady-state inactivation of rat brain type IIA Na channel α-subunit currents expressed in Chinese hamster ovary cells180 and produced both tonic and frequency-dependent inhibition of voltage-dependent Na channels in clonal N4TG1 mouse neuroblastoma cells, but had no effect on cationic currents induced by stimulation of glutamatergic receptors in embryonic rat hippocampal neurons.194
In cultured rat cortical neurons, lamotrigine at high concentrations was able to inhibit peak high-threshold Ca currents and appeared to shift the threshold for inward currents to more depolarized potentials.86 In clonal rat pituitary GH3 cells, lamotrigine at the same concentration did not inhibit high-threshold Ca currents, caused only slight inhibition of low-threshold Ca currents, reduced rapidly inactivating voltage-dependent K currents, and had no significant effect on Ca-activated K currents.82 In cultured rat cortical neurons, lamotrigine did not appear to mimic the effect of diazepam when tested on GABA-evoked Cl currents.86
These results indicate that the antiepileptic effect of lamotrigine, like that of phenytoin and carbamazepine, is at least in part due to use- and voltage-dependent modulation of fast voltage-dependent Na currents. However, lamotrigine has a
broader clinical spectrum of activity than phenytoin and carbamazepine and is recognized to be protective against generalized absence epilepsy and other generalized epilepsy syndromes, including primary generalized tonic–clonic seizures, juvenile myoclonic epilepsy, and Lennox-Gastaut syndrome.16,41,133 The basis for the broader spectrum of activity of lamotrigine is unknown, but could relate to actions of the drug on voltage-activated Ca channels.145 Lamotrigine blocks T-type Ca channels weakly, if at all.44,49 However, it does inhibit native and recombinant high-voltage–gated Ca channels (N- and P/Q/R-types) at therapeutic concentrations.49,167,196 Whether this activity on Ca channels accounts for lamotrigine’s broader clinical spectrum of activity in comparison with phenytoin and carbamazepine remains to be determined.
broader clinical spectrum of activity than phenytoin and carbamazepine and is recognized to be protective against generalized absence epilepsy and other generalized epilepsy syndromes, including primary generalized tonic–clonic seizures, juvenile myoclonic epilepsy, and Lennox-Gastaut syndrome.16,41,133 The basis for the broader spectrum of activity of lamotrigine is unknown, but could relate to actions of the drug on voltage-activated Ca channels.145 Lamotrigine blocks T-type Ca channels weakly, if at all.44,49 However, it does inhibit native and recombinant high-voltage–gated Ca channels (N- and P/Q/R-types) at therapeutic concentrations.49,167,196 Whether this activity on Ca channels accounts for lamotrigine’s broader clinical spectrum of activity in comparison with phenytoin and carbamazepine remains to be determined.
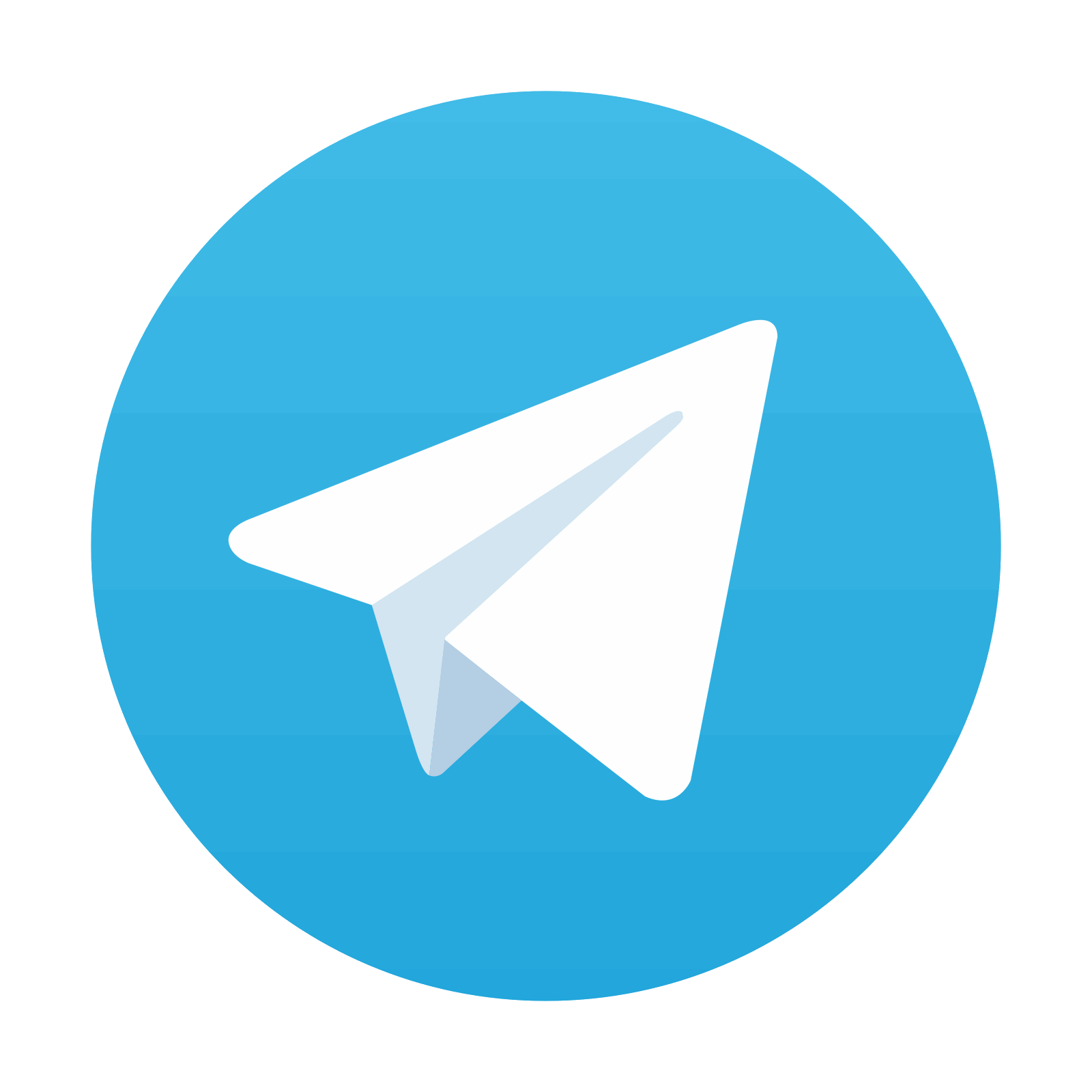
Stay updated, free articles. Join our Telegram channel

Full access? Get Clinical Tree
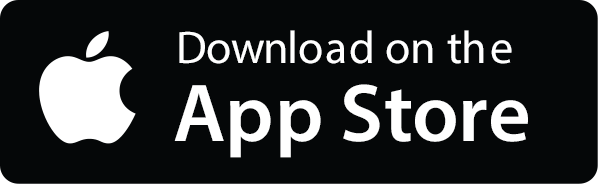
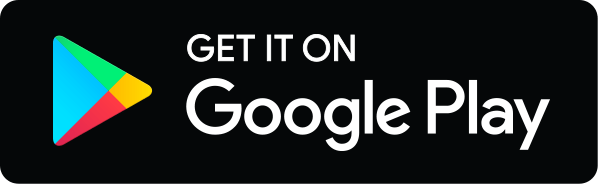