Chapter 3
Central Laryngeal Motor Innervation
Control of laryngeal function has evolved from a simple respiratory reflex (sphincteric and dilation) protecting the pulmonary system in lung fish, into midbrain and subcortical pathways in higher species serving phonatory capabilities associated with warning, mating, and anger as well as with more complex respiratory reflexes. Ultimately, the acquisition of voluntary phonation (speech) in humans was paralleled by direct connections between the laryngeal motoneurons and the cerebral cortex. The central nuclei and pathways providing these roles are reviewed in this chapter (Fig. 3.1).
Fig. 3.1 Circuit diagram of major pathways involved in laryngeal motor control. Structures in boxes with multiple structures are directly connected with each other.
Functional Organization of the Laryngeal Motoneurons in the Nucleus Ambiguus
The functional and topographical organization of the laryngeal motoneurons (LMNs) in the nucleus ambiguus (NA) reflects the evolutionary origin of the larynx from accessory respiratory muscles as well as more recent adaptations to vocalization and speech. Phylogenetically, the NA is first seen in reptiles with the differentiation of the visceral efferent vagus into distinct dorsal and ventrolateral motor nuclei,1 possibly to support the evolution of the orienting reflex, which is characterized by focusing of exteroreceptors, freezing of movement, and neurogenic bradycardia.2 With the evolution of mammals, the additional capacity for voluntary attention, emotions, and communication in the form of facial expressions and vocalizations was added to this orienting behavior as well as adaptations to the greatly increased oxidative metabolism of mammals.2 As such, the mammalian NA projects to supradiaphragmatic structures including the larynx, pharynx, soft palate, esophagus, bronchi, and heart (primary cardioinhibitory motoneurons) with only minor projections to subdiaphragmatic structures restricted to rostral NA. The major innervation to these subdiaphragmatic structures is provided by projections from the dorsal motor nucleus of the vagus.3 The most rostral NA neurons are separated from the main (caudal) division of the NA at birth and are known as the retrofacial nucleus (RFN), which merges with the NA neurons during development.4 Early lesion studies5,6 and horseradish peroxidase (HRP) retrograde labeling investigations in a variety of species4,7–13 have shown that the most rostral LMN group projects to the cricothyroid (CT) muscle and that they extend to or overlap the caudal margin of the facial nerve nucleus. In the caudal two thirds of the NA, the adductor LMNs are located in the dorsolateral division of the NA, running within the recurrent laryngeal nerve. These include, from rostral to caudal, the LMN for the thyroarytenoid (TA), lateral cricoarytenoid (LCA), and interarytenoid (IA) muscles. Although concentrated pools of these LMNs can be distinguished, there is considerable overlap. The LMN pool for the posterior cricoarytenoid (PCA) is located in the middle one third of the NA. The adductor neurons have a diffuse arrangement in the dorsolateral portion of the NA, whereas the PCA abductor neurons are more compactly grouped ventromedially to the adductor neurons.4,7–11,14
Although the topographical representation of the groups of LMNs innervating the individual laryngeal muscles is established, less is known about the functional organization within the motoneuron pools of the laryngeal muscles. In nonlaryngeal muscles the diversity of motoneuron size, synaptic input, and neuromodulator content are integral to the mechanisms underlying motor control, but the extent to which similar relationships extend to the laryngeal muscles is unclear. Increases in muscle force are generally achieved by the progressive recruitment of individual motor units, which consist of a motoneuron and the set of muscle fibers that it innervates (muscle unit).15,16 Because the characteristics of the muscle unit are primarily controlled by the motoneuron, the physiologic and histochemical profiles of all muscle fibers within each muscle unit are uniform.17,18 Furthermore, motor unit characteristics cluster into categories based on their fatigability and their twitch contraction time. In adult human limb muscles, these include type 1 (slow twitch, fatigue resistant), type 2A (fast twitch, fatigue resistant), and type 2X (previously called 2B; fast twitch, fatigable).19 In most nonlaryngeal muscles, motor units are recruited in an orderly size-ordered sequence in which the smallest force and slowest contracting type 1 motor units are recruited first, progressing to the larger force and faster type 2A motor units, and finally to the largest force, 2B motor units.17 This stereotypic recruitment of increasingly larger motor units with greater conduction velocities and larger soma size is known as the “size principle.”16 However, there is evidence that this stereotypic recruitment sequence is modified for the laryngeal motor units as an adaptation to the coordination of their diverse roles in phonation, deglutition, Valsalva maneuver, coughing, and respiration, and possibly also to corresponding functional topographic gradients (compartments or fiber bundles) that have been described in some of the laryngeal muscles. Possibly consistent with these diverse adaptations, some laryngeal motor unit types express unusual myosin heavy chain isoforms such as extraocular myosin or mixtures of myosin heavy chain isoforms.18,20–22
There is evidence for such functional and topographic diversity within the TA muscles and their innervation. Studies in the awake monkey have demonstrated subsets of motoneurons in the NA that are recruited during multiple behaviors for control of generalized laryngeal functions, and other subsets that are recruited during specific behaviors such as vocalization, swallowing, or respiration.23,24 Consistent with these findings, retrograde tracer studies using cholera toxin B have demonstrated distinct muscle fiber bundles in feline TA muscles that are likely to play different roles in laryngeal function.25 Motoneurons innervating muscle fiber bundles in the vocalis were located more medially than the motoneurons of other TA motor units, but there was no apparent rostral to caudal functional diversity among TA motoneurons.25 The motoneuron size did not differ among these TA muscle fiber bundles.25 Although motor unit pools have generally been considered to be equivalent to motor nuclei, reports that some animal limb muscles, such as the cat anterior sartorius, are innervated by two functionally distinct motor unit pools are consistent with this finding.17,26
Cholera toxin tracer studies have also suggested functional diversity within the feline PCA motoneuron pool in that motoneurons projecting to the vertical component of the PCA are located dorsomedially to motoneurons projecting to the oblique subdivision.25 However, there was no significant difference in the soma diameter of these two groups.25 Studies concerning the synaptology of PCA motoneurons provide further support for a diversity of motoneuron pools and recruitment mechanisms. Retrograde transport of cholera toxin subunit B-conjugated HRP was used to label PCA motoneurons in the rat for the determination of their ultrastructure and synaptic organization.27 Two types of neurons were identified in the PCA. One type (PCA-A) was significantly smaller than the other (PCA-B) in frontal sections at the level of the nucleolus. The mean number of axosomatic synapses per section also differed significantly between these motoneuron populations with the largest input to the PCA-B motoneurons (57) followed by the PCA-A motoneurons (36).27 In the PCA-A motoneurons, the majority (65%) of axosomatic terminals were excitatory (Gray’s type II), whereas in the PCA-B motoneurons the majority (61%) were inhibitory (Gray’s type I).27 The ratios of excitatory to inhibitory axodendritic terminals were similar to the data for axosomatic terminals in the respective motoneuron type.27 Based on these findings, it was suggested that PCA-A neurons receive inhibitory inputs containing γ-aminobutyric acid (GABA) from the nucleus tractus solitarius (NTS). This is consistent with GABA-containing neurons in the lateral part of the NTS,28 GABAergic fibers in the caudal part of the NA,28 and physiologic data showing many GABA receptors in the same area.29 It was hypothesized that this inhibitory input to the PCA-A motoneurons is used to relax the PCA, causing the closing of the glottis during swallowing, while the excitatory input to the PCA-B motoneurons would open the glottis during inspiration.27 Possibly consistent with this finding, the human PCA muscle has two divisions: a vertically oriented component, which inserts on the body of the arytenoid cartilage, and a more obliquely directed muscle component, which inserts on the posterior surface of the muscular process of the arytenoid. It has been proposed that the action of the former would be to stabilize the arytenoid in the phonatory action of the cricothyroid (CT), thus lengthening the vocal ligament. In contrast, the oblique portion of the PCA is reported to abduct the vocal ligament during inspiration. In contrast, it has been reported that the dog PCA includes transverse, oblique, and lateral divisions.30 However, muscle stimulation studies indicate that the vertical and oblique divisions are both related to inspiratory abduction, whereas the transverse division regulates the position of the vocal process during phonation.31
It has also been demonstrated that motoneurons projecting to the vertical component of the CT are located ventro-medially to those of the oblique part, suggesting a functional diversity within the CT motoneuron pool.25 However, there is no difference between the soma size of these two groups, although they are both smaller than other laryngeal motoneurons.25 Also, cholera toxin tracer studies concerning the ultrastructural synaptology of CT motoneurons report a single type of neuron in the CT with the majority (70%) of axosomatic terminals being excitatory (Gray’s type II).27 It was suggested that the recruitment of the CT motoneurons during swallowing and respiration is achieved primarily by excitatory input.27 The CT exhibits peripheral subdivisions, suggesting different effects on the vocal cord and voice. The ventral portion of the CT, by closing the cricothyroid gap, would stretch the vocal ligament in apposition to the PCA. The oblique division of the CT could assist in enlarging the glottic aperture during inspiration. Such varied actions of muscle subunits would require separate neural inputs to appropriate motoneurons in the NA.
Laryngeal Respiratory Motor Innervation
Ventral Respiratory Group
The respiratory patterns of laryngeal motoneurons during eupnea are mainly generated by inhibitory and excitatory inputs from neurons in the ventral respiratory group (VRG).32–36 The VRGs in the rat, cat, and rabbit are basically similar, consisting of three divisions. An expiratory division (Botzinger complex) is ventral to the rostral NA, and a second expiratory group extends from the caudal NA though the retroambiguus.35,37 Expiratory neurons in the Botzinger complex project to inspiratory neurons in the rostral VRG.38 The inspiratory division is between the two groups of expiratory neurons.35,37 The respiratory activity patterns originate within the VRG in a neuronal network known as the respiratory central pattern generator (RCPG).39 Augmenting inspiratory neurons in the VRG drive the respiratory spinal motoneurons and interneurons.40 Accessory respiratory muscles are driven by collateral branches of these neurons, which innervate the retrofacial nucleus, the hypoglossal nucleus, and laryngeal motoneurons in the NA.40,41 Neuroanatomic studies based on the retrograde transport of double fluorescent dyes after injection into the cervical spinal cord and the laryngeal muscles have demonstrated that the locations of some bulbospinal interneurons overlap with the NA in both rats and cats.42 Furthermore, in both species they are consistently distributed within the spread of the LMN’s dendritic trees.42 Because there were no double-labeled neurons, none of these laryngeal neurons projected to the spinal cord.42 Although this overlap of bulbospinal interneurons with laryngeal neurons is present throughout the NA in both species, there are rostrocaudal and interspecies variations in the predominant localizations of bulbospinal neurons.42 In both species they were mainly ventrolateral to the LMN in the rostral NA, but at more caudal levels they were predominantly ventromedial and ventrolateral in cats and more concentrated within the NA in rats.42 Physiologic studies have also noted this overlap between the VRG and the NA, and that respiration-related neurons modify their discharge rates during vocalization and swallowing. Most cells have a higher discharge rate during vocalization than during quiet respiration.43
Nucleus Tractus Solitarius
The nucleus tractus solitarius (NTS) plays a major role in the coordination of alimentary and respiratory functions (see also Chapter 2).44,45 However, the neuroanatomic pathways involved in input from this nucleus to the LMN remain unclear in spite of the vital role of these neuronal networks in preventing aspiration. Based on synaptic delay, it has been concluded that the glottic closure reflex in humans following stimulation of the internal branch of the ipsilateral internal branch of the superior laryngeal nerve (SLN) involves at least two synapses, one in the ipsilateral NTS and one in the NA.45 However, the contralateral reflex response has a greater latency, suggesting two or three additional interneurons within the reticular formation.45 It was further proposed that the increased synaptic complexity of the contralateral pathway accounts for the observed greater vulnerability of the crossed adductor reflex to anesthesia due to an increased effect of central facilitation.45 Neuroanatomic tracer studies demonstrating projections from the NTS to the NA and reticular formation are consistent with these findings.46–48
Cortical Respiratory Centers
Although the respiratory patterns of LMN are initiated by the RCPG within the VRG during eupnea, the cerebral cortex plays an essential role in the initiation of volitional respiration such as during breath holding or voluntary inspiratory or expiratory efforts. In humans, premotor cerebral potentials can be recorded in the area of the motor cortex prior to voluntary inspiratory or expiratory efforts, but not during spontaneous quiet breathing.49 Positron emission tomography (PET) has been used to determine the three-dimensional distribution of neuronal activation in the human cerebral cortex during volitional inspiration and expiration.50 During voluntary inspiration, increased blood flow was detected in the right and left primary cortices dorsolateral to the vertex, in the supplementary motor area, the right lateral pre-motor cortex, and the left ventrolateral thalamus.50 The localization of neuronal activity associated with volitional expiration was similar, but more extensive with the inclusion of the ventrolateral thalamus bilaterally and the cerebellum.50 Recently, PET demonstrated increased activity in the ventral rolandic cortex that increased during both voiced and whispered speech.51 It was suggested that the greater activity seen with whispered speech may reflect the greater cortical control of the chest wall and diaphragm during the rapid air loss that occurs during whispering.51 In addition, the observation that this increased activity was left lateralized was suggested to reflect the dominance of the left hemisphere in praxis, because the temporal coordination of the respiratory and laryngeal muscles as well as the rapid and precise recruitment of the laryngeal muscles during pitch adjustments in voiced speech may constitute a praxic demand.51,52
Laryngeal Motor Innervation for Vocalization and Speech
Premotor Neurons
Medullary Reticular Formation
The medullary reticular formation plays a key role in vocal motor coordination. Studies using the anterograde tracer biotin dextran amine have demonstrated numerous efferent projections from the laryngeal motor cortex to the parvicellular, intermediate, and dorsal reticular nuclei,53 but retrograde tracer studies with wheat germ agglutinin HRP indicate that there are no reciprocal projections from these nuclei to the laryngeal cortex.54 Because these reticular nuclei also project to the nucleus ambiguus,55 it has been suggested that they function as a cortico-ambigual relay for motor coordination of learned vocal patterns.53 In addition, these nuclei also receive inputs from the periaqueductal gray for the coordination of innate vocal patterns. Single-unit recordings in the monkey have shown that the parvocellular reticular formation has a greater number of cells with vocalization-related activity changes than other reticular nuclei.56 Because these cells are not active during quiet inspiration, their primary role is not respiratory.56 Conversely, the parvocellular reticular formation contained the greatest number of cells that were active immediately before, but not during, vocalization, indicating that they are more likely involved in the initiation of vocalization or pre-phonatory processes.56 In this respect, they resemble cells with similar activity in the periaqueductal gray (PAG). However, the parvocellular and dorsal reticular nuclei contain neurons with a discharge rate that correlates with changes in the fundamental frequency and intensity,56 whereas the PAG does not contain pattern generating neurons.57
Nucleus Retroambiguus
Anterograde tracer studies with wheat germ agglutinin have demonstrated projections from the nucleus retroambiguus (NRA) to the dendrites of LMNs in the monkey and cat.58,59 These projections are bilateral with a contralateral predominance.59 In both species, the dendrites of cholera toxin-labeled CT motoneurons receive asymmetrical synaptic contacts, containing spherical vesicles, suggesting that they are excitatory.58,59 Physiologic evidence,60,61 neuroanatomic studies,62 and 2-deoxyglucose uptake techniques show that the NRA plays a role in vocalization.63 However, the laryngeal adductor response, following a single stimulus to the internal branch of the superior laryngeal nerve, does not involve neuronal activation of the NRA.64 It has been suggested that this pathway is involved in control of the LMN during vocalization and straining activities.59
Midbrain Centers
Periaqueductal Gray and Laterally Bordering Reticular Formation
Electrical stimulation of the PAG produces vocalizations similar to species-specific calls such as laughing in humans,65 trilling in the squirrel monkey,66 meowing in the cat,67 and ultrasonic vocalizations in the rat.52,68 Ablation of the PAG or its outflow tract in the later tegmental field abolishes all vocalization (mutism).69 However, partial destruction of the PAG generally results in the loss of certain call types with no change in the acoustic structure of the remaining calls, as occurs with lower brainstem lesions.69–71 The observation that these vocalizations can be elicited not only by electrical stimulation but also by glutamate agonists and GABA antagonists demonstrates that they are due to activation of PAG neurons rather than fibers of passage.70,71 Furthermore, single-unit recordings in the PAG have demonstrated vocalization-correlated activity, demonstrating that the PAG is involved in the coordination of brainstem nuclei during vocalization.72 More recent telemetric single-unit studies in the squirrel monkey studies have shown that vocalization-correlated activity with the same reaction profiles extends into the reticular formation bordering the PAG.57
The PAG receives inputs from several limbic structures,69,73 and activities in vocalization-correlated neurons in the PAG correspond to different motivational states.74 Furthermore, all vocalizations elicited from the limbic forebrain require an intact PAG.69,75–77 Recent stereologic studies have demonstrated some regions in the PAG that produce call types differing in the degree of “aversiveness” when pharmacologically stimulated to receive inputs from the same structures, but with quantitative differences in the number of retrogradely labeled cells from certain regions.78 For example, the cell count from the posterior hypothalamus correlated positively with aversiveness, whereas the preoptic area and the nucleus striae terminalis showed a negative correlation.78
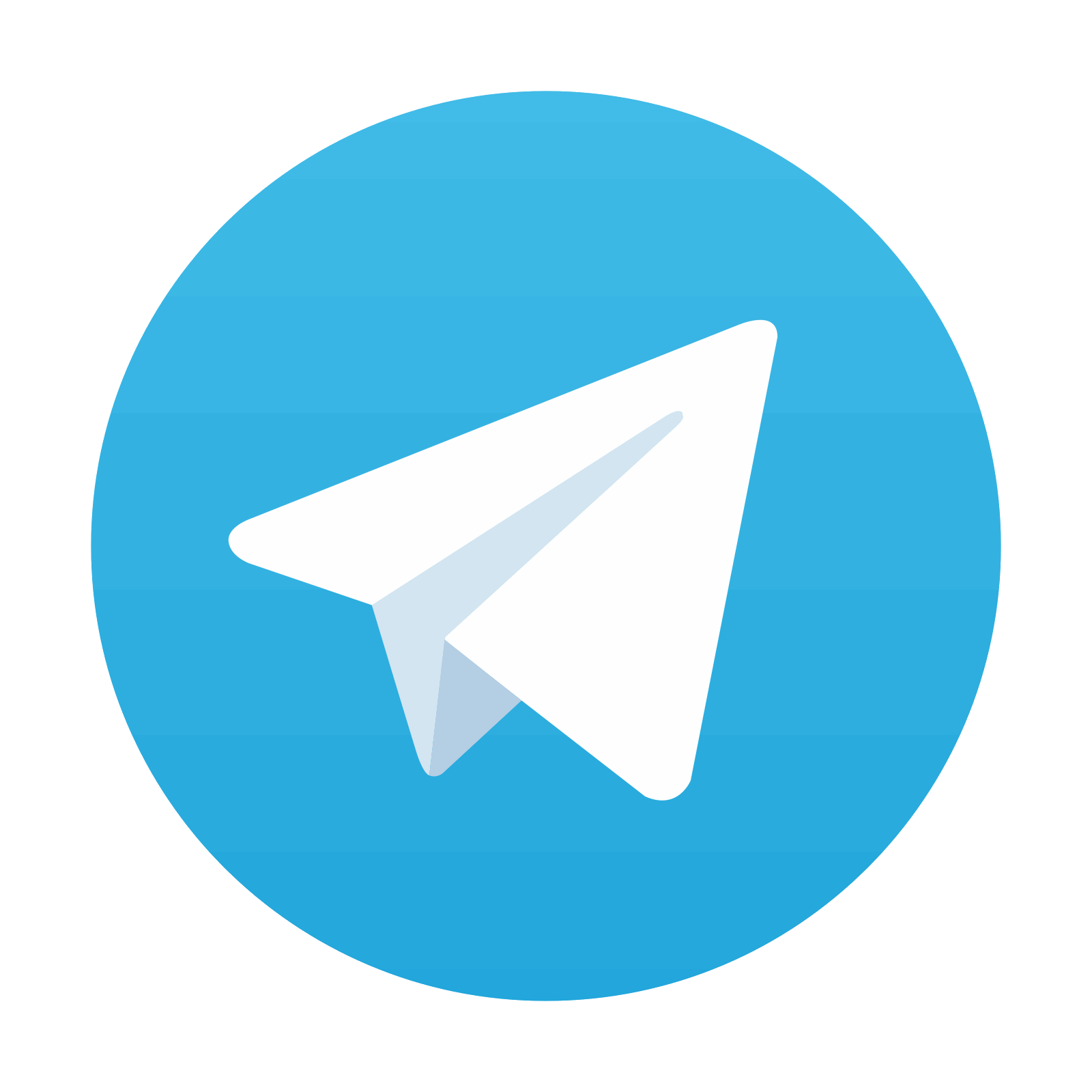
Stay updated, free articles. Join our Telegram channel

Full access? Get Clinical Tree
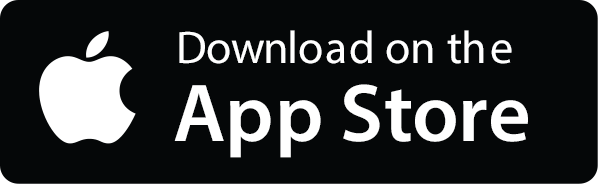
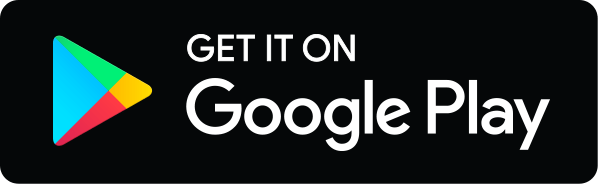