!DOCTYPE html PUBLIC “-//W3C//DTD XHTML 1.1//EN” “http://www.w3.org/TR/xhtml11/DTD/xhtml11.dtd”>
Central Pathophysiology of Dementia and Pain
Michael J. Farrell and Leonie J. Cole
The experience of pain is a function of the brain. Dementia occurs as a result of brain dysfunction, which has the potential to interact with pain processing. It is feasible to make inferences about the likely impact of dementia on pain processing by comparing the functional neuroanatomy of pain with the regional expression of pathology in those diseases that cause cognitive impairment, and other chapters touch on these issues. The primary objective of this chapter is to review the literature of neuroimaging as it relates to pain and dementia. The first part of this chapter will focus on studies involving neuroimaging techniques that have a bearing on interactions between dementia and regional brain responses associated with pain. The second part of the chapter will discuss the possibility that neuroimaging techniques could be used to identify pain in nonverbal people with dementia.
INTERACTIONS BETWEEN DEMENTIA AND REGIONAL BRAIN RESPONSES TO PAINFUL STIMULATION
Other chapters in this book address the dementia syndrome, dementia-related disorders, neuropsychological features of dementia, the functional neuroanatomy of pain processing, and hypothesized interactions between dementia-related disorders and the experience of pain. A general conclusion from these discussions is that the multifactorial nature of dementia and the multiple dimensions of pain are unlikely to intersect in a unitary manner. Indeed, it is possible to hypothesize exacerbation of pain, attenuation of pain, or no change in pain experience among people with dementia depending on contingencies such as dementia-related pathology and environmental factors. It is important to investigate the complexities of dementia and pain because the implications for management could be profoundly different depending on the nature of putative interactions. Neuroimaging techniques are a viable way to gather empirical information about the impact of dementia-related disorders on the brain, the representation of pain in the brain, and how dementia influences central pain processing. This section of the chapter will discuss findings from neuroimaging techniques that relate to these issues. The focus will be on Alzheimer disease (AD) because this disorder, the single most common cause of dementia [22], has been the subject of extensive neuroimaging research [36], whereas the literature pertaining to other dementia-related disorders is less mature.
Neuroimaging of Alzheimer Disease
The last two decades have seen substantial developments in the application of neuroimaging techniques to the investigation of AD. Both positron emission tomography (PET) and magnetic resonance imaging (MRI) have contributed to these advances [1]. The spatial resolutions of the techniques makes it possible to characterize the regional distribution of pathological changes in the brains of people with dementia, which may suggest possible intersections with pain processing regions.
The staging of AD has a major bearing on the outcomes of neuroimaging studies [36]. For instance, contrasts between images of AD-related pathology and healthy brains have fidelity for case identification even before cognitive impairment has been detected. PET images using Pittsburgh compound-B (PiB) to identify beta-amyloid deposits in the brain reveal differences between people at increased risk of AD and controls many years before the onset of cognitive impairment would be expected to occur [50]. Measures of brain hypometabolism made with [18F]-fluorodeoxyglucose–PET and regional decreases in gray matter density measured with MRI are also apparent in preclinical patients of AD, albeit at later times compared to the earliest differences seen with PiB imaging [10]. People with mild cognitive impairment (MCI) show decreased levels of connectivity between brain regions measured with functional MRI (fMRI) suggesting decrements of processing capacity are also apparent before a diagnosis of dementia has been made [26, 68]. Ultimately, dementia and accompanying deficits of cognition lead to changes in regional brain activations during the performance of cognitive tasks, most notably involving memory [64]. However, these changes cannot be characterized simply as decreases. Networks of activation related to cognition in patients with AD often include novel regional responses that have been interpreted as compensation for decrements of processing elsewhere in the brain [13, 64].
Of primary interest for this chapter in the context of dementia neuroimaging are the regional expressions of brain changes associated with dementia-related pathologies in clinical patients. Different imaging methods are more or less likely to be informative when considering the potential for dementia-related pathologies to interact with regions processing pain.
Levels of PiB throughout the brains of people with AD are so pronounced as to have questionable utility as a marker of regional disease expression. The amyloid hypothesis suggests that accumulation of the compound is the earliest step in a series of detrimental effects that eventually lead to cognitive impairment, at which time amyloid levels are very high, but of lesser relevance to subsequent neurodegeneration [37, 38]. Indeed, PiB measures do not show substantial changes in longitudinal or cross sectional comparisons among AD patients with varied levels of cognitive impairment [39]. While the evidence implicating amyloid in AD is undeniable, drawing inferences about regional decrements of brain function on the basis of PiB levels in AD patients may not be applicable when considering pain processing.
Ultimately, it is the loss of functional, interconnected neurons that leads to failing capacity in the brains of people with dementia. Decreased density of neurons is likely to result in atrophy of the gray matter and reduced levels of metabolic activity, both of which can be assessed with neuroimaging. Studies using MRI to compare brain morphology between AD patients and controls have been reported by many independent groups. The volume of this literature has now reached a level where meta-analyses of morphological studies can be undertaken using empirical methods such as anatomical likelihood estimation. A recent meta-analysis of voxel-based comparisons between patients and controls was able to draw upon data collected from 2394 participants from 35 separate studies to characterize the regional distribution of gray matter loss in people with AD [77]. The results of the analysis revealed AD-related atrophy bilaterally in the hippocampii, parahippocampii, amgdalae, medial thalami, caudate, ventromedial prefrontal cortex, precuneus/posterior cingulate, posterior parietal cortices and inferior frontal gyri. This distribution of atrophy is very similar to the regions implicated in AD-related hypometabolism by studies using glucose-PET. The most consistent findings across all studies, irrespective of imaging method are AD-related changes in medial temporal structures, of which the hippocampus is the most prominent.
Outcomes of fMRI studies of memory-related tasks in AD patients and controls have been subject to meta-analysis using activity likelihood estimation [13, 64]. The general conclusion from these analyses is that medial temporal regions show consistent decrements of memory-related function in people with AD, which is wholly compatible with the morphological and metabolic changes seen in this clinical group. Increased levels of task-related activation are also a feature of AD, and are consistently seen bilaterally in the prefrontal cortices [13, 64]. This increase of regional activation is presumably related to compensatory processes. Importantly, the probability and location of such compensatory responses would not be predicted on the basis of AD-related pathology in the brain. The implications of this observation are that pain processing could feasibly incorporate activation in unexpected regions in people with AD if these putative compensatory effects generalize beyond memory tasks.
Additional effects of AD on brain function could have implications for pain processing. Specifically, AD is associated with decrements of inter-regional connectivity. fMRI measures collected from participants lying in a scanner at rest show low frequency fluctuations that reliably occur in distributed networks. There are multiple resting state networks [67], of which the default mode network (DMN) has received the most empirical attention. The DMN is notable for deactivating during tasks and activating during rest [58]. The network includes mesial structures (ventromedial prefrontal cortex, posterior cingulate cortex, precuneus) and the lateral posterior parietal cortices. The functional role of spontaneous signal fluctuations in the DMN is not clear [44], but current views hold that the correlated activity represents introspection and mind wandering [47, 69]. It is notable that memory retrieval tasks activate the same regions [73]. The prefrontal and posterior elements of the DMN are among the regions that show morphological and metabolic decrements in AD, and consequently it is not surprising that regions within the DMN show decreased levels of functional connectivity at rest in people with AD compared to controls [28]. However, AD is not the only disorder that is associated with decreased DMN activity. People with other neurological disorders, such as multiple sclerosis [60], as well as psychiatric disorders [14] and other illnesses [51] have shown impairment of the DMN. Importantly, clinical pain is also associated with decreased activity in the DMN [5].
The overarching conclusion from a synthesis of neuroimaging outcomes in AD is that the regional expression of the disease is most pronounced in the medial temporal lobes, medial thalamus, medial frontal and parietal cortices, and the lateral posterior parietal cortices.
Brain Regions Implicated in Pain Processing
There is a wealth of neuroimaging studies examining pain processing in the human brain, and excellent reviews have been written on varied aspects of this literature [3, 72]. Of critical interest for this chapter is the location of brain regions implicated in pain processing because this information can be integrated with outcomes from dementia neuroimaging to identify points of intersection. An important distinction to be made when considering neuroimaging studies of pain is the difference between experimental and clinical contexts. Studies involving functional brain imaging of responses to noxious experimental stimuli predominate the literature and provide a rich source of information about the location of regional brain responses under the circumstances of relatively short duration pain. Neuroimaging outcomes that implicate brain regions in processes related to the experience of spontaneous, ongoing clinical pain are much less frequently reported in the literature.
Regional Brain Responses to Experimental Pain
Functional brain imaging studies of experimental pain have identified a widely distributed network of brain regions that show responses to noxious stimulation. The components of the pain network that have support from meta-analyses of activation studies are the thalamus, anterior midcingulate cortex (aMCC), insula, primary and secondary somatosensory cortex, right inferior parietal cortex, premotor cortex, prefrontal cortex, lentiform nuclei and cerebellum [18, 21, 23, 31, 45, 62].
Components of the pain network appear more or less frequently in simple contrasts of activity between noxious stimulation and an innocuous or no-stimulus control. For instance, the insula and aMCC are almost invariably reported in pain activation studies, whereas the primary somatosensory cortex activates less reliably. Many factors can modulate responses to noxious stimulation, a discussion of which extends beyond the scope of this chapter. However, modulation of pain responses can be contingent on activation in brain regions that are not principle components of the pain network. These modulation-related regions include the dorsolateral prefrontal cortex, the pregenual cingulate cortex and brainstem regions such as the periaqueductal gray (PAG) and the rostroventral medulla [12, 40]. It is important to consider these regions when assessing the possible impact of dementia-related disorders because impairments of modulation could influence the expression of endogenous analgesia or hyperalgesia.
Recently reported investigations have expanded the list of brain regions implicated in pain processing. Perhaps counter intuitively, regions that deactivate during pain and show activity in non-pain periods have an influence on the experience of pain and levels of activation in the pain network during stimulation. This complex example of network dynamics warrants explanation in the current context because the regions involved are the DMN, which is adversely affected by AD. Independent groups have noted that regions in the DMN deactivate during pain, which is also the case during most tasks or stimuli. However, a recent study reported that the levels of activation in the DMN prior to painful stimulation predicts subsequent levels of pain intensity and regional brain responses [48]. A second study has found that the DMN becomes more active (less deactivated) during noxious stimulation when participants’ minds wander away from the pain, and that functional connectivity between the DMN and the PAG is enhanced when attention is focused elsewhere [41]. The implication of these findings is that coordinated activity in brain regions independent of the pain network can influence the perceptual significance of stimuli and consequently shape the experience of pain. It is possible to speculate that failure of these network dynamics could lead to an impairment of the ability to disengage from noxious events.
Representation of Clinical Pain in the Brain
Functional brain imaging studies of clinical pain appear very rarely in the literature. The paucity of published studies relates to a mismatch between the execution of neuroimaging techniques and the behavior of spontaneous pain. Functional brain imaging is dependent on contrasts between different states that highlight regions where signals increase during a task or stimulus of interest compared to an alternative control period. Spontaneous pain may not conveniently turn on and off nor vary in predictable ways that permit meaningful tests of signal changes measured with functional brain imaging techniques such as fMRI and PET. However, there are some contingencies where clinical pain can be manipulated or varies naturally in ways that can be exploited with functional brain imaging. It is important to consider the outcomes of these studies because the brain regions implicated in clinical pain are not a simple recapitulation of regions identified in studies of experimental pain.
Functional brain imaging studies of clinical pain have included patients with neuropathic and musculoskeletal conditions [4, 6, 24, 29, 34, 42, 56, 76]. Activation has been identified in these groups by modeling spontaneous fluctuations in pain or by contrasting states before and after a manipulation to relieve or exacerbate pain. Differences are apparent between studies, but the outcomes have generally identified a medial/prefrontal network including the ventromedial prefrontal/pregenual cingulate cortices, amygdala, hippocampus, nucleus accumbens and orbitofrontal cortex. These regions are typically ascribed with a role in cognitive/emotional states and reward processing. It is notable that regions including the amygdala and hippocampus are also implicated in animal studies of persistent nociceptive and neuropathic pain [52, 53]. The distinction between outcomes in clinical pain states versus experimental pain models suggests that pain can change over time such that an early emphasis on discriminative aspects is replaced by an experience with emotional overtones, and that these changes stem from processes related to conditioning and learning. A very recent report of cross sectional and longitudinal changes in pain activation involving large cohorts of healthy controls, and subacute and chronic low back pain patients lends support to the evolution of clinical pain processing over time [29]. Furthermore, when compared to controls, brain morphology in chronic pain patients shows regional differences that are compatible with a medial/frontal shift in pain processing [66].
Intersections between Dementia-Related Brain Changes and Pain Processing Regions
Neuroimaging has identified the regional distribution of pathology and related functional changes in AD, as well as characterizing the networks of activation associated with experimental and clinical pain. Comparisons of the respective neuroanatomies of AD and pain networks highlights several points of intersection that allow for tentative hypotheses about the likely impact of dementia on central pain processing during experimental and clinical pain.
Experimental Pain and Dementia-Related Changes in the Brain
The first strain of information that has a bearing on speculative interactions between pain and dementia is the outcome of studies involving PET imaging with PiB. Intuitively, a neuroimaging index of amyloid load would be expected to provide insights into the development and regional expression of AD-related pathology with important implications for understanding how dementia might interact with pain. However, while amyloid levels may be especially useful for understanding the early, preclinical course of AD, PiB signal from clinical patients has less utility. The amyloid cascade hypothesis suggests that the adverse consequences of the substance are expressed early in the disease, and that subsequent accumulation of amyloid is not necessarily a good index of the later stages of pathology that drive decrements of brain function [36]. There are significant implications of this theory for the formulation of putative intersections between AD and pain processing. The widespread distribution of PiB levels in the brains of people with AD, and the lack of correlation between these levels and brain function would suggest that hypotheses about AD-related effects on pain processing based on amyloid plaque distributions could over-estimate intersections between the regional effects of AD and pain processing regions.
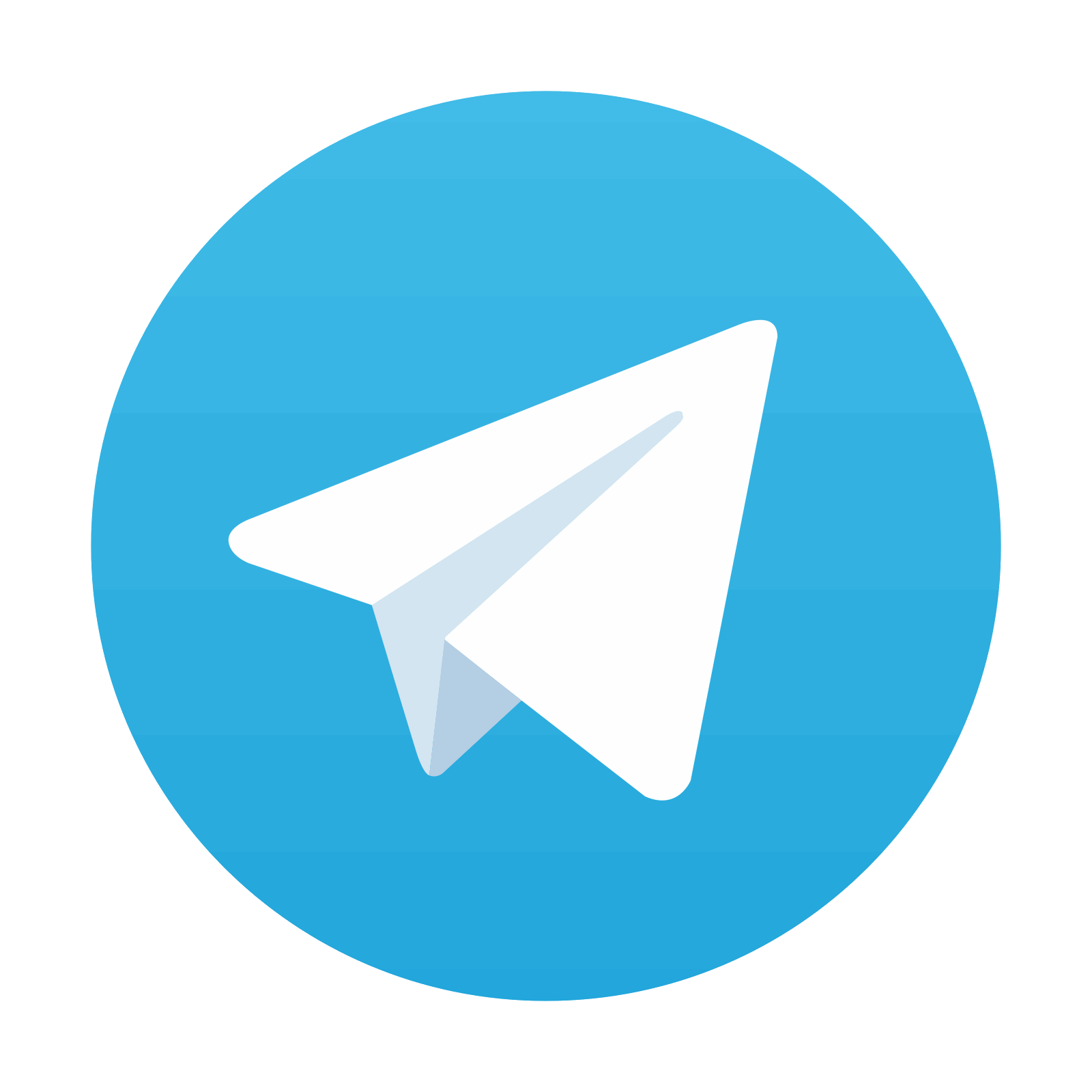
Stay updated, free articles. Join our Telegram channel

Full access? Get Clinical Tree
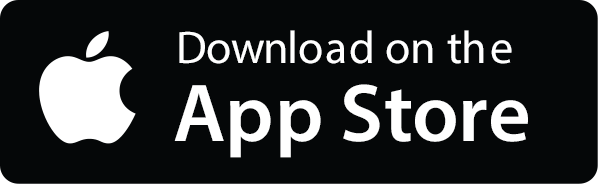
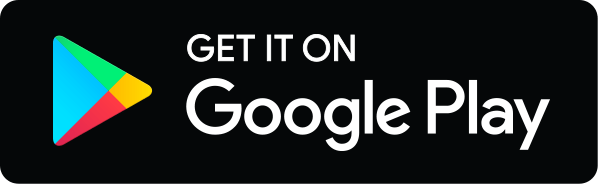