11.1 Introduction
Brain perfusion alterations could conceivably serve as a promising “biomarker” of epileptic seizures. It has been known for some time that focal seizure activity is associated with increased perfusion at the region of the seizure activity (ictal hyperperfusion). It is also known that the focus of seizure activity could have reduced perfusion during the baseline or interictal state (interictal hypoperfusion). Using the appropriate radioligands, ictal hyperperfusion and interictal hypoperfusion could be detected by perfusion SPECT studies. Radioligands such as 99mTc-Hexamethylpropylene Amine Oxime (Tc-HMPAO) and 99mTc-Bicisate (Tc-ECD) have high first-pass extraction in the brain. Therefore, the temporal profile of cerebral uptake and binding of these radioligands corresponds closely to the duration of most focal seizure activity, permitting the pattern of cerebral distribution of the radioligand to map the focus of seizure activity.
A distinction would have to be made between SPECT being a biomarker of epileptic seizure versus a biomarker of epilepsy. The reason is that strictly speaking, ictal SPECT findings are applicable only to the seizure when the radioligand was injected. The SPECT findings could not be extrapolated to the individual’s epilepsy, if the individual has more than one epileptogenic zone or more than one type of epilepsy. Also, the utility and utilization of SPECT findings as a biomarker of epileptic seizure or epilepsy are heavily dependent on a number of parameters, including how soon the radioligand was injected, and how extensive the seizure had spread at the time of injection. Incidentally, ictal and immediate postictal SPECT findings occasionally reveal images of streak patterns or multifocal patterns that suggest pathways of seizure spread or potential epileptic seizure networks.
The objective of this chapter is to critically appraise the current state of knowledge and usefulness of SPECT abnormalities as a biomarker of seizure activity, and potentially as a biomarker of epilepsy. Emphasis will also be given to both the requirements and the constraints that dictate optimization of the techniques for SPECT radioligand injection, ictal and interictal data acquisition, postacquisition data and image processing, and interpretation with correlation with other modalities such as EEG.
11.2 Ictal SPECT Image Acquisition Techniques
11.2.1 Peri-ictal SPECT Dynamics
Ictal SPECT involves injection of Tc99 m ethyl cysteinate diethylester (ECD) or Tc-99 m hexamethyl propylene amine oxime (HMPAO) cerebral blood flow tracer during the early phase of the seizure as blood flow to the seizure onset zone increases. The tracer is delivered to cerebral tissue in proportion to blood flow, crosses the blood-brain barrier in lipophilic form, and is converted to a hydrophylic form intracellularly. In this form the tracer’s distribution is stable for multiple hours with minimal back diffusion and represents a snapshot of the average cerebral blood flow pattern 60–90 seconds after bolus injection of the tracer.1, 2 Tracer injection early in the seizure produces an increased isotope concentration in the seizure onset zone, representing the increased blood flow associated with initiation of the seizure. Late injection of the isotope may capture a reduced cerebral perfusion state and commensurate decreased isotope concentration in the seizure onset zone, though the timing of the change to decreased perfusion is highly variable, and its magnitude is smaller making localization of the seizure onset zone more difficult.3, 4 Following isotope injection and cessation of seizure activity the patient is scanned on a gamma camera system using a high-resolution fan beam collimator. Tomographic images are produced by a filtered back projection or an iterative reconstruction algorithm,5 and algorithms to compensate for photon attenuation in brain tissue are applied6 to produce high-quality images of the isotope’s distribution. Separate acquisition of an interictal or baseline SPECT image provides a patient-specific reference study to aid comparison and analysis of the ictal SPECT. The interictal tracer injection is ideally performed after a minimum of 24 hours of freedom from seizures or auras to exclude any seizure-related effects, although this may not be possible in patients with frequent seizures or in status epilepticus.
11.2.2 Subtraction SPECT Coregistered to MRI (SISCOM)
While visual review of ictal and interictal SPECT studies can be effective, subtraction methods provide a more effective means of quantifying the spatial distribution of differences in isotope concentration.7 Ictal and interictal SPECT volumes are spatially aligned using a voxel intensity based coregistration algorithm.8 Because isotope uptake is highly variable between studies, it is necessary to normalize the pixel intensities of SPECT images prior to analysis. Normalization is performed to equalize the mean intracerebral pixel intensities of the ictal and interictal scans. The spatially aligned image volumes are subtracted, producing a volumetric map of the intracerebral differences in isotope concentration. Spatially, the majority of these differences are low in magnitude and represent normal variation in blood flow patterns, secondary perfusion changes outside the seizure onset zone, fluctuations in the counting statistics of isotope decay, or image reconstruction artifacts, but the perfusion changes in the seizure onset zone are expected to be of higher magnitude than these effects. The standard deviation of difference values in the subtraction volume is calculated, and pixels above or below two standard deviations are reviewed as perfusion abnormalities and potentially delineating the seizure onset zone. Evaluation of the perfusion differences in context with the underlying brain anatomy is facilitated by spatial alignment of the ictal and interictal SPECT and subtraction images with a high resolution MRI.7
11.2.3 SPM-Based Subtraction SPECT
Thresholding the subtraction image results in a map of the largest magnitude perfusion changes between the interictal and ictal images. This map includes normal physiological variations in cerebral blood flow in addition to perfusion changes related to seizure initiation. In order to statistically characterize perfusion changes relative to normal, a map of normal variation in cerebral perfusion can be used to test the significance of ictal-interictal differences.9 Paired resting SPECT images of neurologically normal healthy volunteers acquired using either Tc99 m ECD10 or HMPAO11 are nonlinearly registered, or spatially normalized, into a common standardized template space12 for intersubject comparison. The spatially normalized images constitute a spatially varying statistical distribution characterizing normal variation between resting SPECT scans of an individual. A patient’s ictal, interictal, and subtraction SPECT images can be nonlinearly transformed into the template space, and a statistical test is performed to identify areas where ictal-interictal perfusion changes are statistically significantly different from normal variation. This process is typically accomplished using the open-source statistical parametric mapping (SPM) software,12 running under Matlab (MathWorks, Natick, MA). Spatial smoothing is applied to reduce noise in the images, a cluster size threshold is applied to exclude voxel clusters smaller than the native resolution of the gamma camera system, and Bonferroni or false discovery rate corrections are applied to account for multiple spatial comparisons. Following statistical evaluation the significant perfusion difference map is transformed back into the patient’s image space, and aligned to a high resolution MRI to show the relationship with cerebral neuroanatomy.
11.3 SPECT as a Marker of the Seizure-Onset Zone
11.3.1 Interictal SPECT
The characteristic interictal SPECT perfusion pattern at the seizure-onset focus is hypoperfusion relative to other parts of the brain. Most series that reported the accuracy of interictal SPECT were based on comparison with surface EEG seizure localization, but not with the epileptogenic zone. On this count alone, interictal SPECT can be said to have no proven value to serve as an imaging biomarker of epilepsy or seizures. Furthermore, interictal SPECT has low sensitivity for correctly localizing the seizure focus—correctly localizing in only 28% of extratemporal and 48% of temporal seizure patients.13 A meta-analysis of the literature found that when using surgical outcome as a reference, interictal SPECT has 4.4% false positive rate.14 When the reference is the localization by other established diagnostic tests, the false positive rate is 7.4%. Interictal hypoperfusion changes tend to be more extensive than expected. For example in temporal lobe epilepsy, the hypoperfusion pattern may involve both temporal and extratemporal areas.15 Moreover, in the presence of an MRI epileptogenic lesion such as mesial temporal sclerosis, interictal SPECT is unlikely to give additional localizing information.
Epileptogenic foci have been observed to sometimes be associated with relative reduction of central benzodiazepine receptors. 123I-iomazenil SPECT maps the distribution of central benzodiazepine receptors. SPM analysis of interictal 123I-iomazenil SPECT has more recently been reported in mesial temporal lobe epilepsy to be more accurate than 99 mTc-ECD perfusion SPECT.16 Nonetheless, only 10% of the 123I-iomazenil SPECT localized to the operated medial temporal lobe.
11.3.2 Ictal SPECT by Visual Inspection
The early method of interpreting ictal SPECT studies consist of visually comparing perfusion images derived from ictal SPECT injection with those derived from interictal injection. The result of the comparison is often indeterminate or equivocal. One reason for this is that the amount of radioligand injected and the latency to image acquisition may vary between the two studies, resulting in varying degrees of image intensities. Also, the images obtained may not be at the same anatomical level of the brain; and without coregistration of the SPECT images on MRI images, there is no brain structural detail to aid in the localization of the perfusion changes. Therefore, agreement by visual comparison among reviewers had been low at 41%, compared with SISCOM at 84%. Sensitivity of visual comparison is also low at 39%, compared with 88% with SISCOM. Digital subtraction SPECT such as SISCOM and SPM analyzed SPECT should now be the standard for the use of SPECT in epilepsy.
11.3.3 SISCOM
Computer-aided digital subtraction SPECT has been convincingly demonstrated to be superior to interpretation by visual comparison of the ictal and the interictal images.
Most published studies involving digital subtraction SPECT are those with the SISCOM method. It is unclear whether there is a digital subtraction method that is superior to others, and reports of a number of different subtraction methods have not been validated in a sufficiently large sample of patients.
A much-needed application of ictal SPECT is when the MRI is negative.17 Unfortunately, the number of studies that have addressed this situation is limited. Nonetheless, subtraction ictal SPECT with MRI coregistration is regularly used in some centers for localizing peri-ictal perfusion abnormalities, which can then serve as a target for intracranial electrode implantation to map the seizure-onset zone.18 In a group of 26 MRI-negative refractory temporal or extratemporal epilepsy patients, 92% had a focus of abnormal perfusion detected by reviewers who were blinded to other clinical data.7
When the MRI was negative in refractory temporal lobe epilepsy, SISCOM was still localizing in 82%.19 The resection of the localized abnormality was associated with higher probability of seizure freedom. Seizure-free outcome was also better when subtle MRI structural abnormalities existed at the resection site, or when there were no extratemporal or contralateral spikes or sharp waves.
As for MRI-negative extratemporal epilepsy, the yield of SISCOM was 77% in a small series of 17 patients.20 Of the patients, 55% had excellent postsurgical outcome when there was a SISCOM focus and it was surgically resected. On the other hand, none had excellent outcome when the SISCOM was negative, or when a SISCOM focus was not included in the resection. The difference was statistically significant.
Another study involving a larger sample of patients showed that 68% of 85 MRI-negative extratemporal epilepsy patients had localizing SISCOM abnormalities.21 SISCOM result contributed to the decision to proceed with intracranial EEG recording, but it did not by itself determine which patients proceeded to intracranial electrode implantation. Only 24 patients eventually had resective surgery, and this small number of patients prevented the ability of SISCOM result to distinguish those who had excellent outcome from those who did not.
In a larger group of 130 patients with either temporal or extratemporal epilepsy and with or without MRI lesion, SISCOM was positive in 61%.17 A hypothesis for further epilepsy surgery evaluation was generated in 70% of the SISCOM positive patients. Factors that contributed to the likelihood for SISCOM to generate a hypothesis were shorter duration of seizures, focal seizures, and lesional MRI result. However, only 38% of these patients eventually had resective surgery. The SISCOM abnormality was concordant with the site of surgery in 82% of the patients, and the concordance was associated with 60% rate of seizure freedom at two years’ postsurgical follow-up. In contrast, seizure freedom was observed in only 17% of the 6 patients who had nonlocalizing SISCOM findings. Statistical analysis for the difference was not provided.
11.3.4 SPM-Based Subtraction SPECT
This section discusses the yield of comparing patients’ ictal/interictal subtraction data with normative data by using SPM (SPM subtraction-SPECT). Experience in small series of patients showed that the technique could identify focal or regional perfusion abnormalities in 60% to 93% of patients.11, 22, 23 One particular study disappointingly found that the SPM was not superior to visual comparison of ictal and interictal images.23 In contrast, McNally and colleagues reported that their method of ictal-interictal SPECT analyzed by SPM (ISAS) was 93% sensitive and 87% specific in temporal lobe seizures, and 77% sensitive and 93% specific in neocortical seizures.11 However, these favorable results were achieved with radioligand injection during seizures, because postictal injections are associated with poorly localizing findings, despite being lateralizing in 80% of the injections. SPM-subtraction SPECT has been further applied to analysis of subregions of the mesial temporal lobe in patients with mesial temporal sclerosis. Analysis of the hippocampal subregion correctly lateralized the seizure onset in 91% of patients, and in 87% with amygdala subregion analysis. It should be noted that the foregoing reports of favorable yield of SPM-subtraction SPECT involved predominantly temporal lobe epilepsy and lesional epilepsy. Moreover, some of the studies consist mostly of patients who had responded to epilepsy surgery.11 Therefore, the reported results would not be generalizable to all patients who present for epilepsy surgery evaluation, especially those whose seizure focus is not as obvious, and in whom localizing tests such as SPECT are most needed.
The technique of SPM-based subtraction SPECT analysis that we had developed was statistical ictal SPECT coregistered to MRI (STATISCOM). We conducted an experiment to compare STATISCOM with SISCOM in 87 temporal lobe epilepsy surgery patients.10 Patients were consecutively included in the study. The following statistically significant results were found. Kappa score for agreement between blinded reviewers was good for STATISCOM at .81 and poorer for SISCOM at .36. The sensitivity of STATISCOM in revealing a focus of increased perfusion was 84% vs. 66% with SISCOM. STATISCOM was superior to SISCOM in distinguishing between mesial and neocortical temporal seizures (68% vs. 24% of the patients). The superiority of STATISCOM in this regard was also true for nonlesional patients, with 80% sensitivity vs. 47% for SISCOM. The ability of STATISCOM to distinguish between mesial and lateral neocortical temporal lobe seizures had prognostic significance. Postsurgical seizure-free rate was 81% when the subregional localization was present and 53% when it was absent. In contrast, subregional localization by SISCOM did not have prognostic implication.
One way of assessing the potential for SPECT perfusion abnormality to serve as a focal epileptogenic biomarker independent from the presence of a structural lesion is in MRI-negative epilepsy. A three-way comparison study was performed to evaluate STATISCOM, ISAS, and SISCOM in 21 temporal epilepsy surgery and 28 extratemporal epilepsy surgery patients.24 The range of age at epilepsy onset in the 49 patients was from the first year in life to 34 years. Agreement among the reviewers was equally high for STATISCOM and ISAS, and both were superior to SISCOM in either temporal or extratemporal epilepsy. In temporal epilepsy, kappa score was .55 for STATISCOM, .67 for ISAS, and .32 for SISCOM. In extratemporal epilepsy, kappa score was .56 for STATISCOM, .46 for ISAS, and .40 for SISCOM. The temporal lobe hyperperfusion colocalized with the resected site in 71% of the patients with STATISCOM, 67% with ISAS, and 38% with SISCOM. The extratemporal lobe hyperperfusion colocalized with the resected site in 57% of the patients with STATISCOM, 53% with ISAS, and 36% with SISCOM. In either temporal or extratemporal epilepsy, the rate with either STATISCOM or ISAS was significantly better than SISCOM, but there was no statistically significant difference between STATISCOM and ISAS. In temporal lobe surgery, there was significant association between STATISCOM or ISAS colocalization and excellent postsurgical seizure control, but not for SISCOM colocalization. For extratemporal surgery, there was no association between any of the SPECT method result and postsurgical outcome.
Figure 11.1. This 11-year-old boy was previously healthy and without risk factors for epilepsy. He developed unprovoked spells at age 6.5 years. Spells consisted of a vague aura, staring, flushed complexion, giggling, then restless movement of the legs, but no impairment of awareness. The spells became multiple and daily in occurrence, and uncontrolled by multiple antiepileptic medication trials. Interictal EEGs were unremarkable, but MRI showed subtle signal abnormality at the right mesial frontal white matter and blurring of the nearby cortical margin. Video-scalp EEG monitoring recorded seizures that were mostly bilateral and indeterminate in onset, but with some seizures that were most prominent on the right. SISCOM was nonlocalizing, showing multiple foci (center image), whereas STATISCOM showed hyperperfusion at the right frontal region (right image). Intracranial EEG recording delineated the seizure onset zone at the location of the STATISCOM focus, which was then resected. The patient became seizure-free without antiepileptic medications.
11.3.5 Combined Results from SPECT and Other Functional Imaging Studies
There is the potential for localization accuracy to be improved when SPECT results are combined with those of other functional imaging studies. Perissinotti and colleagues reported in a cohort of children with MRI lesional and nonlesional epilepsy that SISCOM alone agreed with the presumed epileptogenic zone in 67%, and FDG-PET alone in 57%. When the two were combined, the rate improved to 76%.25 However, the presumed epileptogenic zone was determined by video-scalp EEG monitoring and clinical data, not by seizure freedom following focal resective surgery. Only 18 patients underwent surgery, 14 of whom were found to have cortical dysplasia. MRI identified the presumed epileptogenic zone in 72% of these 18 patients, but the rate for the combination of SISCOM and FDG-PET was 100%. The small number of patients did not permit the determination of whether the rates are statistically different between those who were postsurgically seizure-free and those who were not. Such a comparison would be necessary to determine the biomarker value of SPECT by itself or in combination with other functional studies.
The chance of excellent outcome following extratemporal lobe surgery is enhanced when both digital subtraction ictal SPECT and FDF-PET findings colocalize with both temporal lobe MRI lesion and the video-EEG localization. Concordance of both ictal SPECT and PET with MRI and video-EEG was associated with 65% rate of Class I Engel outcome, whereas the rate was significantly lower at only 33% for nonconcordance.26
The value of concordance among diagnostic modalities was also demonstrated in a small cohort of MRI-negative temporal or extratemporal patients.27 All patients who had concordance of SISCOM with MSI and intracranial EEG were seizure-free after resective surgery.
11.4 Role of SPECT in Evaluating Epileptic Networks
As discussed above, SPECT provides information on regional cerebral blood flow (CBF) changes that occur during seizures (ictal) that can be directly compared to interictal (between seizures) brain states. In focal epilepsy the generally accepted theory is that the increased neuronal activity within an isolated seizure focus is associated with increased focal metabolic demand and a compensatory increase in focal CBF. This theory is challenged by animal studies using a quantitative autoradiographic technique, a method similar to SPECT, which demonstrated some ictal hyperperfusion belongs to spreading network, rather than to the focus of seizure onset.28 The time delay between seizure onset and tracer administration is also critically important since the ictal SPECT pattern will reflect both the hyperactivity at the seizure onset and seizure propagation. In addition, there is some evidence that changes in cortical cerebral blood flow may occur as early as 20 minutes before seizure onset.29, 30 Last, the physiological variability of resting cerebral blood flow during the interictal state will also impact SPECT imaging. As discussed in foregoing sections of this chapter, recent advances in SPECT analysis investigating the physiological interictal variability using population ensembles from normal control patients have helped clarify the significant physiological variability in interictal-SPECT.31 The use of statistical parametric mapping and populations of control patients has proven very useful for more accurate analysis of ictal vs. interictal SPECT in focal epilepsy.10, 11, 24
The role of networks in normal and pathological brain activity is an active area of research. While much of the research on epilepsy networks has focused on electrophysiology, the power of SPECT imaging to probe large-scale epileptic networks is well recognized.32 Because of the low temporal resolution of SPECT, related to the combination of time-to-injection (usually 0–60 s) and time-to-uptake delays (30–60 s), ictal SPECT generally reflects the seizure onset zone, activation of coupled networks, and seizure propagation. The propagation has been reported to sometimes appear as a multilobular hourglass appearance,33 but this finding is variable.
Studies from populations of patients with temporal lobe epilepsy show a complex pattern of cerebral blood flow changes in the ipsilateral temporal lobe, contralateral temporal lobe, and other cortical and subcortical structures, and cerebellum. In studies of complex focal seizures in temporal lobe epilepsy, including those with mesial temporal sclerosis, the ipsilateral temporal lobe, middle frontal and precentral gyrus, occipital, and parts of the contralateral postcentral gyrus showed ictal hyperperfusion, but the medial frontal, contralateral posterior cerebellum, and ipsilateral precuneus showed hypoperfusion.34 Additionally, studies have reported ictal hyperperfusion in ipsilateral or contralateral cerebellum,35, 36 ipsilateral thalamus,32 and ipsilateral or contralateral basal ganglia.32, 36
SPECT study of generalized tonic-clonic seizures during electroconvulsive therapy (ECT) demonstrated bilateral cerebellar and parietotemporal hyperperfusion during bilateral and right-sided ECT. Bilateral frontal hyperperfusion was present during bilateral ECT, and right frontotemporal hyperperfusion during right-sided ECT. Relative sparing of many brain regions during generalized seizures suggested that specific networks might be more important than others in ECT-provoked seizures. Interestingly, a group analysis of secondarily generalized tonic-clonic seizures revealed consistent subcortical increases, which were correlated with decreased cerebral blood flow in the frontal and parietal association cortex that resembled the default mode network areas postulated to play an important role in normal attention and consciousness.37
More recently, the brain networks associated with loss of consciousness in temporal lobe epilepsy have been explored. Temporal lobe seizures associated with loss of consciousness show temporal lobe cerebral blood flow hyperperfusion that is followed by increases in bilateral midline subcortical structures (mediodorsal thalamus). The subcortical cerebral blood flow perfusion increase is associated with bilateral frontal and parietal association cortex hypoperfusion. Temporal lobe seizures without loss of consciousness do not demonstrate this complex temporal-subcortical-cortical network pattern of cerebral blood flow changes, suggesting that loss of consciousness is related to activation of a temporal-subcortical network driving more widespread association cortex dysfunction.38 SPECT imaging of cerebral blood flow changes in secondarily generalized seizures showed that prior to secondary generalization there were focal cerebral blood flow increases in the region of focal seizure onset; and during generalization, there was increased perfusion in the superior medial cerebellum, thalamus, and basal ganglia, and hypoperfusion in the “default mode” regions.39
Figure 11.2. SISCOM image showing an hour-glass appearance with right frontal hyperperfusion tapering into an ipsilateral thalamic hyperperfusion focus. Surgical resection of the right frontal focus resulted in seizure control.
Recently, an electrical amygdala kindling model in the macaque monkey was used to investigate SPECT ictal perfusion changes during development of epilepsy, i.e., epileptogenesis. Ictal-SPECT imaging was obtained during epileptogenesis with stimulation-induced seizures that spanned a range of clinical seizure semiologies, from focal seizures causing interruption of ongoing behavior to bilateral convulsions. Ictal SPECT perfusion imaging showed localized and diffuse cortical and subcortical regions of hyperperfusion and hypoperfusion with all seizures, which supports the concept that different seizure types occur within a common network affecting widespread regions of the brain.40 Future studies within such highly controlled animal models may prove very important for advancing the understanding of SPECT imaging as a biomarker of epileptic seizure activity and epilepsy.
11.5 Conclusion
Our foregoing discussion demonstrates that peri-ictal perfusion SPECT is useful in the evaluation for focal resective epilepsy surgery. However, it has major limitations when considered for serving as a biomarker of epilepsy or epileptic seizures. As a biomarker, SPECT should be widely applicable for accurate diagnosis of epilepsy or seizures. Yet, SPECT is expensive, and it requires fairly complex logistical and technical arrangements for implementation. The yield of ictal SPECT is also prominently influenced by the timing of the radioligand injection and the intensity of the seizure. The yield is low when the radioligand is injected during seizures that consist of epileptic auras only.41 The yield is also low when the seizure generalizes or spreads quickly.7 SPECT radioligand has been injected in a more controlled situation during seizures provoked by intravenous administration of pentylentretrazole.42 Using a two-year postsurgical seizure freedom as the outcome measure, concordance of the provoked SPECT focus with the epileptogenic zone had a 90% sensitivity but a specificity of 18%. Moreover, the failure rate of surgery based on localization by chemically provoked seizures has been known to be twice that of localization by spontaneous seizures.43
Much of the evidence for the diagnostic applicability of SPECT is in focal epilepsy and is largely limited to intractable focal epilepsy only. Moreover, ictal SPECT findings are relevant to the seizure episode during which the radioligand was injected. The ability to extrapolate the findings based on one seizure episode to determine the type of epilepsy is challenging in multifocal epilepsy, nonfocal epilepsy, or mixed epilepsy disorders.
Further clinical and animal studies need to be conducted to determine whether peri-ictal SPECT could be a reliable biomarker of epilepsy, or at least of focal epilepsy. This would theoretically entail that the SPECT focus be completely resected whenever deemed medically necessary and safe, and the patient subsequently followed to establish that the resection has resulted in seizure control, preferably without AED intake. One challenge to this prerequisite for peri-ictal SPECT to be a biomarker is the difficulty in standardizing the timing of injection vis-à-vis the stage of progression of the seizure activity. Seizure intensity and spread vary within and between patients. Because of the wide variation in the factors that determine SPECT results, a large study sample would be needed to determine the value of peri-ictal SPECT as a seizure or epilepsy biomarker. However, relatively few epilepsy centers regularly conduct ictal SPECT injection with advanced postacquisition processing of the images.
Further advancements in SPECT radioligands and image processing may improve the marking of the epileptogenic zone by SPECT. For example, SPECT could potentially be a biomarker of cortical dysplasia. SISCOM had been reported to be localizing in 13 of 14 patients with MRI-negative but histologically proven cortical dysplasia.44 Currently available SPM-SPECT analysis could potentially confirm the reliability of SPECT as a biomarker for epilepsy due to cortical dysplasia. There is also the possibility that SPECT may become a component of a “biomarker panel” that consists of results from a number of different tests, which when taken together very highly indicate epilepsy or the epileptogenic zone.
References

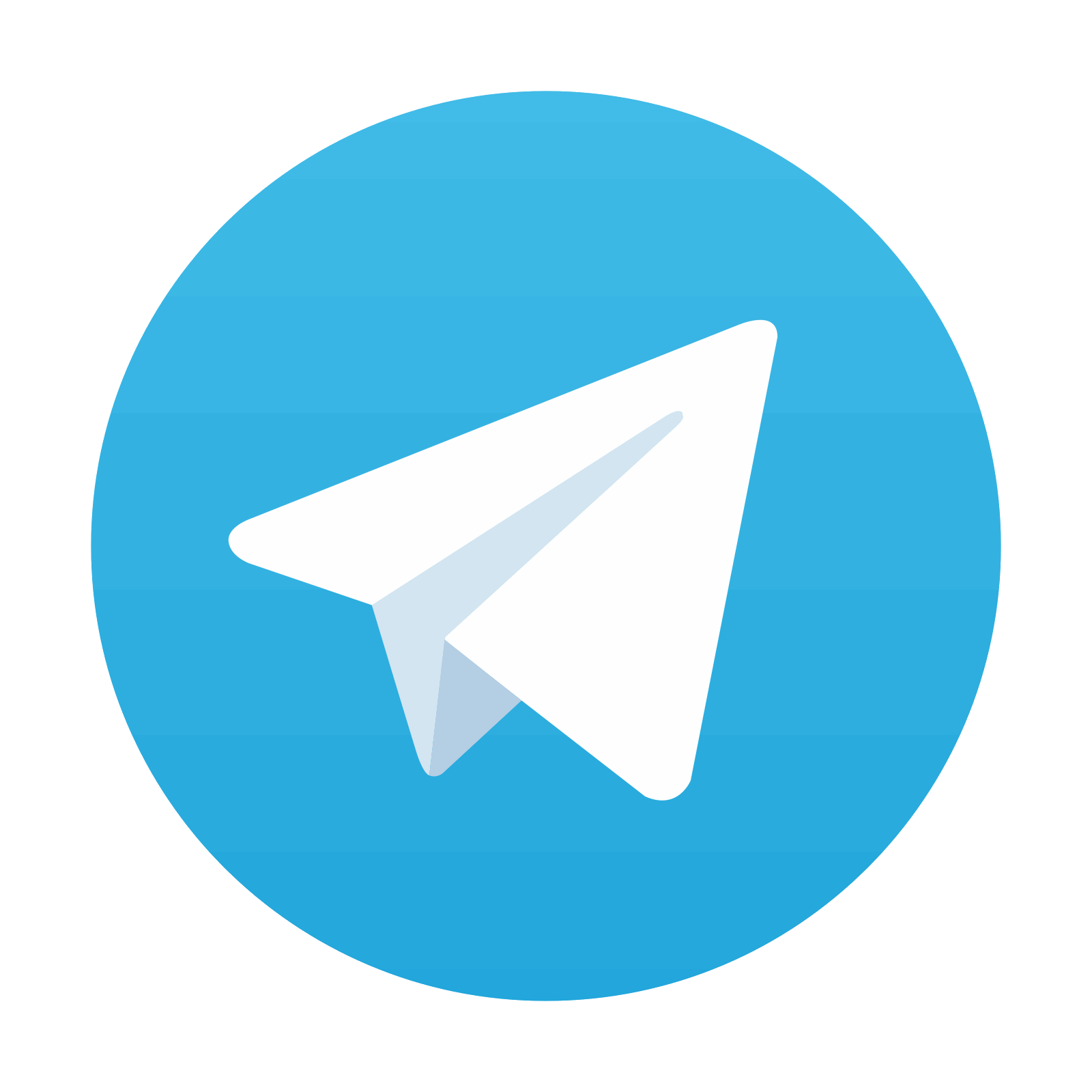
Stay updated, free articles. Join our Telegram channel

Full access? Get Clinical Tree
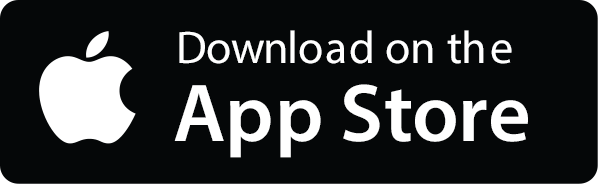
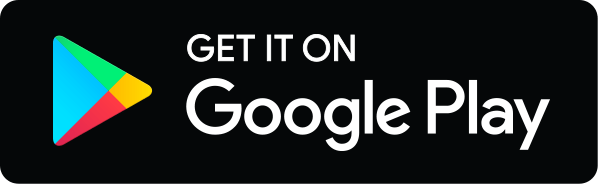
